(23.1)
where λ is the shape factor of the cell (1.5 for spherical cells), r is the radius of the cell, E a is the applied electric field, θ is the angle between electric field and the vector from the cell center to any point on its surface, f s is approximately equal to the frequency where the beta dielectric dispersion occurs (below which the cell membrane charge is in step with the electric field), and f is the frequency of the assumed sinusoidal E a [7].
For most cases, the transient terms can be neglected because the electroporation pulse (10 μs to 50 ms) is much larger than the membrane charging time (about 1 μs) [8]. It is hypothesized that if the potential drop across the membrane exceeds approximately 1 V, structural rearrangement of the lipid bilayer occurs, creating permanent aqueous pathways or pores for ions and macromolecules to pass through, i.e., irreversible electroporation [9]. The theory of pore formation describes the presence of both hydrophobic and hydrophilic pores in the plasma membrane (Fig. 23.1). Hydrophobic pores are naturally occurring creating nonconductive gaps in the lipid bilayer due to thermal fluctuations. They are the precursor for hydrophilic pores, which conduct electricity. The transition from hydrophobic to hydrophilic pore depends on the energy function for each type of pore [11]:
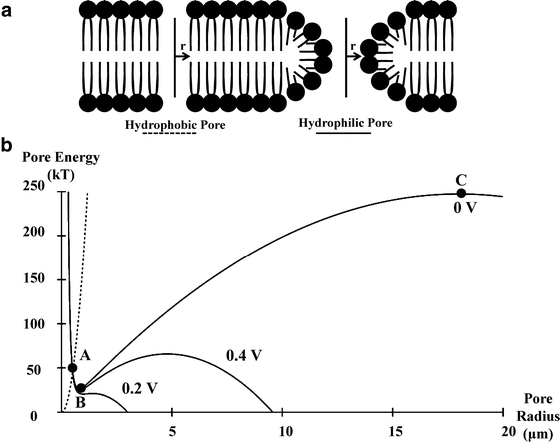
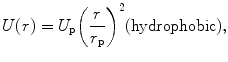
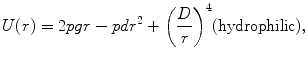
where r is the radius, γ is the edge energy of a pore, δ is the membrane surface tension, D is a constant, and r p and U p are the minimum radius and energy required for the existence of a hydrophilic pore, respectively [12]. The values for the different parameters can be found in [10], and each term is given in units of kT, where 1 kT = 4.11 × 10−21 J. Both curves are shown in Fig. 23.1. In an attempt to minimize energy, a pore with a given radius assumes the lesser of the two configurations. The peak at point A (r p, U p) represents the energy barrier for the creation of hydrophilic pores, while the maximum at point C (r e, U e) is the energy barrier for the spontaneous expansion of hydrophilic pores. When a hydrophobic pore is created with a radius greater than r p, it naturally transitions into a hydrophilic pore. Thermal fluctuations cause the hydrophilic pore radius to settle at a minimum energy radius of r m (point B), and spontaneous expansion of pores is unlikely when the induced V m equals zero. An increase in V m due to the application of an external electric field reduces the pore energy by the capacitive contribution of the membrane to pore energy,
[10], where a p is a constant based on the dielectric properties of the membrane and surrounding medium. In Fig. 23.1, as V m is increased, the likelihood of spontaneous pore expansion increases due to the reduction and eventual elimination of the maximum at point C (r e, U e).
