The chest radiograph is one of the most commonly obtained examinations in pediatric imaging. It is also the examination most likely to be encountered by radiology residents, pediatric residents, general radiologists, and pediatricians. Therefore topics such as chest imaging in neonates and the evaluation of suspected pneumonia are discussed in detail.
▪ Neonatal Chest
Causes of respiratory distress in newborn infants can be divided into those that are secondary to diffuse pulmonary disease (medical causes) and those that are secondary to a space-occupying mass compressing the pulmonary parenchyma (surgical causes).
Diffuse Pulmonary Disease in the Newborn
Diffuse pulmonary disease causes respiratory distress much more commonly than surgical diseases, particularly in premature infants, who make up the majority of cases of respiratory distress in the newborn. A simple way to evaluate these patients and try to offer a limited differential diagnosis is to evaluate the lung volumes and to characterize the pulmonary opacities.
Lung volumes can be categorized as high, normal, or low. Normally the apex of the dome of the diaphragm is expected to be at the level of approximately the tenth posterior rib. If present, lung opacity can be characterized as streaky, perihilar (central) densities that have a linear quality or as diffuse, granular opacities that have an almost sandlike character. Classically cases fall into one of the following two categories: (1) cases with high lung volumes and streaky perihilar densities and (2) cases with low lung volumes and granular opacities ( Table 3-1 ). This is more of a guideline rather than a rule because many neonates with diffuse pulmonary disease have normal lung volumes. The differential diagnosis for cases with high lung volumes and streaky perihilar densities includes meconium aspiration, transient tachypnea of the newborn (TTN), and neonatal pneumonia. Most of the neonates in this group are term. The differential for cases with low lung volumes and granular opacities includes surfactant deficiency and group B streptococcal pneumonia. Most of these neonates are premature.
Meconium Aspiration Syndrome
Meconium aspiration syndrome results from intrapartum or intrauterine aspiration of meconium. It usually occurs secondary to stress, such as hypoxia, and more often occurs in term or postmature neonates. The aspirated meconium causes both obstruction of small airways secondary to its tenacious nature and chemical pneumonitis. The degree of respiratory failure can be severe and may lead to respiratory failure, pulmonary air leaks, and persistent pulmonary hypertension. Radiographic findings include hyperinflation (high lung volumes), which may be asymmetric and patchy, and asymmetric lung densities that tend to have a ropy appearance and a perihilar distribution ( Fig. 3-1 ). Commonly there are areas of hyperinflation alternating with areas of atelectasis. Pleural effusions can be present. Because of the small-airway obstruction by the meconium, air-block complications are common, with pneumothorax occurring in 20% to 40% of cases. Meconium aspiration syndrome is relatively common; 25,000 to 30,000 cases and 1000 deaths occur in the United States annually. Although meconium-stained amniotic fluid occurs in approximately 10% to 15% of deliveries, meconium aspiration syndrome develops in less than 5% of them.
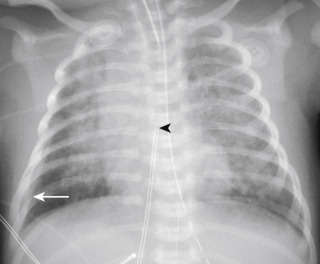
Transient Tachypnea of the Newborn
TTN is also referred to by a variety of other names, including wet lung disease and transient respiratory distress. It occurs secondary to delayed clearance of fetal lung fluid. Physiologically the clearing of fetal lung fluid is facilitated by the “thoracic squeeze” during vaginal deliveries; therefore most cases of TTN are related to cesarean section, in which the thoracic squeeze is bypassed. Other causes include maternal diabetes and fetal distress requiring anesthesia or analgesia. The hallmark of TTN is a benign course. Respiratory distress develops by 6 hours of age, peaks at 1 day of age, and is resolved by 2 to 3 days. There is a spectrum of radiographic findings similar to those seen with mild-to-severe pulmonary edema. There is a combination of airspace opacification, interstitial edema, prominent and indistinct pulmonary vasculature, fluid in the fissures, pleural effusion, and cardiomegaly ( Fig. 3-2 ). Lung volumes are normal to increased.
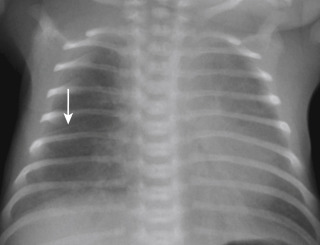
Neonatal Pneumonia
Neonatal pneumonia can be caused by a large number of infectious agents that can be acquired intrauterine, during birth, or soon after birth. With the exception of group B streptococcal pneumonia, which will be discussed separately, the radiographic appearance of neonatal pneumonia is that of patchy, asymmetric perihilar densities and hyperinflation ( Fig. 3-3 ). Pleural effusions may be present ( Fig. 3-4 ). Such cases of neonatal pneumonia may have a similar radiographic appearance to and be indistinguishable from meconium aspiration syndrome, TTN, or surfactant-deficient disease (SDD) when using imaging alone.
