(1)
Department of Clinical Radiology, Amiri Hospital – Kuwait City, Kuwait City, Kuwait
13.1 The Human Fascia
13.2.1 Anatomy
13.3 Imaging Signs
13.4 Facet Joint Syndrome
13.4.1 Basic Anatomy
13.4.2 Pathophysiology
13.5 Imaging Signs
13.6.1 Anatomy
13.6.2 Pathophysiology
13.8.1 Basic Anatomy
13.8.2 Pathophysiology
13.9 Imaging Signs
13.10 Imaging Signs
13.12 Imaging Signs
13.13 Cervicogenic Headache
13.13.1 Anatomy
13.13.2 Pathophysiology
13.14 Imaging Signs
13.15.1 Anatomy
13.15.2 Pathophysiology
13.16 Imaging Signs
13.17.1 Basic Anatomy
13.17.2 Pathophysiology
13.18 Imaging Signs
13.19 Thoracic Outlet Syndrome
13.19.1 Basic Anatomy
13.19.2 Pathophysiology
13.20 Imaging Signs
13.22 Imaging Signs
13.23 Lumbago
13.24 Imaging Signs
13.25 Shin Splint Syndrome
13.26 Imaging Signs
13.27 Cuboid Syndrome
13.28 Imaging Signs
13.30 Imaging Signs
13.31.1 Basic Anatomy
13.31.2 Pathophysiology
13.32 Imaging Signs
13.33 Psoas Syndrome
13.33.1 Basic Anatomy
13.33.2 Pathophysiology
13.34 Imaging Signs
13.35.1 Basic Anatomy
13.35.2 Pathophysiology
13.36 Imaging Signs
13.37 Coccydynia
13.37.1 Anatomy
13.37.2 Pathophysiology
13.39 Imaging Signs
13.1 The Human Fascia
Connective tissue makes up of about 16 % of the body’s weight and stores about 25 % of body’s total water content. Connective tissues are derived embryologically from the mesenchyme and forms the biological blocks of the skin, fascia, muscles, nerve sheaths, periosteum, aponeuroses, bones, ligaments, tendons, joint capsules, adipose tissues, blood vessels, and cartilage.
Fascia, an important form of the body’s connective tissue, encloses body tissues up to the level of the smallest nerve and muscle fibers (e.g., epineurium). Each fascial layer is enclosed by another fascial layer, like a plastic bag within a bigger plastic bag. The human body is composed of many enclosed fluid systems like plastic bags. Since any pressure applied to a liquid-enclosed system at rest from a point will be transmitted equally within this system in all direction (Pascal’s law), then we can say that an increased fluid pressure in one fascial compartment (plastic bag) can be transmitted to another fascial compartment, and vice versa.
The fascia is a continuous organ, and one can travel from any portion of the body to another and never leave the fascia. In the human body, four primary fascial layers are described:
- 1.
Pannicular fascia: it makes the superficial body fascial layer and is derived from the somatic mesenchyme; it surrounds the entire body with the exception of its orifices such as the orbits, nasal passages, and the oral openings.
- 2.
Appedicular (axial) fascia: like pannicular fascia, this fascia is also derived from somatic mesenchyme; it is fused to the panniculus peripherally and extends deep into the body forming the epimysium of skeletal muscle, the fascial layer that surrounds neurovascular bundles (e.g., the brachial plexus), the periosteum of bone, and the peritendon of the tendons.
- 3.
Meningeal fascia: it makes the meninges (pia, arachnoid, and dural layers) that surrounds the central nervous system.
- 4.
Visceral fascia: it forms the fascia that surrounds the viscera like the peritoneum, visceral pleura, perirenal (Gerota’s) fascia, and cervical fascia that surrounds the pharynx and is attached to skull base. Visceral fascia also forms visceral ligaments (e.g., ligament of Treitz). Unlike ligaments in somatic tissue, visceral ligaments typically function to carry blood supply and innervations to an organ system or to loosely anchor an organ in the body cavity.
Fascia is a continuous structure, meaning that the skull’s aponeurosis runs continuously up to the plantar fascia of the foot (fascial or meridian planes). If we think of the spine as a “bow,” then the anterior fascial planes makes the “string” of that bow (bowstring theory). The anterior prevertebral fascia extends from the skull base, continues down into the clavicles, connects to the mediastinum and upper diaphragm, and follows the falciform; from the coronary ligaments of the liver, linea alba, umbilicus, median umbilical ligament of urachus, and investing fascia of the bladder to the pelvic diaphragm (perineum); and lastly from the pelvic diaphragm to the iliotibial band.
Fascia as a tissue poses unique characteristic in the form of deformation and elongation. Fascia can show both permanent (viscous) and temporary (elstatic) deformation; also, fascia can show permanent (plastic) elongation and contraction (mechanical elongation). Many of neurons, blood vessels, and lymphatic vessels travel within fascial planes and tunnels. Therefore, fascial disease such as fibrosis, contraction, and strains can cause musculoskeletal disorders due to exerting pulling tension on the joints, impeding the flow of the interstitial fluid, and impinging on nerves, vessels, and lymphatics.
Beside the mechanical injury, fascial disorder can arise from chemical injury. For example, dehydration causes fascia to shrink, irritating or compressing the sensory nerve fibers within it. Moreover, exogenous intake of corticosteroid or estrogen in the form of oral contraceptive interferes with/inhibits collagen synthesis. Women with chronic intake of oral contraceptive have a higher risk of lower back pain, bone fracture, persistent pelvic pain, and pelvic joint instability than normal women.
It is always wise to consider “neurovascular–lymphatic abnormality” in a patient with fascial disorder (e.g., regional fibrosis). There are many forms of free and encapsulated nerve endings found within the fascial layers that play different roles as “mechanoreceptors,” such as:
- (a)
Golgi receptors (type Ib receptors) are located at the myotendinous junction, only responsive to muscular contraction, and have a role in striated muscles motor tonus control (via inhibiting α–motor neurons).
- (b)
Pacini corpuscle (type II receptors) are located at the myotendinous junction, responsive to “rapid” pressure change and vibration, and have a role in propioceptive feedback of the joints.
- (c)
Ruffini corpuscle (type II receptors) are located in the ligaments and dura matter, responsive to “sustained” pressure, and have a role in “sympathetic activity inhibition” of the joints.
- (d)
Interstitial receptors (type III, IV receptors) are located everywhere in the fascial layers (most abundant receptors), responsive to “sustained and rapid” pressure change, and have a role in vasodilation and plasma extravastion. These receptors are 50 % low threshold units (A-δ fibers) and 50 % high threshold units (C-fibers).
Further Reading
Akeson W, et al. The connective tissue response to immobility: biochemical changes in periarticular connective tissue of the immobilized rabbit knee. Clin Orthop. 1973;93:356–61.
Cummings M, et al. Regional myofascial pain: diagnosis and management. Best Pract Res Clin Rheumatol. 2007;21(2):367–87.
Dormus M, et al. The effects of single-dose dexamethasone on wound healing in rats. Anesth Analg. 2003;97:1377–80.
Elder CL, et al. A cyclooxygenase-2 inhibitor impairs ligament healing in the rat. Am J Sports Med. 2001;29(6):801–5.
Hedley G. Notes on visceral adhesions as fascial pathology. J Bodyw Mov Ther. 2010;14:255–61.
Iwahashi M, et al. Mechanism of intervertebral disc degeneration caused by nicotine in rabbits to explicate intervertebral disc disorders caused by smoking. Spine. 2002;27(13):1396–401.
Murnaghan M, et al. Nonsteroidal anti-inflammatory drug-induced fracture non-union: an inhibition of angiogenesis? J Bone Joint Surg Am. 2006;88:140–7.