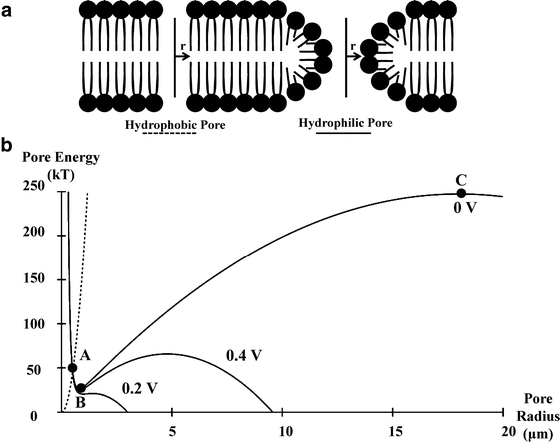
Fig. 23.1
Theoretical illustration of pore formation in a planar bilayer membrane adapted from [10]. (a) Schematic diagram of hydrophobic and hydrophilic pores with radius, r. (b) Energy of hydrophobic (dashed line) and hydrophilic (solid line) pores as a function of radius and the influence of increasing transmembrane potential on each. The cutout represents a zoomed view of pore energy around the energy barrier for the creation of hydrophilic pores at point A (r p, U p). The point C (r e, U e) is the energy required for the spontaneous expansion of hydrophilic pores and B (r m, U m) is the minimum stable energy of a hydrophilic pore
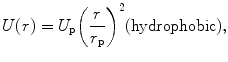
(23.2)
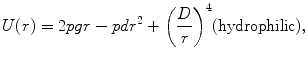
(23.3)

Scanning Electron Microscopy Data of IRE-Treated Tissue
A team of researchers from UCLA’s Radiology Department were the first investigators to successfully take scanning electron microscope (SEM) micrographs of tissue that had previously been irreversibly electroporated [13]. They employed a NanoKnife® LEDC System to induce IRE in the hepatic tissue of New Zealand rabbits and Yorkshire swine using a bipolar IRE electrode operated at 2,500 V. Ultrasound guidance was used to place the electrode which was used to deliver 90 pulses each with duration of 100 μs at approximately 1 Hz. The rabbits were euthanized at 15 min and 1 h post-NanoKnife ablation, whereas the swine were euthanized after 24 h.
For many years, it had been theorized that the mechanism of action of irreversible electroporation was the formation of nanoscale pores in the cell membrane that led to the cell’s loss of homeostasis and subsequent death. Until this study was conducted, there was little to no direct evidence that nanoscale pores were actually being formed by the induced high-intensity electric fields and accompanying current flow.
This study’s key findings were the following:
1.
Nanopores were still visualized 24 h after an IRE ablation.
2.
Nanopores were visualized using as low as 5,000× magnification, but fine details of the pore could only be observed at 50,000× magnification or higher.
3.
Untreated normal liver hepatocytes cell membranes on control samples did not exhibit any pores or nanopores.
4.
The location of the IRE-induced nanopores was all found within the IRE-ablated zones with complete cell death confirmed by histological analysis of the H&E stained slides.
5.
The nanopore features in the IRE-ablated rabbit liver samples were identical to the nanopores of IRE-ablated swine liver (Fig. 23.2).
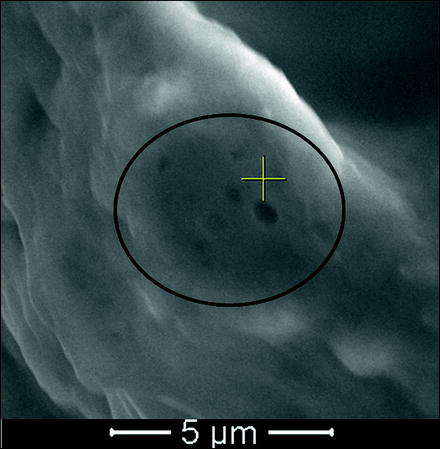
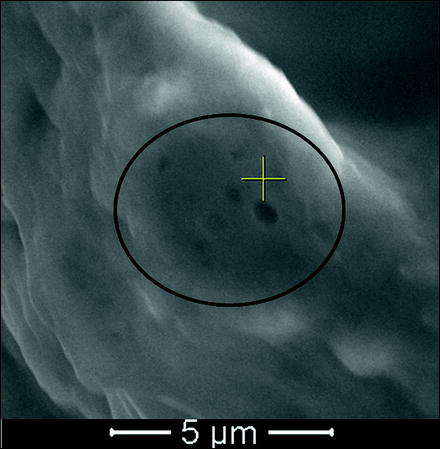
Fig. 23.2
SEM image of swine liver at 20,000× magnification showing clusters of nanopores ranging in size from 150 nm to 400 nm (Image courtesy of Dr. E. Lee, UCLA Radiology)