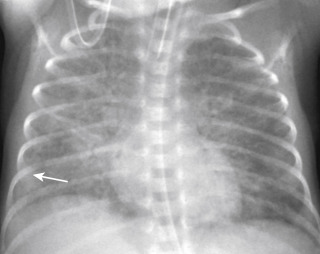
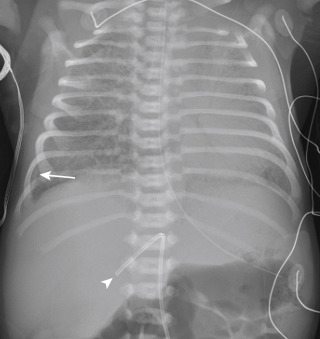
Surfactant-Deficient Disease
SDD, or respiratory distress syndrome or hyaline membrane disease, is a common disorder, with approximately 40,000 new cases annually in the United States. It is primarily a disease of premature infants, affecting up to 50% of them, and it is the most common cause of death in live newborns. The risk of SDD increases with decreasing gestational age. SDD is related to the inability of premature type II pneumocytes to produce surfactant. Normally surfactant coats the alveolar surfaces and decreases surface tension, allowing for the alveoli to remain open. As a result of the lack of surfactant, there is alveolar collapse, resulting in noncompliant lungs. The radiographic findings reflect these pathologic changes ( Fig. 3-5, A and B ). Lung volumes are low. There are bilateral granular opacities that represent collapsed alveoli interspersed with open alveoli. Because the larger bronchi do not collapse, there are prominent air bronchograms. When the process is severe enough and the majority of alveoli are collapsed, there may be coalescence of the granular opacities, resulting in diffuse lung opacity. A normal film at 6 hours of age excludes the presence of SDD.
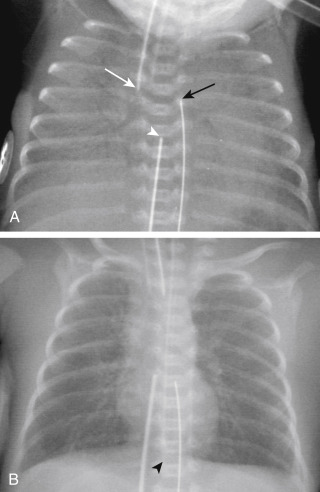
Surfactant Replacement Therapy
One of the therapies for SDD is surfactant administration. Surfactant can be administered via nebulized or aerosol forms. It is administered into the trachea via a catheter or an adapted endotracheal tube. The administration of surfactant in neonates with SDD is associated with decreased oxygen and ventilator setting requirements, decreased air-block complications, decreased incidence of intracranial hemorrhage and bronchopulmonary dysplasia (BPD), and decreased death rate. However, there is an associated increased risk for development of patent ductus arteriosus and pulmonary hemorrhage, and there can be an acute desaturation episode in response to surfactant administration. Surfactant administration can be given on a rescue basis when premature neonates develop respiratory distress or can be given prophylactically in premature infants who are at risk. Prophylactic administration is commonly given immediately after birth and is becoming a more common practice. In response to surfactant administration, radiography may demonstrate complete, central, or asymmetric clearing of the findings of SDD (see Fig. 3-5 ). There is usually an increase in lung volumes. Neonates without radiographic findings of a response to surfactant have poorer prognoses than those who have radiographic evidence of a response. A pattern of alternating distended and collapsed acini may create a radiographic pattern of bubblelike lucencies that can mimic pulmonary interstitial emphysema. Knowledge of when surfactant has been administered is helpful to render accurate interpretation of chest radiographs taken in the neonatal intensive care unit (NICU).
Group B Streptococcal Pneumonia
Group B (GBS) streptococcal pneumonia is the most common type of pneumonia in neonates. The infection is acquired during birth, and at least 25% of women in labor are colonized by the organism. Premature infants are more commonly infected than are term infants. In contrast to the other types of neonatal pneumonias, the radiographic findings include bilateral granular opacities and low lung volumes ( Fig. 3-6 ), the identical findings in SDD. The presence of pleural fluid is a helpful differentiating factor because it is very uncommon in surfactant deficiency but has been reported in between 25% and 67% of cases of β-hemolytic streptococcal pneumonia.
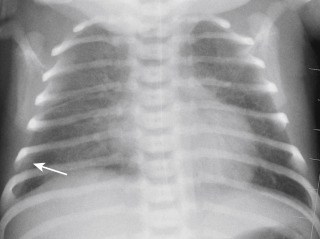
Persistent Pulmonary Hypertension of the Newborn
Persistent pulmonary hypertension of the newborn (PPHN), or persistent fetal circulation, is a term often used in the NICU and is addressed here because it can be a source of confusion. The high pulmonary vascular resistance that is normally present in the fetus typically decreases during the newborn period. In normal conditions, after clamping the cord and with the first breaths, there is a drastic drop in pulmonary vascular resistance permitting the circulation that in utero bypasses the lungs, to perfuse the lungs. If this drastic decrease in pulmonary vascular resistance does not take place, blood would continue to bypass the lungs and pulmonary pressures would remain abnormally high; the condition is termed persistent pulmonary hypertension . It is a physiologic finding rather than a specific disease. It can be a primary phenomenon or it can occur secondary to causes of hypoxia, such as meconium aspiration syndrome, neonatal pneumonia, or pulmonary hypoplasia associated with congenital diaphragmatic hernia (CDH). These patients are quite ill. The radiographic patterns are variable and are more often reflective of the underlying cause of hypoxia than the presence of persistent pulmonary hypertension. Occasionally it may be seen in neonates with clear chest radiographs, and in these cases it is referred as primary PPHN.
