4 Carotid Arteries in Patients with Transient Ischemic Accidents, Stroke, or Carotid Bruits The results of multicenter trials have shown that carotid endarterectomy benefits a group of patients with significant carotid artery stenoses. These results apply to patients with or without symptoms.1–3 The determination of what represents a significant carotid artery lesion is based on the results of the North American Symptomatic Carotid Endarterectomy Trial (NASCET) study where the benefit of carotid artery surgery was shown when the intervention is done for a stenosis of ≥ 50% diameter narrowing.4 However, carotid surgery is not the only intervention that can be offered to patients with severe carotid artery stenoses. Carotid stenting is emerging as a viable alternative to carotid surgery in selected patients.5 This chapter reviews data on the incidence of ischemic stroke and the linkage to carotid artery disease. Emphasis will be given to certain pathological aspects of atherosclerotic plaque growth and the way that ultrasound can be used to identify carotid lesions that are more likely to cause neurovascular events. Cerebrovascular events, stroke, and transient ischemic attack (TIA) can have different causes. Strokes can be classified as hemorrhagic or ischemic. In young subjects, neurological events are likely due to subarachnoid hemorrhage caused by an aneurysm rupture or due to emboli from a cardiac source or patent foramen ovale. In older individuals, strokes can be due to diseased intracranial arteries or to atheroembolism from outside the cerebral circulation. Another major cause of ischemic events is lacunar strokes. These are believed to be caused by small vessel disease with lesions affecting the smaller branches of the middle cerebral arteries. Because this chapter focuses on carotid ultrasound, the atheroembolic process is emphasized. Embolic phenomena account for most cases of stroke in the United States. Cardioembolic events tend to be associated with atrial fibrillation, with embolization of thrombi forming in the left atrium, embolization of thrombi forming on prosthetic valves, or left ventricular thrombi developing in severely diseased and aneurysmal left ventricles. This linkage is supported by the detection of presumed embolic events with transcranial Doppler sonography. These signals, called high intensity transient events, are believed to represent emboli. Their event rates are much greater in patients with prosthetic valves and atrial fibrillation than in patients without either.6 Atheroembolism is the likely etiology of most strokes in patients with large carotid artery plaque deposits. This linkage is also supported by transcranial Doppler signal detection of high-intensity transitory signals (HITS). The event rate of HITS increases as the severity of carotid plaques increases.7 This does not exclude the possibility that carotid stenoses can progress and cause significant enough narrowing of the artery to cause an ischemic stroke by simply reducing blood flow to the brain. This hypoperfusion syndrome affects the watershed area between the middle and the posterior and anterior circulations of the brain and is a much less common source of acute symptoms than embolic events from atherosclerotic plaque. In the patient presenting with a suspected stroke, the clinical decision algorithm seeks, first, to distinguish ischemic from hemorrhagic strokes. The therapeutic options seem broader in the group of patients with ischemic stroke because the inciting event often has an extracranial origin. Hemorrhagic strokes are often linked to intracranial pathologies, aneurysms, or tumors, or to hypertensive episodes and small vessel rupture. Once a neurological event has been classified as being ischemic, the role of sonography is better defined because it is used to identify lesions that are directly associated with the presenting symptoms. Ultrasound can be successfully applied to the evaluation of these patients if the technique has proven its efficacy at three levels: (1) identifying the type of lesion that causes ischemic stroke and defining its appearance on ultrasound images, (2) establishing the diagnostic accuracy of ultrasound imaging and Doppler evaluation of blood flow in patients with and without neurological symptoms or carotid bruits, and (3) linking the results of specific ultrasound findings with clinical outcomes. Examination of pathological specimens removed during carotid endarterectomy has shown that plaque hemorrhage is associated with the presence of TIAs and with stroke.8 Subsequent studies have further suggested that areas of intraplaque hemorrhage correspond to hypoechoic zones seen with B-mode sonography.9,10 Based on these observations, it would seem possible that sonography might be used to identify specific plaque characteristics that are indicative of an increased risk for subsequent stroke. Studies have shown that the baseline appearance of carotid plaque is linked to the severity of carotid stenosis.11,12 The sonographic appearance of carotid artery plaque changes as the severity of disease progresses: large plaques (causing greater degrees of stenosis) are more likely to be heterogeneous and contain hypoechoic areas.11 The hypoechoic areas seen on ultrasound