This induced potential is dependent on a variety of conditions such as tissue type and cell size. There are also a number of pulse parameters that affect V m and thus the degree of electroporation, such as the pulse shape, duration, number, and repetition rate. Weaver et al. illustrated how an applied electric field in tissue affects the transmembrane potential of its cells and the equivalent circuit of tissue undergoing electroporation [14]. The microscopic electric field distribution is difficult to determine analytically due to tissue anisotropy and the distribution of electrical impedance within the tissue. The issue is further complicated because the bioelectrical properties will change due to minor localized heating of the tissue from the pulses and due to the cells becoming permeable as a result of treatment [15, 16]. However, for a specific tissue type, the macroscopic electric field distribution to which the tissue is exposed is the primary parameter [17]. Therefore, knowledge of the electric field distribution in tissue is critical in optimizing pulse parameters for in vivo applications, which is described in a subsequent section.
First Preclinical Prostate Study Findings
The first preclinical IRE study on focal prostate ablation [18] was performed in six male canines in which ablations were created in normal prostates using transrectal ultrasound (TRUS) and either 18-gauge monopolar electrodes or bipolar electrodes. These animals were followed for either one or 14 days. The high-voltage energy source was a DC power supply (Oncobionic Inc.) designed for preclinical IRE studies that delivered pulses up to 100 μs in duration, with a variable pulse interval and voltage range. There were several key findings made in this pioneering study by Onik et al. [18]. This study validated that pancuronium bromide (administered at 0.1 mg/kg) provides a sufficient muscle blockade to insure safely diminished amounts of involuntary muscular response to the applied high voltages. As is pointed out by the authors, this detail is important because it significantly reduces the likelihood that the subject will experience internal tissue damage from an electrode puncture wound. This became the standard muscle blockade protocol for all subsequent preclinical studies. From one to eighty pulses of 100 μs in duration at 1,000 V/cm and greater electric field strengths were well tolerated by the animals with no reported morbidity.
At an electric field strength of 1,000 V/cm, histology demonstrated that the urethra’s structural integrity was preserved without evidence of necrosis within the submucosa despite necrotic glandular tissue evidenced adjacent to the urethra. Furthermore, this first study in prostatic tissue found that the lumen of vessels receiving eighty pulses in an electric field of 1,500 V/cm remained patent and without evidence of thrombosis. Nerves within neurovascular bundles, in zones of ablated tissue, appeared to be intact and unaffected by the ablation process. Regional lymph nodes were enlarged in the drainage area. After only 2 weeks, zones of hemorrhagic tissue had mostly resolved within the zones of ablated tissue. Marked reductions in the volumes of ablated tissue were noted compared to untreated tissue. Rectal tissue layers adjacent to zones of ablated tissue appeared viable with no evidence of fistula formation. This first preclinical prostate study corroborated several findings from an earlier preclinical study in normal swine hepatic tissue [19] that demonstrated rapid IRE ablation zone resolution, sparing of critical structures such as vessels, and uniform destruction throughout the IRE zone of ablation.