Neonatal Intensive Care Unit Support Apparatus
One of the primary roles of chest radiography in the NICU is to monitor support apparatus. They include endotracheal tubes, enteric tubes, central venous lines, umbilical arterial and venous catheters, and extracorporeal membrane oxygenation (ECMO) catheters. The radiographic evaluation of many of these tubes is the same as that seen in adults and is not discussed here. When evaluating the positions of endotracheal tubes in premature neonates, it is important to consider that the length of the entire trachea may be only approximately 1 cm. Keeping the endotracheal tube in the exact center of such a small trachea is an impossible task for caregivers, and phone calls and reports suggesting that the tube needs to be moved 2 mm proximally may be more annoying than helpful. Direct phone communication may be more appropriately reserved for times when the tube is in a main bronchus or above the thoracic inlet. There is an increased propensity for inadvertent esophageal intubation to occur in neonates as compared with in adults. Although it would seem that esophageal intubation would be incredibly obvious clinically, this is not always the case. The authors have experienced cases in which a child has in retrospect been discovered to have been esophageally intubated for more than 24 hours. Therefore the radiologist may be the first to recognize esophageal intubation. Obviously, when the course of the endotracheal tube does not overlie the path of the trachea, the use of esophageal intubation is fairly obvious. Other findings of esophageal intubation include a combination of low lung volumes, gas within the esophagus, and gaseous distention of the bowel ( Fig. 3-7 ).
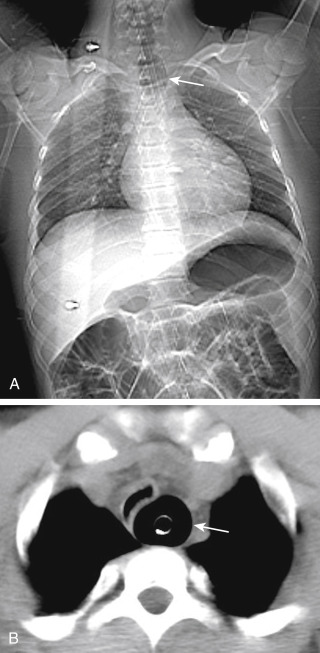
Umbilical Arterial and Venous Catheters
Umbilical arterial and venous catheters are commonly used in the NICU. Umbilical arterial catheters pass from the umbilicus inferiorly into the pelvis via the umbilical artery to the iliac artery. The catheters then turn cephalad within the aorta (see Fig. 3-5 ). These catheters can be associated with thrombosis of the aorta and its branches. Therefore it is important to avoid positioning the catheter with the tip at the level of the branches of the aorta (celiac, superior mesenteric, and renal arteries) or at the origin of the great arteries (arch). There are two acceptable umbilical arterial catheter positions: high lines have their tips at the level of the descending thoracic aorta (T8-T10) and low lines have their tips below the level of L3. The catheter tip should not be positioned between T10 and L3 because of the risk for major arterial thrombosis. There is no clear consensus as to whether a high or low umbilical artery catheter line is better, and both positions are still currently used, although some authors advocate that the high position has fewer complications.
The pathway of the umbilical venous catheter is umbilical vein to left portal vein to ductus venosus, the most superior portion of the ductus venosus joins, or becomes part of, one of the hepatic veins as they enter the inferior vena cava ( Fig. 3-8 ). In contrast to umbilical arterial catheters, the course is in the superior direction from the level of the umbilicus. The ideal position of an umbilical venous catheter is with its tip at the junction of the right atrium and the inferior vena cava at the level of the hemidiaphragm (see Figs. 3-4 and 3-5 ). The umbilical venous catheter may occasionally deflect into the portal venous system rather than passing into the ductus venosus. Complications of such positioning can include hepatic hematoma or abscess.