images can correspond to zones of intraplaque hemorrhage, but can also represent areas where there are higher concentrations of smooth muscle cells, lipid, or thrombus.13 As such, the ultrasound appearance of echolucent areas is nonspecific and cannot be linked to a specific type of tissue. The value of plaque characterization is further placed in doubt by pathological studies which have shown that the presence of intraplaque hemorrhage need not be associated with any symptoms.14,15 However, others have shown that as the degree of sonolucency increases from homogeneous to heterogeneous (class 4 to class 1), the percentage of patients with cerebral infarction proven with computed tomography (CT) also increases significantly from 10.5 to 66%.16 Surface irregularities in the atherosclerotic plaque are believed to serve as the nidus for the formation of platelet aggregates that can then embolize. Carotid plaque ulceration seen during arteriography seems to be associated with a high likelihood for stroke.17 This observation may be relevant to symptomatic individuals with high-grade (≥ 70%) stenoses. By itself, however, the arteriographic appearance of carotid plaque does not appear to directly correspond to the findings seen in pathological samples.9,17,18 Similarly, sonographic detection of carotid ulceration is poor when pathological specimens are used as a gold standard.19,20 The one characteristic of atherosclerotic plaque that consistently correlates with the risk of incident stroke is the severity of the internal carotid artery narrowing. The more the internal carotid artery is narrowed, the higher the likelihood for incident stroke. The positive relationship between incident stroke and internal carotid artery disease has also been shown in patients where carotid artery stenosis severity has been measured by Doppler-derived blood flow velocities.321,22 Although the large endarterectomy trials, Asymptomatic Carotid Atherosclerosis Study (ACAS), European Carotid Surgery Trial (ECST), and NASCET, did not offer any evaluation of plaque characteristics, a study of carotid stenting did include the evaluation of the gray-scale texture of plaque.23 Plaques with more echolucent components were associated with procedure-related events.23 In epidemiological studies, echolucent plaques are also associated with the risk of incident stroke.24,25 A high-frequency transducer (above 5 MHz) is typically used to visualize the carotid arteries because they are superficial. Doppler imaging is used to determine if a lesion causes a severe stenosis. Color Doppler imaging is first used to look for areas of turbulence, seen as mosaic patterns, or a zone of increased blood flow velocities, seen as aliasing of the color Doppler signal. This helps identify the area of greatest stenosis. Doppler spectral analysis is then used to determine the peak systolic velocity and the end diastolic velocity of the internal carotid artery by sampling the region of increased blood flow velocity. Measurements are also made of the peak systolic velocity and end diastolic velocity of the unobstructed common carotid artery, usually 2 cm proximal to the bulb. Ratios can be measured of peak systolic and end diastolic velocities. Evaluation of carotid plaques is then made in both the transverse and the sagittal projections by high-resolution gray-scale, often without color Doppler imaging (Fig. 4–1). The combined use of color Doppler images does, however, permit the visualization of plaques that are mostly echolucent or have echolucent components near their surface. Hemodynamically significant stenoses are, by definition, associated with a pressure drop as blood flows across the stenosis. This will typically take place when a plaque causes at least a 50% narrowing of the lumen diameter. The velocity of blood flow increases as the lumen narrows and is the greatest at the point of maximal stenotic narrowing. This phenomenon can be used to determine stenosis severity with continuous-wave Doppler waveform analysis (nonimaging),26 pulsed-Doppler waveform analysis,27 and color flow imaging.28,29 The zone of increased velocity that is established at the point of maximal stenosis can continue as a stenotic jet, typically extending 1 to 2 cm distal to the stenosis.30 Figure 4–1 (A) Sagittal and (B) transverse images of heterogeneous or type II plaque. Note the presence of a well-defined focal hypoechoic area within the plaque, which is more than 50% of the plaque volume. Color Doppler imaging is used in combination with pulsed Doppler waveform analysis to detect and grade the severity of internal carotid artery stenoses.28 Stenosis severity can also be estimated directly from the color Doppler or power Doppler image by measuring the size of the color channel (Fig. 4–2) that corresponds to the residual lumen of the artery.29,31,32 Direct measurements of residual lumen require that the color and power Doppler sensitivity be set high enough to minimize bleeding of the color Doppler or power Doppler signals outside of the artery walls or into a plaque. Mean velocity estimates can also be made from the color Doppler map by determining the point where the color signals alias,33 or by selecting a velocity “tag.”29,34 These direct measurements are not