Study of 2007
The 1376 study was a 30-day chronic study conducted in five adult male canines to explore the effect on prostatic tissue of 90 pulses of 100 μs in duration delivered with monopolar electrodes spaced 1 cm apart with 1,000 V between them (also referred to simply as a 1,000 V/cm parameter). The system used in this study was the Oncobionic Inc. System which was essentially a prototype version of the NanoKnife® Low Energy Direct Current (LEDC) System (AngioDynamics Inc., New York). Probe pairs were placed in the prostate with a transcutaneous perianal to transperineal approach with TRUS guidance. A biplane TRUS probe was employed to enable both sagittal and axial views. IRE probes used have a variable length insulation that was adjusted to set the length of the treatment zone. The first two pairs were placed 1 cm apart to form the first treatment zone. All tissue between them was intended to be ablated. Successive adjacent treatment zones were created to cause overlapping treatment volumes to sufficiently cover the targeted treatment volume. Once the treatment probes were in the correct position, a 22-gauge spinal needle was placed into Denonvilliers’ fascia, and approximately 25 cc of 5% dextrose (D5W) was injected into Denonvilliers’ fascia to separate the rectum from the prostate capsule. This technique has been referred to elsewhere in the medical literature [20] such as in radio frequency ablation (RFA) procedures as “hydrodissection” and is to create a safety margin by distancing the energy source from tissue to be protected. Immediately after the hydrodissection was completed, the pulse sequences were applied to each pair of probes as determined in the planning phase of the procedure. This is important because the spacing initially created by the hydrodissection will not remain for too long because the injected D5W dissipates as there is no barrier keeping the fluid in place. This hydrodissection protocol became standard in all subsequent preclinical prostate studies. All animals tolerated the ablation procedure well.
Nerve tissue remained largely unremarkable, although the nerves and ganglia were surrounded by increased fibrous and inflammatory tissue (Fig. 23.3).
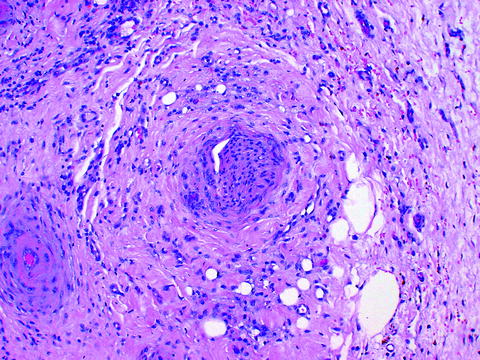
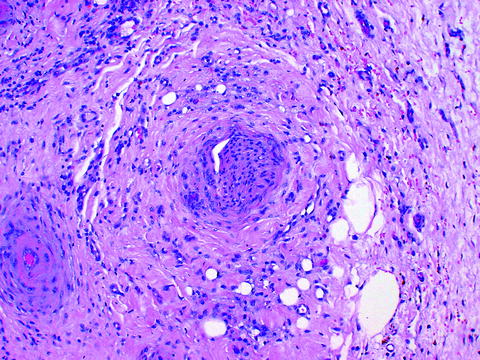
Fig. 23.3
Unremarkable nerve tissue in center surrounded by increased fibrous and inflammatory tissue
All aspects of the rectal tissue in these five animals were unremarkable or undamaged. From 20% to 50% of the glandular tissue in these animals was unremarkable, indicating that the overall applied electric field strength was below the ablation threshold for much of this tissue. Despite this fact, the prostates in this study shrank an average of 54% in the 30-day chronic animals, suggesting that this type of IRE ablation could potentially be beneficial to treat benign prostatic hypertrophy (BPH) once lower percentages of unremarkable tissue are achieved with refinements of the ablation technique or devices (Figs. 23.4 and 23.5).