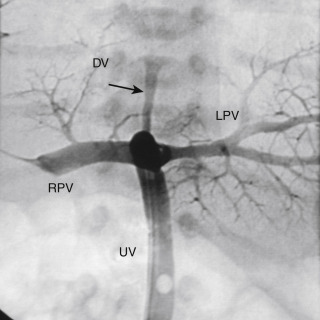
Peripherally Inserted Central Catheters in Children
One of the more common lines now seen in children, as in adults, is peripherally inserted central catheters (PICCs). In contrast to adults, in whom some of the PICCs can be as large as 6F, the PICC lines used in children, particularly infants, are often small in caliber (2F or 3F) so that they can be placed into their very small peripheral veins. These small-caliber PICCs can be very difficult to see on chest radiography, so some of them must be filled with contrast to be accurately visualized. The tip of the PICC line that enters the child from the upper extremity or scalp should be positioned with the tip in the midlevel of the superior vena cava (see Fig. 3-27 ). It is essential that PICC lines not be left in place with the tip well into the right atrium. Particularly with the small-caliber lines, the atrium can be lacerated, leading to pericardial tamponade, free hemorrhage, or death. Many such cases have been reported nationally. In addition, the PICC should not be too proximal in the superior vena cava because the distal portion of the line can flip from the superior vena cava into the contralateral brachiocephalic or jugular vein. At many children’s hospitals, the PICC lines are inserted in a dedicated interventional radiology suite by a team of nurses, with supervision by pediatric interventional radiologists. Ultrasound is often used to guide vein cannulation, and certified child-life specialists coach most kids through the procedure often without having to sedate them. Fluoroscopy is used at the end of the procedure to adjust and document tip position in the cavoatrial junction.
Extracorporeal Membrane Oxygenation
ECMO is a last-resort therapy usually reserved for respiratory failure that has not responded to other treatments. ECMO is essentially a prolonged form of circulatory bypass of the lungs and is used only in patients who have reversible disease and a chance for survival. The majority of neonates who are treated with ECMO have respiratory failure as a result of meconium aspiration, persistent pulmonary hypertension (resulting from a variety of causes), severe congenital heart disease, or CDH. ECMO seems to be used less commonly now than it was in the 1990s.
There are two types of ECMO: arteriovenous and venovenous. In arteriovenous ECMO, the right common carotid artery and internal jugular veins are sacrificed. The arterial catheter is placed via the carotid and positioned with its tip overlying the aortic arch. The venous catheter is positioned with its tip over the right atrium ( Fig. 3-9 ). One of the main roles of a chest radiograph of children on ECMO is to detect any potential migration of the catheters. Careful comparison with previous studies to make sure that the catheters are not coming out or moving too far in is critical. These patients have many bandages and other items covering the external portions of the catheters, so migration may be hard to detect on physical examination. In addition, anasarca is invariable during ECMO because the increasing soft tissue edema may result in accidental dislodgement and subsequent accidental decannulation. Note that there are various radiographic appearances of the ECMO catheters. Some catheters end where the radiopaque portion of the tube ends, and others have a radiolucent portion with a small metallic marker at the tip (see Fig. 3-9 ). It is common to see white-out of the lungs soon after a patient is placed on ECMO as a result of sudden decrease in airway pressure, decreased ventilator settings, and third-space shifting of fluid (see Fig. 3-9 ). Patients on ECMO are anticoagulated and are therefore at risk for hemorrhage.
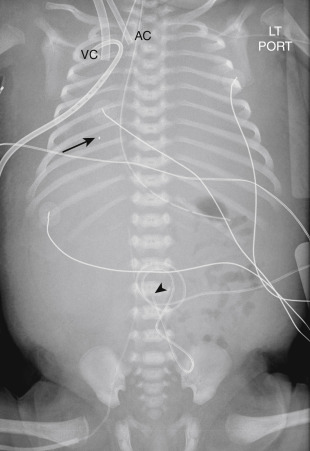
Types of Ventilation: High-Frequency Oscillator versus Conventional Ventilation
High-frequency oscillators are commonly used to treat neonates in the NICU. In contrast to conventional ventilation, high-frequency oscillators use supraphysiologic rates of ventilation with very low tidal volumes. Conventional ventilation has been likened to delivering a cupful of air approximately 20 times a minute. In contrast, high-frequency oscillation is like delivering a thimbleful of air approximately 1000 times per minute. The air is vibrated in and out of the lung, and appropriate gas exchange occurs at significantly lower peak inspiratory pressures than those required with conventional ventilation. The mechanism of oscillators is poorly understood. In conventional ventilation, the diaphragm moves up and down, whereas during high-frequency ventilation the diaphragm stays parked at a certain anatomic level. This level can be adjusted by changing the mean airway pressure of the oscillator. Caregivers usually like to maintain the diaphragm at approximately the level of the tenth posterior ribs. In general the radiographic appearance of neonatal pulmonary diseases is not affected by whether the patient is being ventilated by conventional or high-frequency ventilation.
Complications in the Neonatal Intensive Care Unit
As in adult intensive care units, major complications detected by chest radiographs include those related to air-leak complications, lobar collapse, or acute diffuse pulmonary consolidation. Another type of complication seen in neonates is the development of BPD. Imaging findings of lobar collapse and air-block complications, such as pneumothorax, pneumomediastinum, and pneumopericardium, are similar in neonates and adults. One type of air-block complication that is unique to neonates is pulmonary interstitial emphysema.