Myers TW. The “anatomy trains”, part 2. J Bodyw Mov Ther. 1997a;1(3):135–45.
Myers TW. The “anatomy trains”. J Bodyw Mov Ther. 1997b;1(2):91–101.
Schleip R. Fascial plasticity – a new neurobiological explanation: Part 1. J Bodyw Mov Ther. 2003a;7(1):11–1.
Schleip R. Fascial plasticity – a new neurobiological explanation: part 2. J Bodyw Mov Ther. 2003b;7(2):104–16.
Tozzi P, et al. Fascial release effects on patients with non-specific cervical or lumbar pain. J Bodyw Mov Ther. 2011;15:405–16.
Tozzi P. Selected fascial aspects of osteopathic practice. J Bodyw Mov Ther. 2012a;16:503–19.
Tozzi P. Selected fascial aspects of osteopathic practice. J Bodyw Mov Ther. 2012b;16:503–19.
13.2 Vertebral Malalignment (Subluxation) Syndromes
Malalignment syndrome, also known as “vertebral subluxation complex” (VSC), is a disorder characterized complex pathological processes that can be summarized as the following:
- 1.
Vertebral joint articulation disturbance which can be due to ligamentous laxity, posttraumatic event, or due to degenerative changes (spondyloarthropathy). Vertebral malalignment commonly results in rotation of one vertebra over the other initially (Fig. 13.2.1), which will progress if not corrected to retro- or anterolisthesis due to bilateral pedicular fracture (spondylosis).
Fig. 13.2.1
An illustration that demonstrates the rotational malalignment on axial and coronal planes
- 2.
Distortion of the pelvic ring associated with compensatory scoliosis of the lumbar vertebral column, which in turn causes compensatory distortion of the upper thoracic and cervical column. Rotational pelvic malalignment is detected in 80 % of cases, where the right or left pelvic bone rotates forward and the other usually compensates by rotating backward relative to the sacroiliac/coccygeal bone (Fig. 13.2.2).
Fig. 13.2.2
An illustration that demonstrates the complications of vertebral malalignment syndrome and its mechanical–pathological consequences
- 3.
Compensatory changes in the soft-tissue structures resulting in uneven back muscles contraction, which predispose to chronic back pain and myofascial trigger points formation.
- 4.
A long-standing vertebral malalignment causes intervertebral disk herniation disorder due to torsion of the annulus in a clockwise direction as a result of rotatory movement of one vertebra over the other (Fig. 13.2.3).
Fig. 13.2.3
An illustration that demonstrates the effect of the vertebral malalignment over the intervertebral disks; in a perfect alignment (a), the pressure overload is equally distributed over the intervertebral disk volume. In contrast, a malaligned vertebra (b) will exert uneven pressure load over the intervertebral disk predisposing it for herniation or protrusion
- 5.
Uncommonly, vertebral malalignment can cause regional and distant somatovisceral dysfunction attributed to:
- (a)
Spinal kinesiopathology: the spinal mechanics and motion irregularities resulted from pelvic obliquity can affect the genitourinary structures, for example.
- (b)
Neuropathy: it is due to impingement or stretching of the nearby neural tissues.
- (c)
Myopathy: it is due to myofascial trigger points formation in the regional muscular structures (see myofascial pain syndromes topic).
- (d)
Inflammation: fascial tension that arises from rotatory vertebral malalignment impedes the interstitial and neurovascular–lymphatic flows, causing regional wastes accumulation, hypoxia, local inflammation, and edema.
- (e)
Patho-anatomical changes: when all the past four components persists, local tissue degeneration occurs.
- (a)
Anatomy
The vertebral column vertebrae are composed of two main segments: the vertebral body and the posterior arch. The posterior arch is the portion that extends posteriorly from the vertebral body and helps to formulate the vertebral foramen.
Each vertebra is articulated with each other by two joints: (1) fibrocartilaginous joint that lies in between the vertebral bodies (contains intervertebral disk) and (2) a synovial facet joint that exists between the vertebral arches. The fibrocartilaginous disk space dissipates forces that are transmitted along the vertebral column.
The vertebrae along the vertebral column are held in position via multiple ligamentous structures that include:
- 1.
Anterior and posterior longitudinal ligaments: they run in an uninterrupted fashion along the anterior and posterior surfaces of the vertebral body, and they are highly innervated structures.
- 2.
Ligamentum flavum: it attaches the vertebral laminae together.
- 3.
Interspinous ligament: it attaches the superior and inferior portions of the spinous processes together.
- 4.
Supraspinous ligament: it attaches the tips of the spinous processes together.
- 5.
Intertransverse ligament: it attaches the transverse processes together.
A normal axial skeleton alignment is visualized in radiography as properly aligned pelvis, leg length is equal, and the spinouts processes are relatively straight, detected by a straight line that passes through all the spinous processes in anteroposterior radiograph (Fig. 13.2.4). Clinically, a normally aligned spine is characterized by equal length and tension in matching pairs of ligaments, muscles, and other soft tissues on the right and left sides of the human back. The past “ideal” description of the back is not applied, unfortunately, to many people, with up to 80 % of people “out of alignment” or “malaligned” by teens.
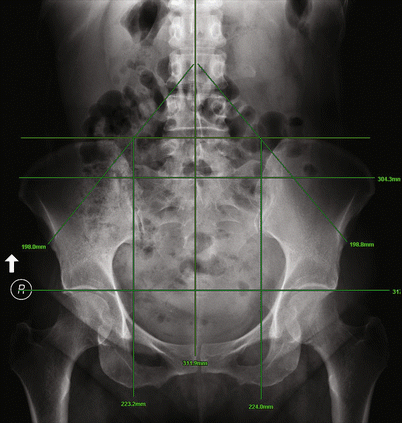
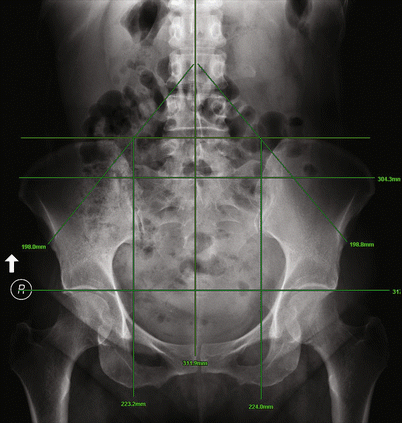
Fig. 13.2.4
An anteroposterior plain radiograph that demonstrates perfect alignment of the vertebral column in relation to the pelvis
According to the chiropractic and osteopathic medical literature, up to 97 % of causes of lower back and leg pain are “mechanical” in origin, and only 23 % are due to degenerative changes such as spondyloarthropathies and herniated disk disease. In up to 2 % of cases, lower back pain can be due to visceral disease (e.g., pancreatitis, aortic dissection, etc.).