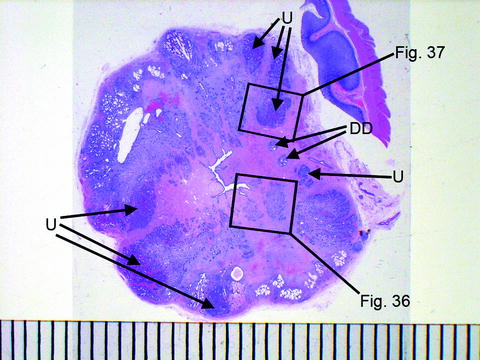
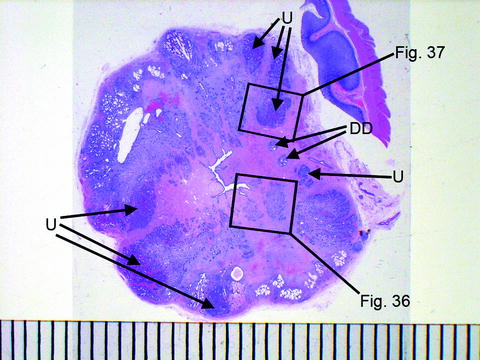
Fig. 23.4
Prostate cross section showing urethra in center and scattered zones of unremarkable tissue. Scale is in mm; DD deferent ducts, U unremarkable glandular tissue
Study of 2007
The 1393 study was a 3-day chronic study conducted in five adult male canines to explore the improvement in ablation efficiency at an electric field strength of 1,500 V/cm versus 1,000 V/cm. This study used the early NanoKnife LEDC System for all ablations. All prostatic tissue was ablated with 90 pulses of 100 μs in duration in monopolar electrodes spaced 1 cm apart with 1,000 V between them for ablations in the right hemi-prostate and 1,500 V between them for ablations in the left hemi-prostate of each animal. There was a 250-ms delay between each pulse and a delay of 3 s every ten pulses. All animals were treated successfully, tolerated the procedure well, and survived to their intended endpoint. This study contained more observations of viable blood vessels and nerves in zones of ablated tissue. The left hemi-prostates had greater volumes of ablated tissue (treated at 1,500 V/cm) than the right hemi-prostates (treated at only 1,000 V/cm) validating the utility and safety of employing greater electric field strengths on the order of 1,500 V/cm.
Study of 2009
This study also used a NanoKnife LEDC System. Standard anesthesia and muscle blockade techniques were used. The 9001 study was a 21-day chronic study conducted in 4 male canines to determine the safety and effectiveness of ablations with 90 pulses each with pulse duration of 70 μs. Single electrode probes whose active electrode length could be varied from 0.5 to 4.0 cm were used to form a box configuration with four parallel monopolar electrodes placed under TRUS guidance approximately one cm apart from each other in each lobe of the canines’ prostate for a total of eight ablations. The actual electrode distances from each other were 5–17 mm normally due to anatomical restrictions such as the canine’s pelvic bone causing interference. The voltage was set to 1,500 V for electrodes spaced 10 mm from each other and was adjusted accordingly for electrodes with a greater or smaller spacing.
All ablations were well tolerated and were performed without major procedural device-related complications. No major postoperative or adverse events were experienced by any of the subjects during the 21-day in-life phase of the study, and there were no instances of morbidity or mortality. Histopathology revealed no nerve damage and no significant urethral damage other than minimal to moderate submucosal hemorrhage. The shorter 70 μs pulses appeared to have the same ability to ablate tissue as the longer 100 μs pulses used previously but were evidently more efficient as they were delivering 30% less energy.
Study of 2010
The 10004 study was ultimately required in order to present unequivocal findings to the Food and Drug Administration (FDA) in support of an IDE application to perform a pilot clinical trial to demonstrate the safety of focal prostate ablations with the NanoKnife LEDC System for the treatment of prostate cancer. This has been the largest and most comprehensive preclinical IRE prostate study carried out to date. Twelve adult male canines were anesthetized and transperineally, under dual-axis TRUS guidance, were treated with three NanoKnife Single Electrode Probes (AngioDynamics Inc., New York) in each half of their prostates. The NanoKnife Single Electrode Probes are monopolar IRE electrodes with an overall working length of 15 cm in which the active electrode length can be continuously adjusted from zero to four cm but with a locking detent every 5 mm by sliding an insulating sheath back and forth along the cannula to expose more or less active electrode as is required to accomplish the desired treatment plan. The three electrodes were inserted parallel to each other to roughly form an equilateral triangle with the tips of the electrodes aligned (Fig. 23.6). The active electrode lengths of either 1.5 cm or 2.0 cm created ablation zone lengths of 2.5 cm and 3.0 cm, respectively. This is because the monopolar electrodes ablate about 0.5 cm beyond the distal tip of the electrode and about 0.5 cm behind the proximal edge of the active electrode length. This detail should be accounted for when planning any treatment (Fig. 23.7).