Pulmonary Interstitial Emphysema
In patients with severe surfactant deficiency, ventilatory support can result in marked increases in alveolar pressure, leading to perforation of alveoli. The air that escapes into the adjacent interstitium and lymphatics is referred to as pulmonary interstitial emphysema (PIE). PIE appears on radiographs as bubblelike or linear, nonbranching lucencies and can be focal or diffuse ( Fig. 3-10 ). The involved lung is usually noncompliant and is seen to have a static volume on multiple consecutive films. The finding is typically transient. The importance of detecting PIE is that it serves as a warning sign for other impending air-block complications, such as pneumothorax, and its presence can influence caregivers in decisions, such as switching from conventional to high-frequency ventilation.
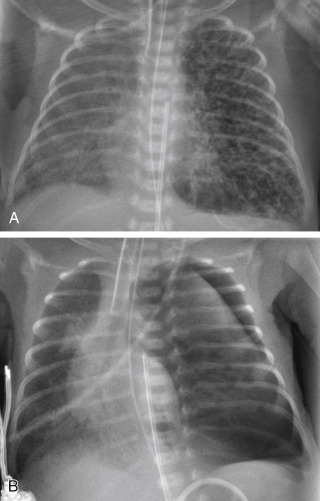
It can be difficult to differentiate diffuse PIE from the bubblelike lucencies that are associated with developing BPD. When encountering this scenario, the patient’s age can help to determine which is more likely. Most cases of PIE occur in the first week of life, a time at which BPD is very unlikely. In patients older than 4 weeks, BPD is more likely. In addition, in patients who have undergone a series of daily films, PIE may be noted to occur abruptly, whereas BPD tends to occur gradually. As previously mentioned, SDD partially treated by surfactant replacement can cause a pattern of lucencies that may mimic PIE as well.
Rarely PIE can persist and develop into an expansive, multicystic mass. The air cysts can become large enough to cause mediastinal shift and compromise pulmonary function. Often the diagnosis is indicated by sequential radiography showing evolution of the cystic mass from original findings typical of PIE. In unclear cases, computed tomography (CT) demonstrates that the air cysts are in the interstitial space by showing the bronchovascular bundles being positioned within the center of the air cysts. The bronchovascular bundles appear as linear or nodular densities in the center of the cysts.
Causes of Acute Diffuse Pulmonary Consolidation
Acute diffuse pulmonary consolidation is nonspecific in neonates, as it is in adults, and can represent blood, pus, or water. In the neonate the specific considerations include edema, which may be secondary to the development of patent ductus arteriosus ( Fig. 3-11 ); pulmonary hemorrhage, to which surfactant therapy predisposes; worsening surfactant deficiency (during the first several days of life but not later); or developing neonatal pneumonia ( Table 3-2 ). Diffuse microatelectasis is another possibility because neonates have the propensity to artifactually demonstrate diffuse lung opacity on low lung volume films (expiratory technique; Fig. 3-12, A and B ); this should not be mistaken for another cause of consolidation. Such radiographs showing low lung volumes offer little information concerning the pulmonary status of the patient and should be repeated when clinically indicated.
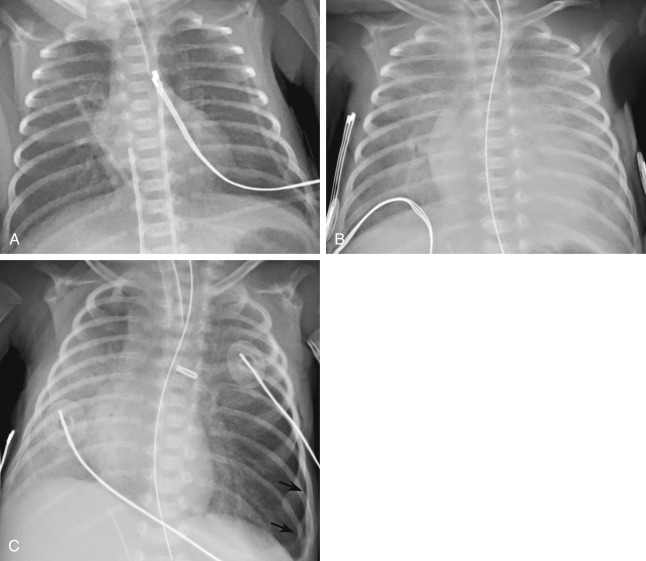
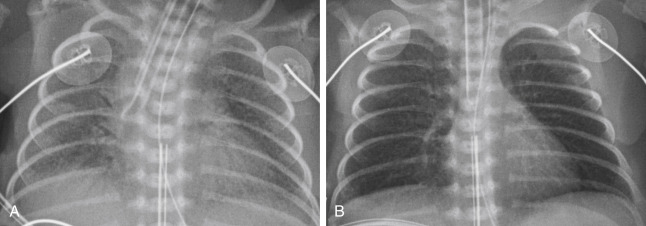
Bronchopulmonary Dysplasia
BPD, or chronic lung disease of prematurity, is a common complication, seen in premature infants and is associated with significant morbidity rates. BPD typically occurs in neonates treated with oxygen and positive pressure ventilation for respiratory failure, usually SDD. The definition of BPD has evolved over time; currently, the most accepted definition is that of oxygen dependence for at least 28 days after birth, whereas the severity is graded in proportion to the respiratory support required at term. It is uncommon in children born at greater than 32 weeks of gestational age, but it occurs in more than 50% of premature infants born at less than 1000 g. BPD is the most common chronic lung disease of infancy.