Somatovisceral Malalignment Symptoms
As mentioned earlier, the vertebral malalignment syndrome can cause somatovisceral symptoms due to irritation of the spinal nerves exiting the neural foramina either by direct impingement or by the regional inflammation it produces. Many somatovisceral symptoms are known in the chiropractic and osteopathic medical literature to be directly or indirectly related to vertebral malalignment of a specific spinal segment because of the autonomic innervation emitted from that segment to visceral organs. Some of the common vertebral-origin symptoms include:
C1 (pituitary, scalp, brain vessels innervation): headache, tinnitus, insomnia, hypertension, and vertigo
C2 (optic, auditory, sinuses, mastoid, tongue innervation): chronic sinusitis, pain around the eyes, and hearing loss
C3 (facial skin innervation): occipital neuralgia (headache) and eczema (skin neurons irritation)
C4 (Eustachian tube innervation): hay fever, hearing loss, and otitis media
C5 (vocal cords innervation): hoarseness, laryngitis, and sore throat
C6 (neck muscles innervation): neck pain, shoulder pain, and chronic cough
C7 (thyroid, shoulder bursa innervation): thyroid conditions and shoulder bursitis
T1 (esophagus, trachea, upper limbs innervation): asthma, cough, apneas, and upper limb radiculopathy
T2 (coronary arteries innervation): functional heart diseases
T3 (lung, bronchial tree, heart innervation): bronchitis, pulmonary congestion, and pleurisy
T4 (GB, heart innervation): jaundice, GB disorders, and cardiac angina (T3–T7)
T5 (solar plexus, liver innervation): hypertension, arthritis, and liver disease
T6 (stomach innervation): gastroesophageal reflux disease, functional dyspepsia, and indigestion
T7 (pancreas, duodenum innervation): peptic ulcer and gastritis
T8 (spleen innervation): lowered immunity
T9 (adrenal innervation): allergies and hives
T10 (kidneys innervation): fatigue, nephritis, and renal arteriosclerosis
T11 (kidneys, ureters, skin innervation): eczema, boils, and acne
L1 (colon, inguinal rings innervation): colitis, diarrhea, constipation, and hernias
L2 (abdomen, thighs innervation): cramps, difficulty in breathing, and minor varicose veins
L3 (genitalia, ureters, bladder, knees innervation): bladder disorders, painful menstruation, miscarriages, impotency, and knee trigger points
L4 (prostate, perineum, sciatic innervation): sciatica, back pain, and dysuria
L5 (lower legs, feet innervation): leg pain/cramps, cold feet, and weak ankles
Sacrum: buttock pain, back pain, and sacroiliitis
Coccyx: coccydynia and pudendal neuralgia
13.3 Imaging Signs
- 1.
On plain radiographs, a normal vertebral alignment is detected by drawing a straight line overlying the spinous processes in anteroposterior (AP) radiographs of the cervical, thoracic, or lumbar vertebral column. Malalignment is simply detected as deviation of one or more spinous processes from the straight line alignment (Figs. 13.2.5 and 13.2.6). The more the rotational malalignment, the more scoliosis or kyphosis detected in the radiograph.
Fig. 13.2.5
Sequential anteroposterior plain radiographs of the lumbar vertebral column of different patients demonstrating the progression of malalignment syndrome from normal (left side) to the extreme form of compensatory scoliosis (right side)
Fig. 13.2.6
An example of anteroposterior plain radiograph of the neck in a patient presented with neck pain and posterior cranial headache; the plain radiograph shows uneven alignment of the cervical vertebrae detected by a line that joins between the spinous processes
- 2.
On MRI, vertebral malalignment can be detected indirectly via observing the spinous process axis along the scan area on axial images. The spinous processes will show different axes in each vertebral segment on axial images (Fig. 13.2.7).
Fig. 13.2.7
An example of MR images that indirectly show rotational malalignment in a patient presented for MR imaging to investigate lower back pain. The spinous process axis is deviated at the level of L4 vertebra (left image) and to a lesser degree at the level of L2 image (right image)
Further Reading
Bolton PS. Reflex effects of vertebral sublaxations: the peripheral nervous system. An update. J Manipulative Physiol Ther. 2000;23(2):101–3.
Good C. The sublaxation syndrome: a condition whose time has come? J Chiropr Humanit. 2004;11:38–43.
Harris JH. Malalignment: signs and significance. Eur J Radiol. 2002;42:92–9.
Husson JL, et al. The lumbar-pelvic-femoral complex: applications in hip pathology. Orthop Traumatol Surg Res. 2010;96S:S10–6.
Ratliff J, et al. Increased MRI signal intensity in association with myelopathy and cervical instability: case report and review of the literature. Surg Neurol. 2000;53:81–3.
Vernon H. Historical overview and update on sublaxation theories. J Chiropr Humanit. 2010;17(1):22–32.
13.4 Facet Joint Syndrome
Facet joint syndrome is a term used to describe pain originating from the facet joint, typically due to subluxation, nerve irritation, local inflammation, or degenerative changes and frictional spondyloarthritis.
Basic Anatomy
Each spinal segment consists of a “three-joint complex” formed by an intervertebral disk with small, posterior, paired synovial facet joints. A disturbance in one element affects the other two elements of this triple joint complex. The facet joint is made of a superior articular process derived from an inferior vertebra and faces dorsomedially, while the inferior articular process is derived from a superior vertebra and faces ventrolaterally. The facet joints are locked together during an attempted axial rotation of the spine. Also, the facet joint capsule has a role also in limiting excessive joint motion.
The healthy intervertebral disk dissipates its axial load uniformly across its endplates under compression and eccentric–compressive (e.g., compression and bending) loading conditions. Disk degeneration disturbs the “three-joint complex” allowing excessive facet joint motion to occur, which in turn assists in facet joint degeneration. The facet joint and the paraspinal muscles are supplied by proprioceptive nerve endings that help in analyzing the mechanical state of the facet joint each second by the central nervous system (position, tension, pressure, etc.).
Pathophysiology
Spinal motions are governed by the orientation of the facet joints and the intervertebral disks, costal cage, and muscular and ligamentous attachments. The superior articular facets in the cervical, thoracic, and lumbar spine vary in their orientation, for example:
- (a)
Cervical vertebrae (C1–C7): the facets face posteriorly and superiorly at a 45° angle.
- (b)
Thoracic vertebrae (T1–T12): the facets face posteriorly and laterally in the vertical plane.
- (c)
Lumbar vertebrae (L1–L5): the facets face medially at 45° to the sagittal plane.
Asymmetry of facet orientation, also known as “facet tropism,” can play a significant role in the production of faulty postural mechanics during activities of daily living, causing back and neck pain.
13.5 Imaging Signs
- 1.
Wrap-around bumper sign: this is a sign described in CT, as a degenerated facet joint will show sclerosis and form an osteophyte along the capsular attachment of the facet joint, as an attempt to stabilize the degenerated joint (Fig. 13.3.1).
Fig. 13.3.1
Multiple computed tomography (CT) images with 3D-reconstruction image that demonstrates the “wrap-around bumper sign” detected as halolike sclerosis wrapping around the left facet joint of L5/S1 vertebrae (arrows), with inner vacuum phenomenon detected as gas density in between the left facet joint space
- 2.
Facet joint angle: this is calculated by drawing a straight line along the posterior aspect of the disk space at a given spinal level and another two lines bisecting each facet joint. A facet joint <77.9° has a double risk of developing degenerative spondylolisthesis compared with patients who have facet joint angle >77.9°. This angle is mostly described in thoracolumbar facet joints (Fig. 13.3.2).
Fig. 13.3.2
An axial MR image at the level of L4 vertebra demonstrates the measurements of the “facet joint angle” bilaterally
- 3.
CT signs (Pathria’s classification): facet joint arthropathy is graded on CT as grade 0 (normal), grade 1 (joint narrowing), grade 2 (sclerosis and hypertrophy), and grade 3 (severe sclerosis and osteophyte formation).
- 4.
Facet joint effusion (MRI): it is normal to find 1–2 ml synovial fluid in facet joints on T2-weighted MT images, especially at the levels of L4–L5 vertebrae. A significant facet joint effusion is a sign of “spinal segmental instability” in up to 82 % of facet joint syndrome cases.
- 5.
On radiography, the facet joint shows reduced joint space and sclerosis of the facet joint, typically detected on lateral radiographs (Fig. 13.3.3).