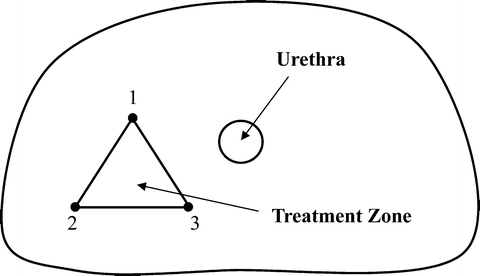
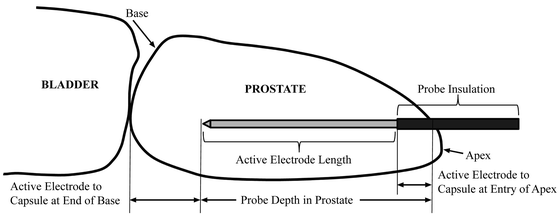
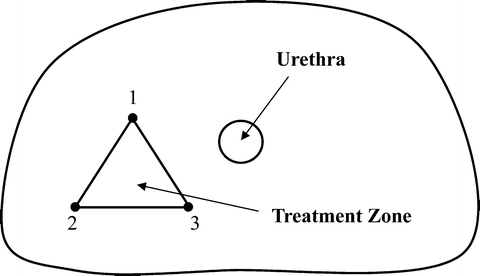
Fig. 23.6
Axial view of right prostate lobe treatment zone
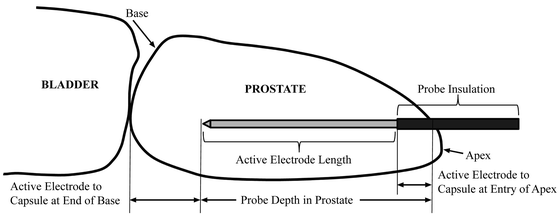
Fig. 23.7
IRE electrode placement diagram showing prostate capsule in relationship to the active electrode length (diagram not to scale)
The treatments consisted of delivering LEDC pulses from the NanoKnife LEDC System to two of the three probes until all three probe pairs had been energized to ablate local prostate tissue (probes 1–2, 1–3, and 2–3). LEDC energy was applied at 90 pulses per monopolar (single) electrode pair, 70 μs pulse duration, and electric field strength values ranging from 1,500 to 1,800 V/cm. Pancuronium bromide was administered just prior to NanoKnife LEDC System energy delivery to reduce skeletal muscle contraction. Animals were then allowed to recover for 7 (n = 6) or 27 and 28 days (n = 6). Erectile function was assessed both prior to and at least 3 days following the prostate ablations (Table 23.1; Figs. 23.8 and 23.9). Animal health including urination, defecation, and emesis was also assessed through the termination of the in-life phase of the study. After either 7 or 27 and 28 days of recovery, the animals were subjected to a comprehensive gross necropsy in which the prostate, rectum, bladder, vas deferens, ureters, and urethra were explanted and fixed and stored in 10% neutral buffered formalin (NBF). Many other tissues that were distant from the prostate were also examined for gross lesions. Histopathology was performed using standard microhistological tissue preparation techniques and H&E staining.