possible when the calcified portions of a plaque are sufficiently large to mask the artery lumen by acoustic shadowing of any Doppler information from the artery lumen.31 However, because the flow disturbances associated with a stenosis extend, this limitation does not extend to Doppler waveform analysis because the flow disturbances associated with a stenosis extend for at least 1 cm, and possibly 2 cm downstream to the lesion.30,35 The stenotic jet can still be sampled and graded with Doppler waveform analysis (Fig. 4–3). Although this can limit the accuracy of grading stenosis severity, it does permit the identification of a significant stenosis of greater than 50%. There is, therefore, almost complete reliance on Doppler velocity estimates made with pulsed Doppler sonography when detecting the presence of lesions causing at least 50% diameter narrowing. Reported accuracies are close to 90% or higher.28 Power Doppler sonography has been shown to help in distinguishing stenoses in the range of 50 to 70% and additionally may be used as a screening test.32,36,37 Figure 4–2 This color flow image shows a long stenosis of the internal carotid artery. (A) The actual size of the color flow lumen cannot be used to grade the severity of the lesion because some of the color signals likely “bleed” into the diseased carotid wall. (B) The high-grade lesion is confirmed by analysis of the Doppler waveform. Marked elevations of the peak systolic and end-diastolic velocities are consistent with a greater than 80% stenosis. Figure 4–3 This color Doppler image shows aliasing of the color flow signals. (A) Beyond the stenosis, the jet impacts on the outer wall of the internal carotid artery while a zone of flow reversal (blue) forms to the side and below. (B) The high-grade stenosis is confirmed by Doppler waveform analysis showing marked elevations in the peak-systolic and end-diastolic velocities. Blood flow velocity measurements are made by sampling the Doppler waveform at the site of stenosis and by correcting the frequency shift caused by moving blood with the aid of the Doppler equation. This requires that the sonographer estimate the direction of motion of blood. Errors made in determining the angle between the Doppler ultrasound beam and the direction of flowing blood increase at angles above 60 degrees. The angle-corrected velocity measurements, therefore, become progressively less reliable as the angle between blood flow and the ultrasound beam increases above 60 degrees. Ambiguity in determining this angle is also a major source of intersonographer variability. Grading stenosis severity has become an important issue since the results of several randomized controlled trials have demonstrated net benefits of carotid endarterectomy. The first NASCET38 report has shown an absolute reduction in risk of 16.5% over a 2-year period for symptomatic patients with at least a 70% stenosis. A more recent NASCET report4 of symptomatic patients with a 50 to 69% stenosis found an absolute risk reduction of 10.1% at 5 years, but no benefit for those with less than 50% stenosis. The ECST trial report documented an absolute risk reduction of 11.6% at 3 years in symptomatic patients with stenosis of 60% or greater as measured by the NASCET method. The ECST trial also showed that patients who had less than a 40% stenosis (by the NASCET method) who underwent surgery had significantly poorer outcomes at 2 or 3 years than those treated medically.39 Lastly, the ACAS3 study showed an absolute reduction of 5.9% in the 5-year risk of ipsilateral stroke or any perioperative stroke or death for surgical intervention of asymptomatic patients who had a greater than 60% stenosis. These studies have now reemphasized the importance of using accurate noninvasive ways to identify patients with flow-limiting stenoses. The carotid endarterectomy trials also changed the method used to measure the degree of stenosis severity on contrast angiograms. In the past, lumen diameter narrowing was measured by comparing the residual lumen of the internal carotid artery with a “best guess” of the diameter of the internal carotid artery at the same level (Fig. 4–4). This was originally used in the ECST trial.2 The approach adopted by the NASCET and ACAS studies3,38 was to compare the residual diameter of the artery with that of the distal internal carotid artery beyond the stenosis (Fig. 4–5). The arteriograms acquired as part of the ECST trial were subsequently reanalyzed and stenosis graded in the NASCET fashion.40 Results similar to the NASCET results were observed, with benefit for surgery for stenoses above 50%, no benefit for stenoses between 30 and 50% diameter narrowing, and increased morbidity for surgery performed for stenoses of < 30%.40,41 With the acceptance of the NASCET method of grading stenosis severity comes the need of standardizing criteria for Doppler evaluation of stenosis severity. With this in mind, a consensus panel reviewed the diagnostic criteria published in the literature and made recommendations with regard to parameters that offer a consistent way of using Doppler parameters to grade stenosis severity.42 Figure 4–4 This diagram depicts the method of measuring stenosis severity as it has traditionally been performed.