Fig. 13.3.3
Lateral plain radiograph of a patient with neck pain shows facet joint spondyloarthropathy at the level of C3/C4 vertebrae (arrow)
Further Reading
McLain RF. Mechanoreceptor endings in human cervical facet joints. Spine. 1994;19(5):495–501.
Silbergleit R, et al. Imaging-guided injection techniques with fluoroscopy and CT for spinal pain management. RadioGraphics. 2001;21:927–42.
Stone JA, et al. Treatment of facet and sacroiliac joint arthropathy: steroid injections and radiofrequency ablation. Tech Vasc Intervent Rad. 2009;12:22–32.
Varlotta GP, et al. The lumbar facet joint: a review of current knowledge: part 1: anatomy, biomechanics, and grading. Skeletal Radiol. 2011;40:13–23.
13.6 Myofascial Pain Syndrome
Myofascial pain syndrome is a term used to describe pain and maybe concomitant visceral dysfunction due to the presence of a “tight fascial–muscular band” within a muscle or muscle group that acts like a pain “trigger point,” causing referred pain in another area. The area affected by this trigger point will show pain, decreased range of movement, muscular weakness, and often accompanied autonomic phenomenon. Predisposing factors for myofascial pain syndrome include:
- 1.
Leg length discrepancy
- 2.
Postural dysfunction and abnormalities (e.g., malalignment, scoliosis)
- 3.
Endocrine disorders (e.g., hypothyroidism)
Anatomy
To understand the muscle trigger point effect, it is crucial to understand the effect of fascia on the central nervous system. Stimulation of the fascial mechanoreceptors (Ruffini/Pacini corpuscles), like in tissue manipulation therapy, exerts effect on cortical system via the “proprioception pathway” transmitted via the spinal dorsal column–medial lemniscus system. This spinal effect will evoke an efferent response on skeletal muscles, causing change in their motor units tone. Stimulation of the fascial mechanoreceptors (A-δ and C-fibers), like in tissue manipulation therapy, exerts effect also on the autonomic nervous system. This autonomic effect will cause changes in local fascial capillary dynamics, intrafascial smooth muscles relaxation, and change in global muscle tone via hypothalamic tuning. The hypothalamus is tuned by the autonomic nervous system after stimulation of the fascial mechanoreceptors (A-δ and C-fibers), which will rust in change in global skeletal muscles tone. This effect seen in tissue manipulation therapy or deep tissue massage for skeletal muscles is also true for trigger points effect on the central nervous system. The former is a therapeutic effect, while the latter is a pathologic effect.
Another example of myofascial–nervous system interaction is seen in a technique known as “deep visceral massage,” which targets tissue manipulation of the visceral fascia, which in turn stimulates the mechanoreceptors of the enteric system. Many of the sensory neurons of the enteric nervous system are mechanoreceptors, which – if activated – trigger important neuroendocrine changes. These include a change in the production of serotonin (an important cortical neurotransmitter, 90 % of which is created in the intestine) and histamine (which increases inflammatory processes).
The superficial fascia is perforated in multiple regions in the body by a triad of vein, artery, and nerve (unmyelinated autonomic nerves). Heine, a German researcher who has been involved in the study of acupuncture and other complimentary health disciplines, found that the majority (82 %) of these perforation points are topographically identical with the 361 classical acupuncture points in traditional Chinese acupuncture, another proof of the myofascial–nervous system interaction.
A very interesting observation in the latest fascial physiology research correlates with the traditional Chinese medicine philosophy regarding the “Qi” energy. In Chinese medicine, Qi refers to movement or activity, not just any movement but the “proper” movement or activity of anything (e.g., human anatomy biomechanics). In Chinese medicine definition, Qi is the source of all movement in the body, protects the body, is connected to harmonious transformation (metabolism), retains the body’s substances and organs, and warms the body. The “Qi” definition in Chinese medicine is the same definition of “fascia” in Western medicine, so we could say that fascia is the same as Qi. Moreover, the “meridian channels” that connect the Qi points in acupuncture in the meridian maps are almost the same paths of the “fascial planes” in anatomical illustrations.
Pathophysiology
Fascia is an “electrical tissue” and considered the “largest sensory organ” in the body; also, it plays an important role in musculoskeletal biomechanics, peripheral motor coordination, proprioception, regulation of posture, and as a potential pain generator. Fascial restrictions can create abnormal strain patterns that can crowd or pull the osseous structures out of proper alignment, resulting in compression of joints producing pain and/or dysfunction (e.g., enthesitis). Moreover, neural and vascular structures can also become entrapped in these restrictions, causing neurologic symptoms, entrapment syndromes, veno-lymphatic stagnation and edema, or ischemic conditions.
The autonomic nervous system does not directly innervate the parenchymal cells, but it exerts its effect on the cells via mediating the chemical within the interstitial media (the extracellular fluid). If an injury is applied to the interstitial connective tissue (e.g., fascia), functional disturbance to the parenchymal cells homeostasis occurs and disease arises. For example, if a trauma is applied to the nerve fascia (e.g., the epineurium and perineurium), neurogenic inflammation arises which evoke nociception and possibly nerve trunk pain. Due to the bioelectric information capacity of the extracellular matrix (fascia), any situation that alters the electrical tone of the fascia can spread and be processed through the entire region, a potential by-product of cellular shock.
A myofascial trigger point pathophysiology can be summarized as the following:
- 1.
Restricted movement: taut muscle bands lead to shortening of the muscles, which in turn leads to reduced mobility and articular dysfunction. Depending on the muscle involved, different symptoms can arise.
- 2.
Vascular perfusion abnormality: if the taut bands compress the intra- or extra-muscular blood vessels, this leads to tissues ischemia, formation of edema, and trophic/metabolic changes (e.g., lactic acidosis within the muscle).
- 3.
Neuromuscular entrapment: fascia contains many nerve fibers embedded within fascial tunnels (e.g., medial superior cluneal nerve tunnel). A tense muscle and fascial fibers can exert pressure over the surrounding nerve fibers, resulting in entrapment syndromes and variety of neurological symptoms.
- 4.
Irritation of deep propioceptive and nociceptive nerve endings: connective tissue dysfunction alters the flow of impulses which come from the receptors which lie in the connective tissue of the muscle.
- 5.
Metabolic abnormalities: fascial tension can disturb circulation in the interstitium, disturbing the cellular environment.
- 6.
Breathing disorder: myofascial tension affects posture as well as abdominal and intrathoracic pressure.
To treat a myofascial trigger point, “myofascial release,” a hands-on therapeutic technique is used to treat tightened fascia that facilitates a stretch into the restricted fascia. It is performed by a sustained pressure that is applied into the restricted tissue barrier; after 90–120 s, the tissue will undergo histological length changes, allowing the first release to be felt. The goal of myofascial release is to elongate and soften the connective tissue, creating permanent three-dimensional length and width (e.g., change the ground substance from a sol to a gel).
Some Known Symptomatology of Myofascial Trigger Points Origin
Breast pain (mastalgia): pectoralis major and minor muscles.
Headache: temporalis, pterygoid, upper trapezius, and sternocleidomastoid muscles.
Lower back/SJ joint pain: pyramidalis, gluteus maximum, medius, and minimus muscles.
Inguinal pain: quadratus lumborum, Iliopsoas, and abdominal oblique muscles.
Pain during sitting: pyramidalis and obturator internus muscles.
Vulvar pain (vulvodynia): external anal sphincter.
Testicular pain (orchialgia): abdominal oblique, gluteus maximum, medius, and minimus muscles.