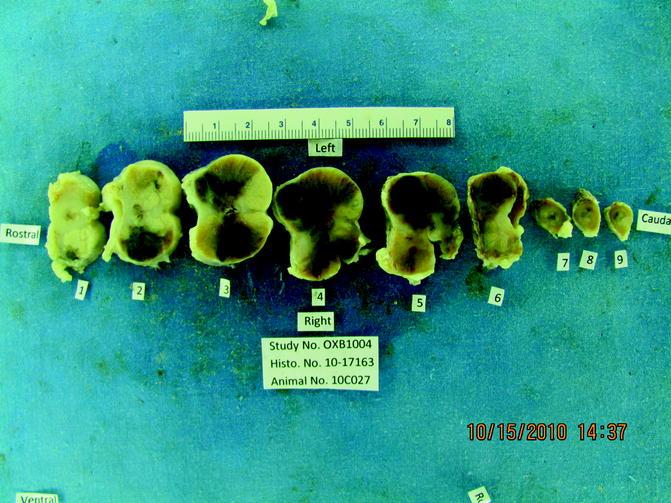
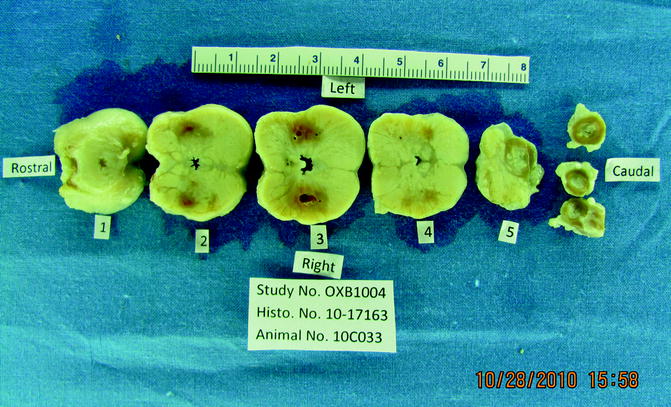
Table 23.1
Animal evaluation of erectile function before and after IRE prostate ablations; all animals successfully demonstrated potency in which attempts were made either during exercise, examinations, or with manual stimulation
Animal | Pre-ablation erectile function | Post-ablation erectile function |
---|---|---|
Number of attempts | ||
10C022, 10C024 | 1 | 3 |
10C023,10C025,10C026, 10C028 | 2 | 3 |
10C027,10C029,10C030, 10C033 | 3 | 2 |
10C031 | 2 | 2 |
10C032 | 1 | 1 |
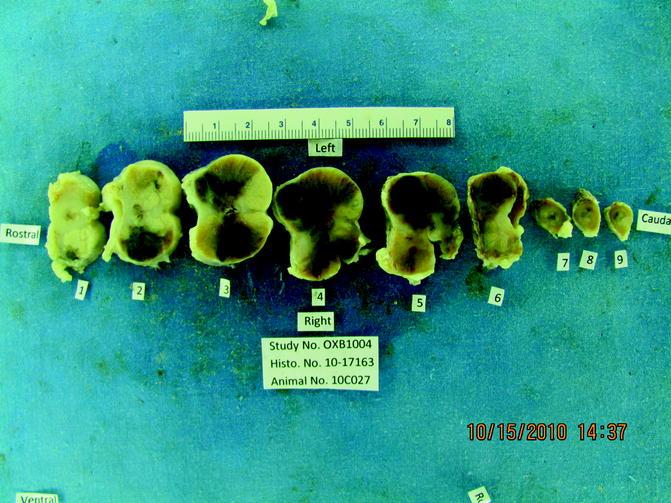
Fig. 23.8
Cross section of one of the 7-day animal prostates. The dark areas clearly visible in the central sections of the 7-day prostate represent hemorrhage and necrotic detritus
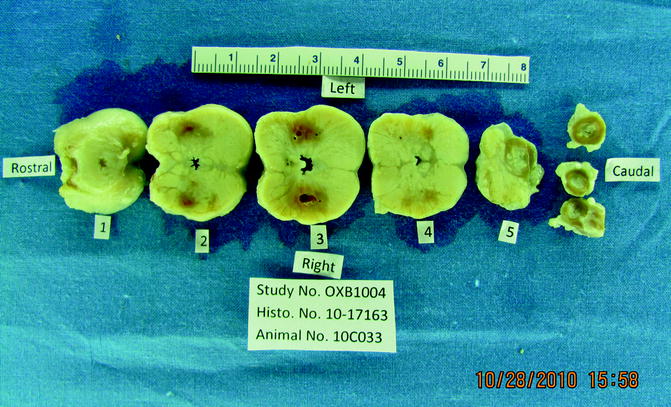
Fig. 23.9
Characteristic cross section of one of the 30-day animal prostates. From the 30-day image, it is evident that the hemorrhage and nearly all of the necrotic detritus are gone by 30 days. A significant amount of the glandular tissue has been replaced by fibrous tissue at 30 days
Prostate ablations were successfully carried out in all animals as per the protocol, and all animals survived to their respective study endpoints. Although relatively large areas of prostate exhibited necrosis, hemorrhage, inflammation, and edema at 7 days, numerous alveoli, tubules, and ducts remained viable not only at the periphery of the ablated zones but within the ablated