Link of Stroke and Transient Ischemic Attacks to Carotid Artery Disease
Differential Diagnosis
Diagnostic Evaluation
Ultrasound Imaging
Appearance of Carotid Plaque and Association with Ischemic Stroke
Technique
Accuracy of Ultrasound for Evaluating Carotid Artery Disease
Stenosis Grading
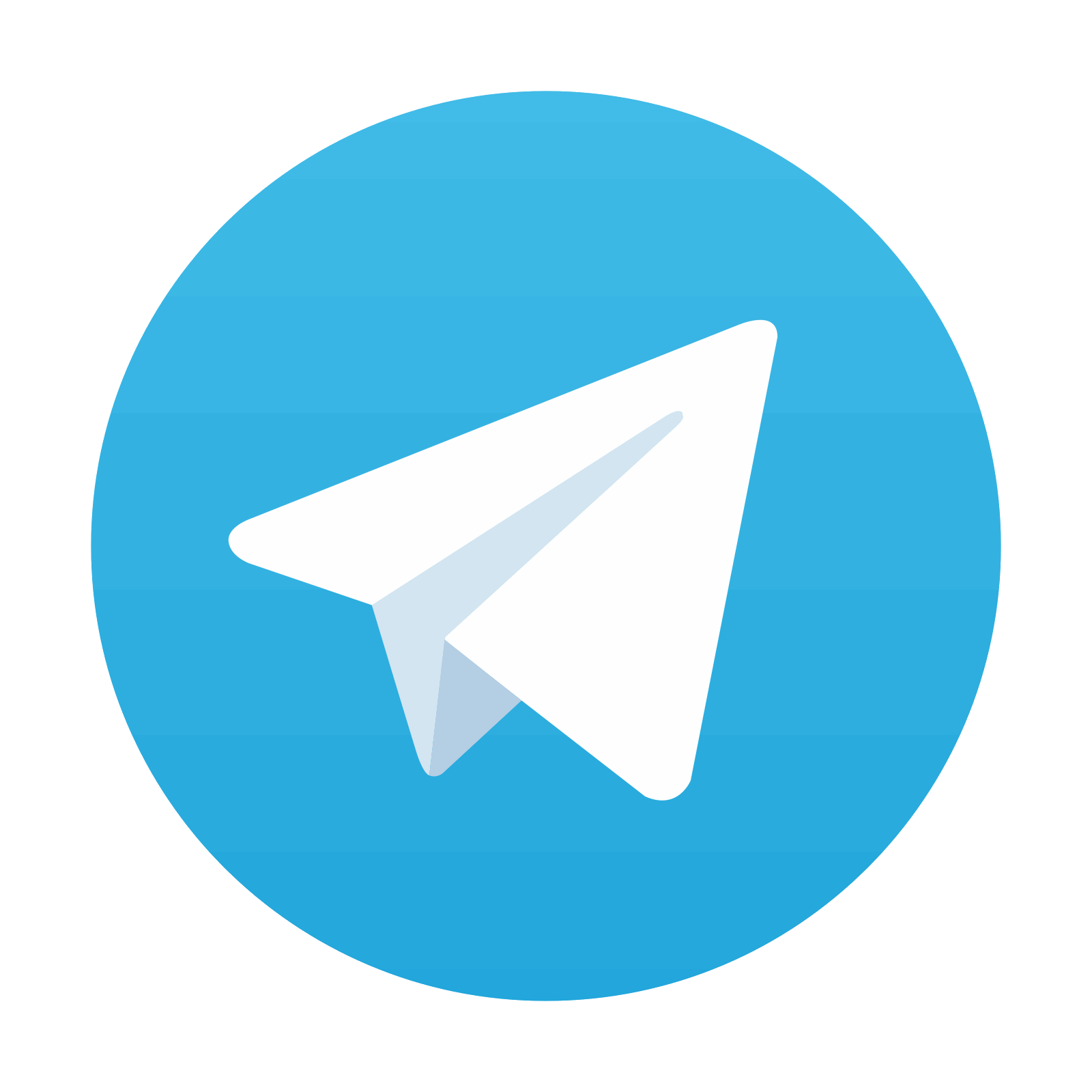
Stay updated, free articles. Join our Telegram channel

Full access? Get Clinical Tree
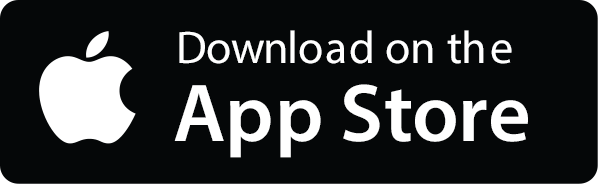
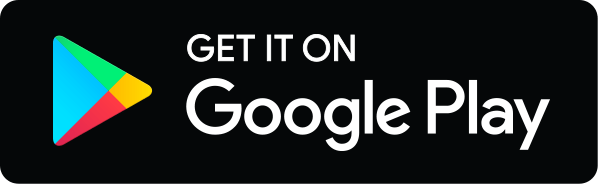