Penile pain: levator ani, bulbospongiosus, ischiospongiosus, and rectus abdominis muscles.
Prostate pain (prostatodynia): levator ani muscle.
Perianal pain: levator ani muscle.
Rectal pain (proctodynia): levator ani, coccygeus, and external anal sphincter muscles.
Sensation of a ball in the rectum: levator ani and obturator internus muscles.
Urethral syndrome: defined as urinary urgency, frequency, dysuria, and supravesical pain in the absence of any objective urological or laboratory findings. The disease arises due to spasm and myofascial triggers of the external urethral sphincter, obturator internus, and pyramidalis muscles (Fig. 13.4.1).
Fig. 13.4.1
A computed tomography (CT) image with 3D reconstruction in coronal plane that demonstrates the pyramidal muscle (arrows), a common cause of suprapubic pain due to myofascial trigger point
13.7 Imaging of a Case Study
A young 33-year-old female presented to our radiology department complaining of severe, intermittent, right-sided abdominal pain of the lower quadrant of stabbing nature with radiation downward to the thigh and upward to the breast. She had a history of abdominoplasty with a big scar in the abdomen that shows keloid changes (Fig. 13.4.2). At first, my initial impression was that she is complaining of postoperative abdominal adhesions, adding to the fact that she has history of three caesarian section deliveries.
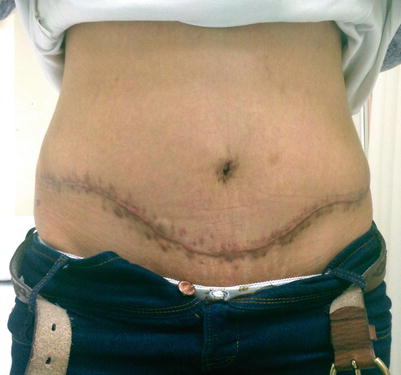
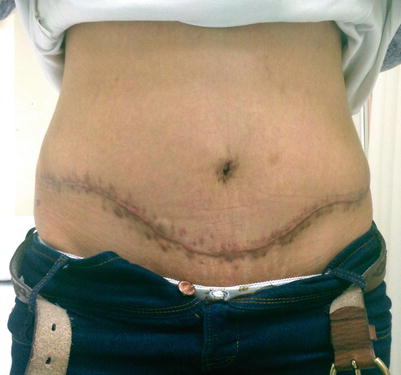
Fig. 13.4.2
A photograph of the patient’s abdomen that shows the abdominoplasty keloidal scar extension
Computed tomography (CT) for her abdomen and pelvis did not show any intestinal adhesions; however, it showed recti muscle diathesis and a superficial fatty stranding around the scar (Fig. 13.4.3a, b). MRI examination was done for her abdomen which showed enhancement of the scar at the region where anatomically the ilioinguinal and the iliohypogastric nerves are found (Fig. 13.4.4). The diagnosis of suspected “abdominal cutaneous nerve entrapment syndrome” was finalized in the report as a high suspicion diagnosis that needs surgical conformation. A surgical exploration was done for the patient, and very thick nerves were found. A biopsy of these nerves was sent to the pathology lab which came back positive for fibrotic, hypertrophied nerves.
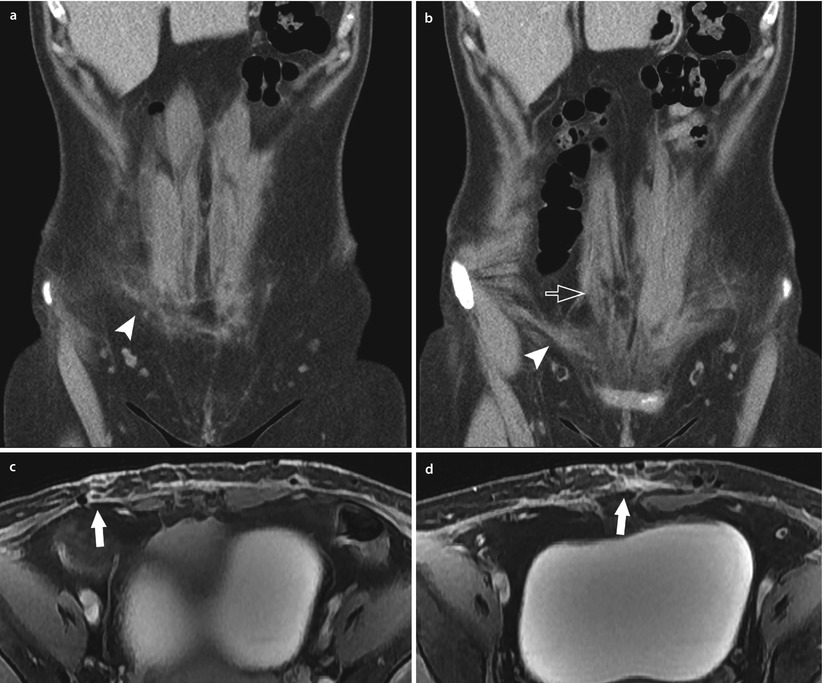
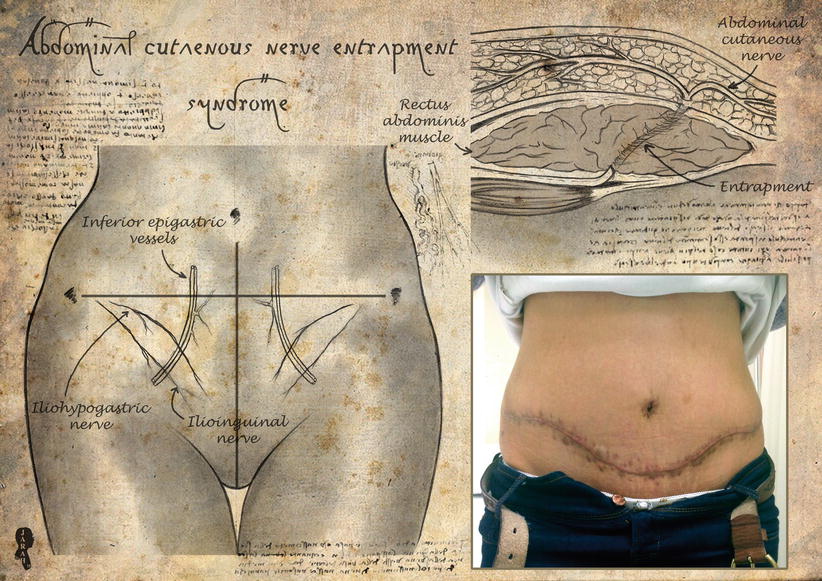
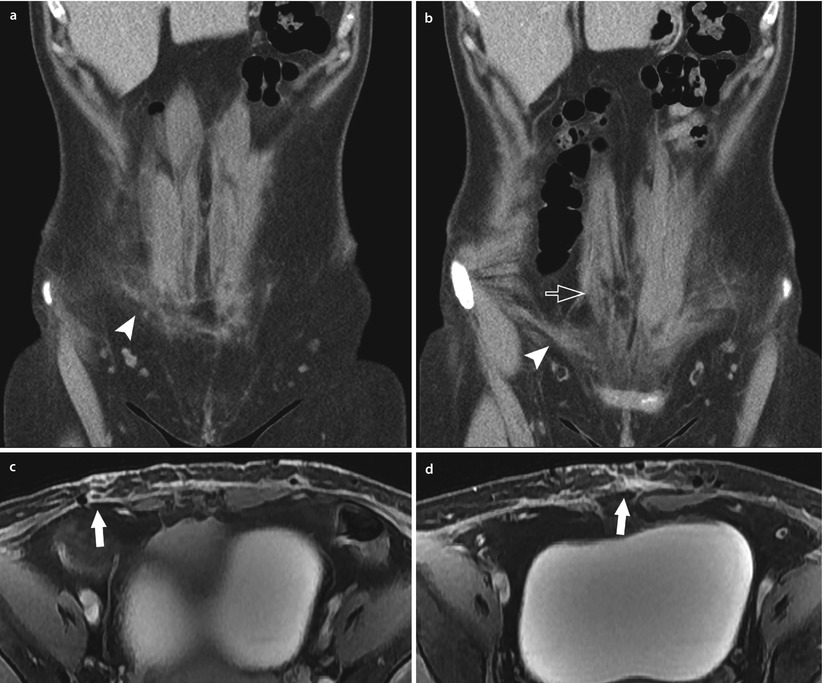
Fig. 13.4.3
Multiple CT images in coronal plane (a, b) and MR abdominal images in axial planes (c, d) of the patient; the myofascial scar is clearly seen as linear area of hyperdense fascia with fatty stranding (arrowheads in a, b). The right-sided rectal muscle show marked atrophy (empty arrowhead in b); on postcontrast MR images, the marked enhancement of the scar tissue is detected at the anatomical area of the ilioinguinal and the iliohypogastric neurovascular bundle; the diagnosis of suspected abdominal cutaneous nerve entrapment syndrome was made, which was confirmed by biopsy
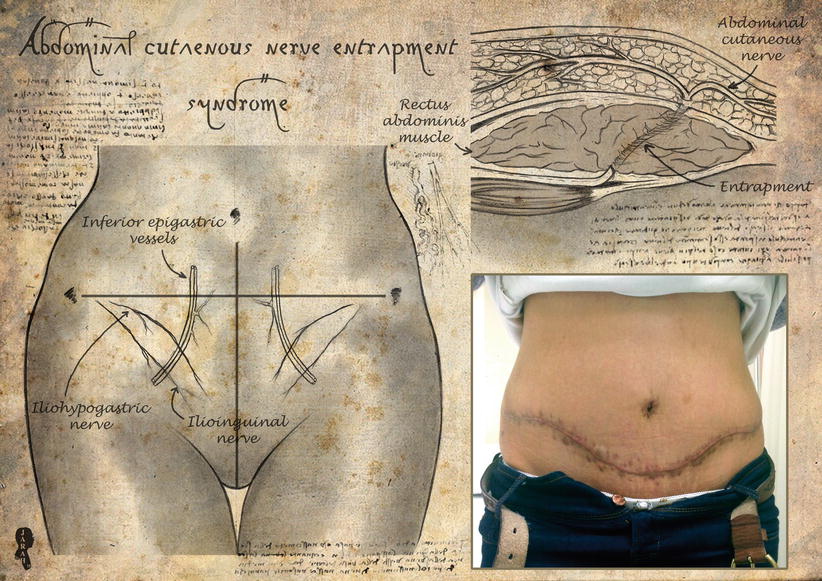
Fig. 13.4.4
An illustration that demonstrates the anatomical site of the ilioinguinal and the iliohypogastric nerves and the entrapment process