zones as well. The portion of the prostatic lobe that had been completely replaced by fibrous connective tissue at 30 ± 3 days was difficult to measure, but generally, it was quite small (<10%). Viable glandular tissue remained in most areas although the alveoli, ducts, and tubules were separated by increased interstitial fibrosis. The IRE ablations resulted in no significant lesions in the blood vessels or nerves leading to or from the prostate or in the tissues surrounding the prostate (Fig. 23.10).
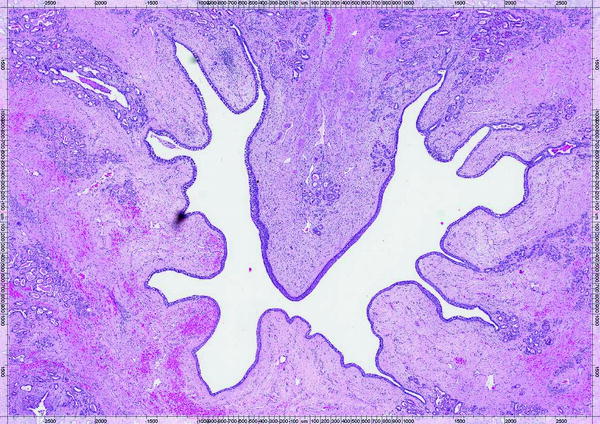
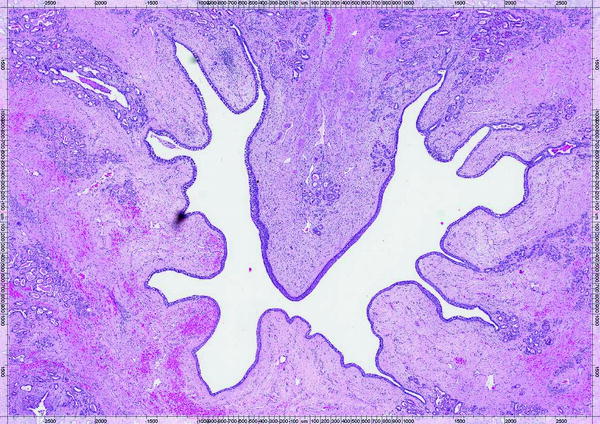
Fig. 23.10
Prostate cross-sectional image of 7-day animal showing mild submucosal hemorrhage surrounding the urethral lumen but with urethral epithelium intact; capsular blood vessels and nerves were unremarkable
The total fraction of the prostate ablated in these animals ranged from 40% to 87% (Table 23.2). The pre-ablation prostate volume was calculated using the conventional prolate ellipsoid formula (length × height × width × [π/6]) with measurements taken from an intraprocedural TRUS examination [21]. The ablation volumes were taken from TRUS-measured electrode probe spacings, and three-dimensional COMSOL 4.1 finite element analysis (FEA) models were used to correct the volumetric estimates made from ablation cross-sectional area estimates that were taken from the NanoKnife LEDC System software.
Table 23.2
Prostate volumes ablated as fraction of total prostate volume by animal
Animal |
---|