BPD is related to injury to the lungs that is thought to result from some combination of mechanical ventilation and oxygen toxicity. Although four discrete and orderly stages of the development of BPD were originally described, they are not seen commonly and are probably not important to know. BPD typically occurs in a premature infant who requires prolonged ventilator support. At approximately the end of the second week of life, persistent hazy density appears throughout the lungs. Over the next weeks to months a combination of coarse lung markings, bubblelike lucencies, and asymmetric aeration can develop ( Fig. 3-13 ). Eventually, focal lucencies, coarse reticular densities, and bandlike opacities develop. In childhood survivors of BPD, many of these radiographic findings decrease in prominence over the years and only hyperaeration may persist. The radiographic findings may completely resolve. Clinically, many children with severe BPD during infancy may eventually improve to normal pulmonary function or may only have minor persistent problems such as exercise intolerance, predisposition to infection, or asthma.
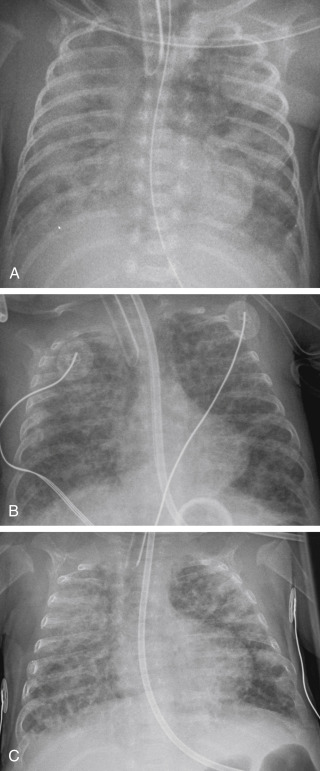
Wilson-Mikity syndrome is a confusing and controversial term. It historically refers to the development of BPD in the absence of mechanical ventilation. It is now believed to be related to an underlying alveolar growth disorder.
Focal Pulmonary Lesions in the Newborn
In contrast to diffuse pulmonary disease in newborns, focal masses can present with respiratory distress due to compression of otherwise normal lung. Most of these focal masses are related to congenital lung lesions. Congenital lung lesions may appear solid, as air-filled cysts, or mixed in appearance. The differential for a focal lung lesion can be separated on the basis of whether the lesion is lucent or solid appearing on chest radiography ( Table 3-3 ). The most likely considerations for a lucent chest lesion in a newborn are congenital lobar emphysema, congenital pulmonary airway malformation (CPAM) persistent PIE, and CDH. CT may be helpful in differentiating among these lesions by demonstrating whether the abnormal lucency is related to air in distended alveoli, in the interstitium, or in abnormal cystic structures. Lesions that typically appear solid during the neonatal period include sequestration and bronchogenic cyst. Many of these lesions can present in children beyond the neonatal period, and those aspects of these entities are also discussed here.
The following sections are divided into specific congenital lesions. However, it has been increasingly recognized that there can be mixed or hybrid lesions, which show characteristics of more than one type of lesion (see Fig. 3-16 ). The most common mixed lesions are those that show characteristics of both congenital pulmonary airway malformation (CPAM) and sequestration. Currently, the majority of the congenital lung lesions are believed to be the result of fetal airway obstruction with varying degree of associated dysplastic changes. This explains the significant overlap of imaging findings of the various lesions. Bronchial atresia is the most commonly identified type of lesion on resected specimens and is found in 69% of CPAM lesions and in 100% of bronchopulmonary sequestrations.
It is also worth mentioning that there has been a change in the way these lesions present that is related to the increased use of prenatal ultrasound and magnetic resonance imaging (MRI). Historically, congenital lung lesions were identified when the infant became symptomatic. Indeed, the most common clinical presentation in cases of underlying bronchopulmonary sequestration was that of a young adult with recurrent pyogenic pneumonias of progressive severity. In the current clinical algorithms, most of the congenital lung lesions we currently see are identified and followed through fetal life, with additional postnatal imaging obtained shortly after birth. A significant number of these children are asymptomatic. This has raised issues related to when and whether to perform surgical treatment in infants with asymptomatic lesions.