Further Reading
Barnes MF. The basic science of myofascial release: morphologic change in connective tissue. J Bodyw Mov Ther. 1997;1(4):231–8.
Chang S. The meridian system and mechanism of acupuncture – a comparative review. Part 1: the meridian system. Taiwan J Obstet Gynecol. 2012;51:506–14.
Chang S. The meridian system and mechanism of acupuncture – a comparative review. Part 2: mechanism of acupuncture analgesia. Taiwan J Obstet Gynecol. 2013;52:14–24.
Dorsher PT, et al. Acupuncture for chronic pain. Techn Reg Anesth Pain Manag. 2011;15:55–63.
Finando S, et al. Fascia and the mechanism of acupuncture. J Bodyw Mov Ther. 2011;15:168–76.
Itza F, et al. Myofascial pain syndrome in the pelvic floor: a common urological condition. Actas Urol Esp. 2010;34(4):318–26.
Ivens D, et al. Abdominal cutaneous nerve entrapment syndrome after blunt abdominal trauma in an 11-year-old girl. J Pediatr Surg. 2008;43:E19–21.
Lindsetmo RO, et al. Chronic abdominal wall pain-A diagnostic challenge for the surgeon. Am J Surg. 2009;198:129–34.
Manni L, et al. Neurotrophins and acupuncture. Auton Neurosci Basic Clin. 2010;157:9–17.
Montenegro MLLS, et al. Abdominal myofascial pain syndrome must be considered in the differential diagnosis of chronic pelvic pain. Euro J Obstet Gynecol Reprod Biol. 2009;147:21–4.
Rahn DD, et al. Anterior abdominal wall nerve and vessel anatomy: clinical implications for gynecologic surgery. Am J Obstet Gynecol. 2010;202:234.e1–5.
Raj PP, et al. Myofascial pain syndrome and its treatment in low back pain. Semin Pain Med. 2004;2:167–74.
Zhao ZQ. Neural mechanism underlying acupuncture analgesia. Prog Neurobiol. 2008;85:355–75.
13.8 Spinal Transitional Zone Syndromes
The spinal transitional zone (STZ) is defined as the vertebral zone located between two adjacent spinal vertebrae with various differences in their “posterior element” orientation and differing degrees of mobility. A “transitional lumbosacral vertebra” is defined as a vertebra that shows elongation of its transverse process with variable degrees of fusion to the “first” sacral (S1) segment.
Basic Anatomy
The spinal transitional zones (STZs) are prone to vertebral rotational malalignment (vertebral subluxation complex) with various subcutaneous fat, enthesis, and muscle (cellulo-teno-periosteo-myalgic) manifestations. Four vertebral transitional zones are described:
- 1.
Cervico-occipital (CO) junctional zone
- 2.
Cervicothoracic (CT) junctional zone
- 3.
Thoracolumbar junctional zone
- 4.
Lumbosacral (LS) junctional zone
Pathophysiology
Malalignment/subluxation of the vertebrae in the STZs can result in somatovisceral symptoms according to the myotome/dermatome involved. STZ syndromes, elegantly described by the French osteopath Robert Maigne, are characterized by a triad (not necessary all three present together) of:
- 1.
Cellulalgia: described clinically as painful, deep, burning-like subcutaneous tissue pain, swelling, and induration in all or part of the affected dermatome
- 2.
Myalgia: described clinically as painful, taut bands of muscle fibers – trigger points – localized in some muscles of the affected myotome
- 3.