Congenital Lobar Emphysema
Also referred to as congenital lobar hyperinflation, congenital lobar overinflation, and infantile lobar emphysema, congenital lobar emphysema is related to overexpansion of alveoli, but the mechanism is debated. The alveolar walls are maintained; therefore there is not true emphysematous change. Some reports suggest a ball-valve type of anomaly in the bronchus leading to the affected lung, which causes progressive air trapping. Most cases present with respiratory distress during the neonatal period; 50% present within the first month, and 75% present within the first 6 months of life. There can be associated anomalies, usually cardiac, but they occur in the minority of patients with congenital lobar emphysema. There is a lobar predilection; the most common site is the left upper lobe (43%), followed by the right middle lobe (35%) and right upper lobe (21%), with less than 1% in each of the other lobes. On chest radiography a hyperlucent, hyperexpanded lobe is seen ( Fig. 3-14, A and B ). On initial radiographs the lesion may appear to be a soft tissue density because of retained fetal lung fluid. This density resolves and is replaced by progressive hyperlucency. On CT the air is in the alveoli, so the interstitial septa and bronchovascular bundles are at the periphery (not the center) of the lucency (see Fig. 3-14 ). The air spaces are larger than those in the adjacent normal lung, and the pulmonary vessels appear attenuated. The treatment is lobectomy.
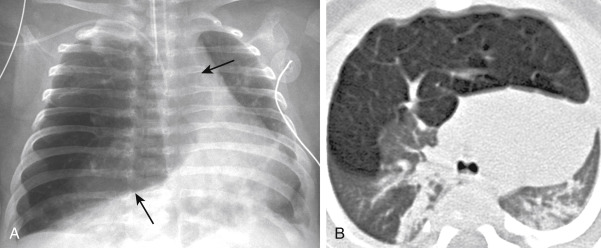
Congenital Pulmonary Airway Malformation
CPAM, or CCAM or dysplastic lesions caused by overgrowth of mesenchymal elements and airway maldevelopment, is a congenital adenomatoid proliferation that replaces normal alveoli. The majority are detected prenatally or are present with respiratory distress at birth. Most involve only one lobe and, in contrast to congenital lobar emphysema, there is no lobar predilection. CPAMs were originally classified by Stocker into three types based on cyst size and microscopic similarities to presumed sites of origin of the malformation along the airway. Type 1 shows cysts greater than 2 cm with probable bronchial or bronchiolar origin; type 2 shows more uniform cysts measuring less than 2 cm