Enthesitis: described clinically as hypersensitivity of the teno-periosteal insertions (entheses) of the affected sclerotome
According to Maigne and many other researchers in the osteopathic, chiropractic, and manipulative medicine field, four main STZs syndromes are recognized (Fig. 13.5.1):
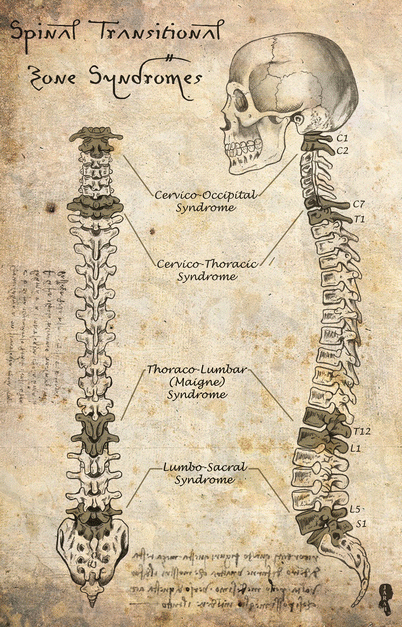
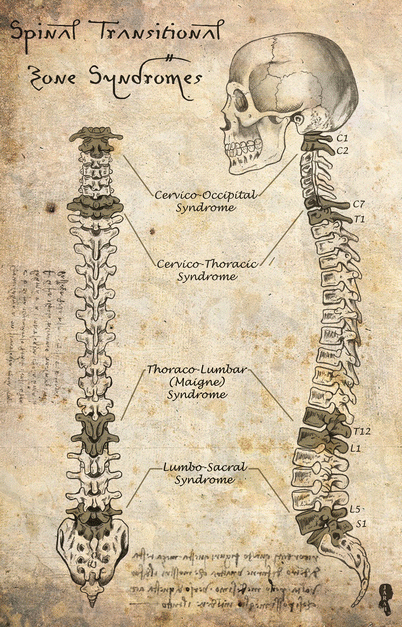
Fig. 13.5.1
An illustration that shows the four types of transitional zone syndromes according to Maigne and others
Cervico-Occipital Transitional Zone Syndrome
Cervico-occipital (CO) junction zone abnormality refers to the manifestations of vertebral subluxation complex that are detected mainly between the occipital skull base condyles and atlas (C1) vertebra. Beside C1 subluxation, other conditions that can cause CO junction zone abnormality include (a) atlanto-occipital assimilation (a condition characterized by fusion between the atlas and the skull base due to failure to segmentation of these two structures embryologically), (b) whiplash injury, (c) foramen magnum syndrome, (d) cervical torticollis, and (e) and basilar indentation (defined as displacement of the odontoid process into the foramen magnum).
Clinical manifestations of CO junction zone syndrome include:
- 1.
Cervicogenic headache is defined as a headache that is caused by a lesion within the cervical spine or in the soft tissues of the neck detected by clinical, laboratory, and/or imaging evidence. Cervicogenic headache is the commonest manifestation of the CO subluxation and manifested typically as severe pain experienced in the occipital, hemicranial (migraine-like), or supraorbital region.
- 2.
Trigeminal neuralgia arises typically due to indirect involvement of the “spinal trigeminal nucleus and tract.” The trigemino-cervical nucleus is a region of the upper three cervical spinal cord (C1–C3) where sensory nerve fibers in the descending tract of the trigeminal nerve (trigeminal nucleus caudalis) are believed to interact with sensory fibers from the upper cervical roots. These functional convergences of upper cervical and trigeminal sensory pathways allow the bidirectional referral of painful sensations between the neck and trigeminal sensory receptive fields of the face and head.
Cervicothoracic Transitional Zone Syndrome
Cervicothoracic (CT) junction zone syndrome refers to the manifestations of vertebral subluxation or facet joint abnormalities between C5–C6 and C6–C7 vertebrae. Clinical manifestations include one or all of the following features ipsilateral to the subluxation complex (Fig. 13.5.2):
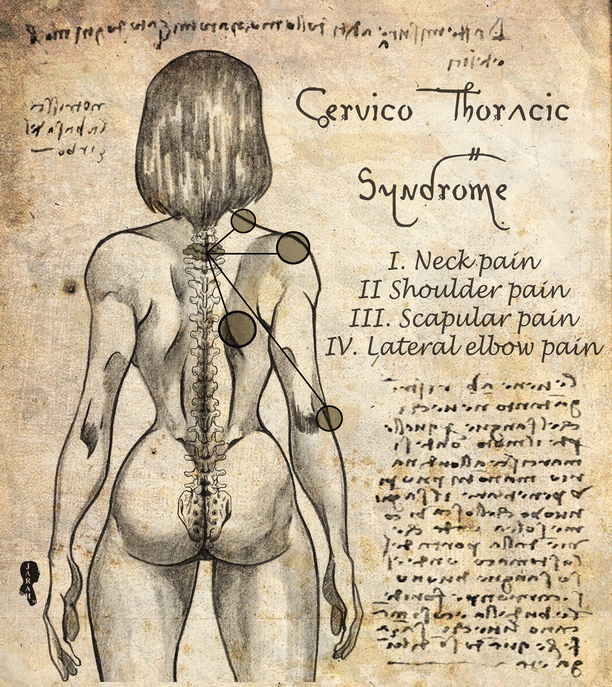
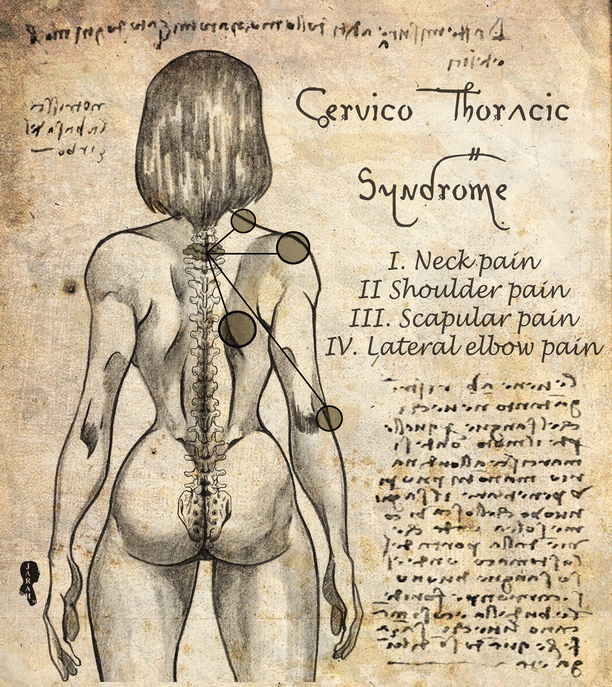
Fig. 13.5.2
An illustration that shows the four types of cervicothoracic transitional zone syndrome clinical pain distributions
- 1.
Neck pain: due to myofascial trigger points in the neck muscles
- 2.
Shoulder pain: due to involvement of the brachial plexus
- 3.
Interscapular pain: due to vertebral malalignment, functional scoliosis, and/or thoracolumbar fascia tightness
- 4.
Lateral elbow epicondylar pain: presents with tennis-elbow-like presentation (lateral epicondylitis)
Thoracolumbar Transitional Zone Syndrome
Thoracolumbar (TL) junction zone abnormality, also known as “Maigne syndrome,” “Dorsal ramus syndrome,” and “T12–L2 segmental vertebral cellulotenoperiosteomyalgic syndrome,” refers to the manifestations of vertebral subluxation or facet joint abnormalities between T12 and L2 vertebrae. Manifestations of Maigne syndrome include (Fig. 13.5.3):
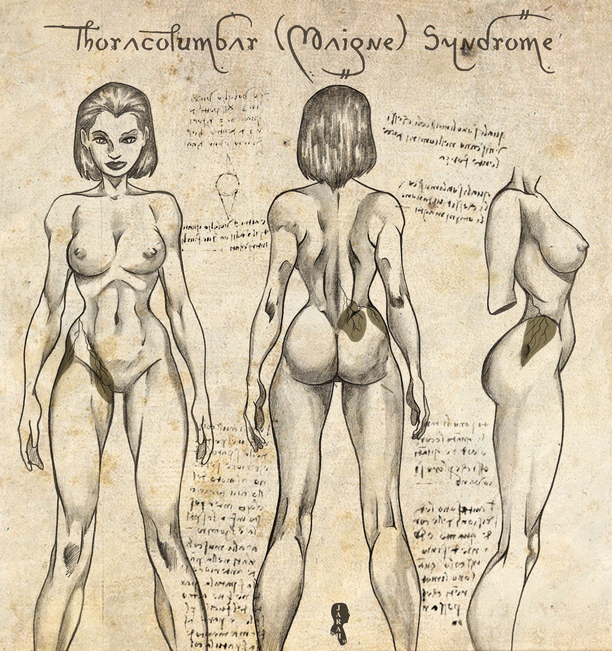
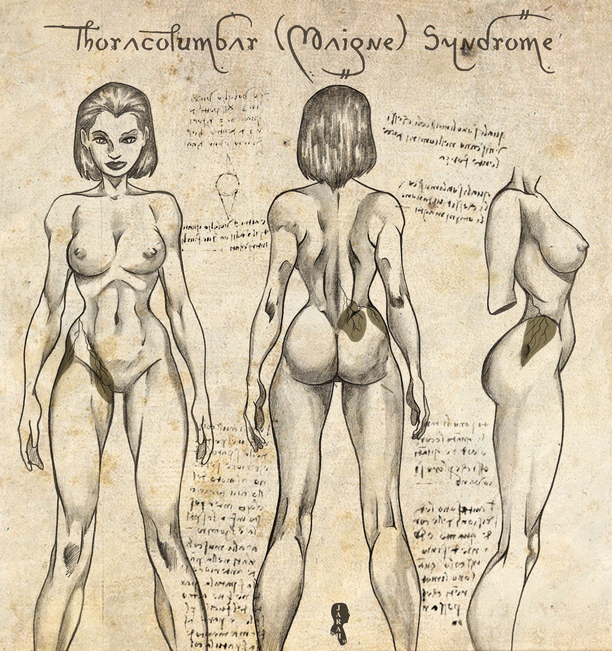
Fig. 13.5.3
An illustration that shows the three clinical pain distributions of Maigne syndrome
- (a)
Low back pain (97 %): it arises due to irritation/impingement of the “dorsal” spinal nerve root ramus of T12–L1 (Iliohypogastric nerve), which supplies the superior gluteal and inferior lumbar subcutaneous tissues. Trigger points can be found within the rectus abdominis and the quadratus lumborum muscles. Back pain is the most common manifestation of Maigne syndrome.
- (b)
Lower abdominal visceral pain (60 %): clinically can present with symptoms that mimic gynecological, testicular, renal colic, or irritable bowel syndrome such as bloating, constipation, and abdominal meteorism. These manifestations arise due to irritation/impingement of the “anterior” spinal nerve root ramus of T12–L1 (Iliohypogastric nerve). The iliohypogastric nerve is known to carry visceral sympathetic fibers to the pelvic organs.
- (c)
Hip pain (56 %): arises due to irritation/impingement of the “perforating lateral cutaneous branch” of T12–L1, which innervates the trochanteric region.
- (d)
Pubic pain (32 %): due to irritation of the symphysis pubis, often unilateral to the vertebral subluxation site.
13.9 Imaging Signs
- 1.
Most of radiographs in Maigne syndrome are normal; however, vertebral subluxation, degenerative changes, facet joints hypertrophy, or disk protrusion can be seen affecting the level of T12–L2 vertebrae, which can be diagnostic after excluding an organic cause of pain plus the classical distribution of symptoms (Fig. 13.5.4).
Fig. 13.5.4
Plain radiograph (a & b) of a patient who presented with right-sided lower back pain and inguinal pain; the radiograph shows slight, right-sided vertebral malalignment at the level of T12-L1 vertebrae (arrow in b)
- 2.
Atrophy and fatty degeneration of the paraspinal muscles with lack of other vertebral column pathology (e.g., normal vertebral disks MRI) is highly suggestive of Maigne syndrome because the main pathology is irritation of the dorsal spinal nerve ramus in the first place, which cannot be visualized in MRI directly yet.
- 3.
In CT, Maigne syndrome can be suggested by a lesion affecting the intervertebral foramen, where the dorsal ramus exits. Other features on CT include facet joint spondylosis or hypertrophy, periarticular calcifications, and calcification of the ligamentum flavum at the level of T12–L2.
Lumbosacral Transitional Zone Syndrome
Lumbosacral transitional vertebra (LSTV) is a common finding in plain radiography of the general population reaching up to 20 %. Two main lumbosacral transitional vertebrae are described:
- (a)
Lumbarization of S1 vertebra: described when the first sacral (S1) vertebra shows short transverse processes with an intervertebral disk between S1 and S2 vertebrae, resulting in S1 vertebra that mimics the lumbar vertebrae. On imaging, the patient will show 6 lumbar vertebrae rather than 5 (Figs. 13.5.5 and 13.5.6).
Fig. 13.5.5
Plain radiograph of a patient who presented with chronic lower back pain; the plain abdominopelvic radiograph shows lumbarization of S1 vertebra
Fig. 13.5.6
MR images of the same patient in Fig. 13.5.5 shows clear, well-developed intervertebral disk that exists between S1 and S2 vertebrae due to lumbarization of S1 vertebra (arrowhead)
- (b)
Sacralization of L5 vertebra: described when the 5th lumbar (L5) vertebra shows short transverse processes with bilateral fusion of the L5 to the first (S1) sacral vertebra with no intervertebral disk in between the two vertebrae. On imaging, the patient will show 4 lumbar vertebrae rather than 5 (Fig. 13.5.7).
Fig. 13.5.7
Plain radiograph and 3D-CT reconstructed image of a patient who presented with chronic lower back pain; the plain abdominopelvic radiograph and the CT show sacralization of L5 vertebra
LSTV has been described with lower back pain since 1917 by Bertolotti, and it was referred to as “Bertolotti’s syndrome” (e.g., lower back pain due to sacralization/lumbarization of L5–S1 vertebrae). The mechanisms of such the pain formation can be due to:
- 1.
Abnormal L5–S1 biomechanics: LSTV predisposes to early L5–S1 spondyloarthropathy and intervertebral disk disease, causing uneven paraspinal muscle contraction and formation of trigger points within the paraspinal and sacral muscle.
- 2.
Far-out syndrome: described as a clinical condition where the L5 nerve is impinged “far-laterally” between the elongated transverse process of L5 and the ala of the sacrum. The condition was first described by Wiltse et al. (1984).
13.10 Imaging Signs
- 1.
Lumbosacral transitional vertebrae (LSTV) have been classified by Castellvi (1984) into five main subtypes based on imaging features:
LSTV type I: long, dysplastic transverse process >19 mm found unilaterally (Ia) or bilaterally (Ib) (Fig. 13.5.8)
Fig. 13.5.8
Pelvic plain radiograph that demonstrates bilateral lumbosacral transitional vertebrae (Castellvi type I; arrowheads)
LSTV type II: large, squared transverse process with false joint formation (pseudoarthrosis) between the transverse process and the sacrum ala unilaterally (IIa) or bilaterally (IIb), forming incomplete lumbarization/sacralization (Fig. 13.5.9)
Fig. 13.5.9
Multilevel coronal, pelvic CT images that demonstrate lumbosacral transitional vertebra with formation of pseudoarthrosis between the left-sided transverse process and the sacral ala (Castellvi type II; arrowhead)
LSTV type III: large, squared transverse process with complete fusion between the transverse process and the sacrum ala unilaterally (IIIa) or bilaterally (IIIb)
LSTV type IV: mixed type (e.g., type IIIa on one side and type IIa on the other side)
- 2.
Far-out syndrome can arise due to large osteophyte impinging on the L5 nerve, Castellvi type IIa LSTV, and impingement of the nerve inside a “lumbosacral tunnel” formed by the L5 vertebral body, the sacral ala, and the lumbosacral ligament.Stay updated, free articles. Join our Telegram channel
Full access? Get Clinical Tree