with probable bronchiolar origin; and type 3 appears solid or shows very small cysts (<0.2 cm) with probable bronchiolar or alveolar origin. Subsequently, Langston developed a more comprehensive pathologic classification of all congenital lung malformations, which is the most accepted at present. In this classification the type 1 CPAM is named large cyst type and the type 2 CPAM is named small cyst type based on similar cyst size and histologic criteria. In this classification type 3 CPAM is excluded because it is considered to represent a form of pulmonary hyperplasia. Stocker later expanded his CPAM classification to include five types; however, the Langston classification is the most currently accepted because it correlated well with findings. In fetal imaging the classification of these anomalies is also mainly based on cyst size as microcysts (<5 mm) and macrocysts (>5 mm). Given the controversial and confusing terminology, it is best to thoroughly describe the imaging findings that characterize the malformation to guide management because CPAMs can have multiple appearances when imaged. We include in our reports the gross number of cysts (e.g., single, several, or multiple), size of the largest cyst, lobe or lobes involved, degree of aeration of the CPAM, and presence or absence of an aberrant, systemic vessel. CPAMs communicate with the bronchial tree at birth and therefore fill with air within the first hours to days of life. On radiography and CT a completely cystic, mixed cystic and solid, or completely solid mass is seen depending on the number and size of cysts and whether those cysts contain air or fluid ( Figs. 3-15, A-C, and 3-16, A-C ). The management of symptomatic CPAM is surgical resection. The management of asymptomatic CPAM is currently somewhat controversial. However, many caregivers advocate elective resection because these lesions are at increased risk for infection and, rarely, type 1 or large cyst lesions may develop malignancy.
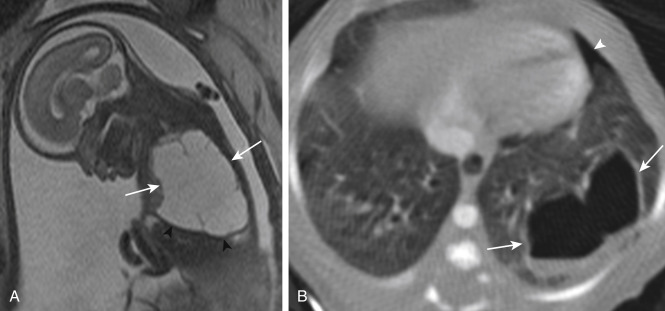
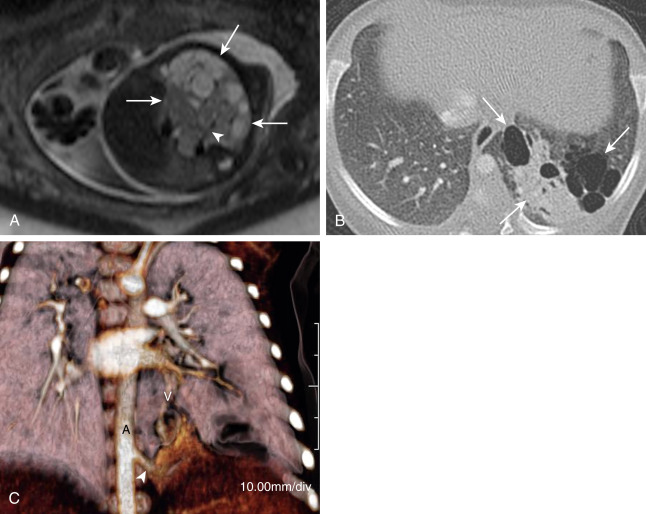
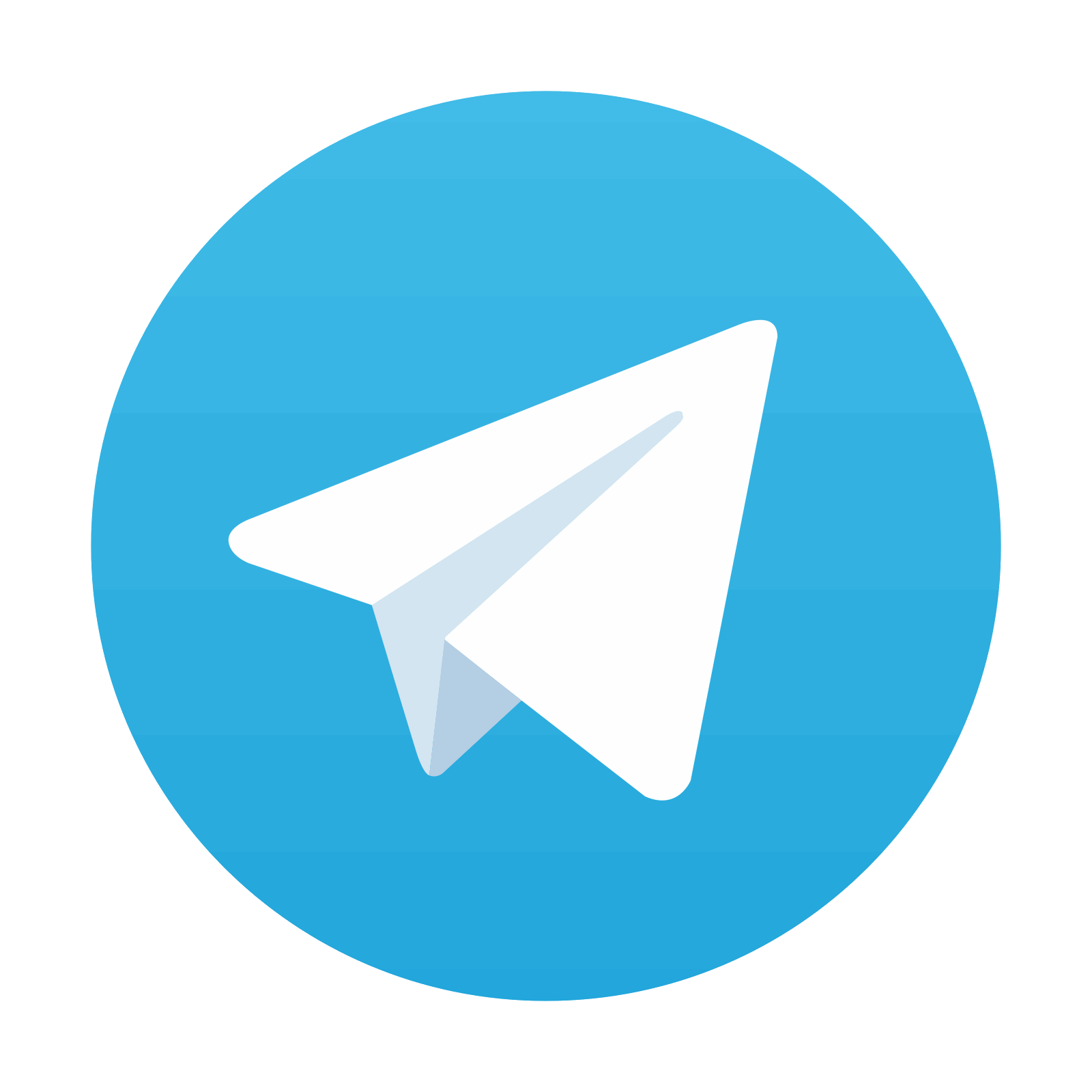
Stay updated, free articles. Join our Telegram channel

Full access? Get Clinical Tree
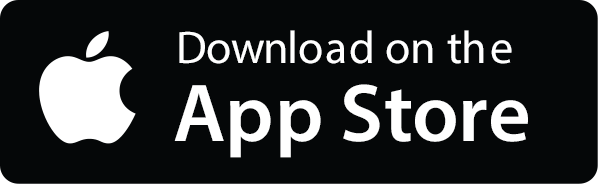
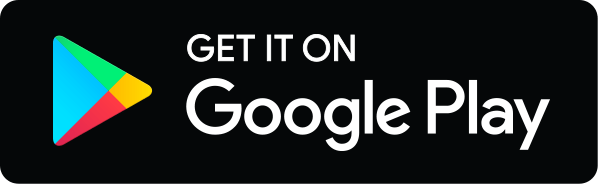
