Fig. 3.1
Sixty-one-year-old female with carotid stenosis. In TOF images proton dephasation occurs at the site of stenosis causing intraluminal loss of signal simulating focal occlusion. Contrast-enhanced images demonstrate lumen patency at~the site of stenosis, with severe reduction of lumen caliper
4.2 Imaging with 3D T1-Weighted Sequences After Injection of Contrast Medium
Contrast-enhanced MRA techniques are based on the capability of paramagnetic agents (Gd chelates) to reduce the T1 of flowing blood inside the vascular structures in comparison to that of stationary tissues; the main advantage of these techniques is to eliminate the dependence of signal intensity of blood from the blood flow velocity in the vessel (Fig. 3.2). The importance of this feature appears to be more important in small arteries such as carotids, which, if affected by stenosing lesions, may have significant reductions in the flow rate at the level of irregularity of the wall, simulating stenosis, or occlusion in the flow-dependent sequences. The technical and diagnostic success of these studies with contrast medium is based on the conjugation of three parameters: spatial resolution, temporal resolution, and synchronization between image acquisition and contrast agent administration [6]. The study is performed using 3D T1-weighted gradient-echo sequences with spoiler gradient, acquired with low values of TR and FA (in order to optimize the difference in signal intensity between blood and stationary tissue) and low values of TE to minimize T2* effects; the acquisition is performed before and after administration of contrast medium using subtraction techniques to remove the signal of the anatomical extravascular structures and increase image contrast. The setting of scanning parameters should be adjusted to the reduced size of the vascular segments in question, to the relatively large field of view (from aortic arch to the intracranial circulation), and especially to the rapid circulation time of the cervical vessels. Using state-of-the-art equipment, acquisition may be performed with semi-isotropic or isotropic voxel size (maximum 1 mm3) and a minimum of 384 × 384 matrix; acquisition time must be optimized for a duration between 14 and 18 s depending also on the flow speed of contrast medium administration. Synchronization between contrast medium administration and acquisition start is generally set by fluoro-MR bolus-tracking techniques, with multiple low spatial resolution scans acquired at the aortic arch in order to display in real time the arrival of the contrast agent [7]. Contrast administration rate affects both the duration and intensity of peak enhancement: at low flows the rate corresponds to reduced but lasting enhancement, while at high flows it corresponds to greater but shorter enhancement. The use of high relaxivity contrast agent allows low flow administrations with high and prolonged enhancement.
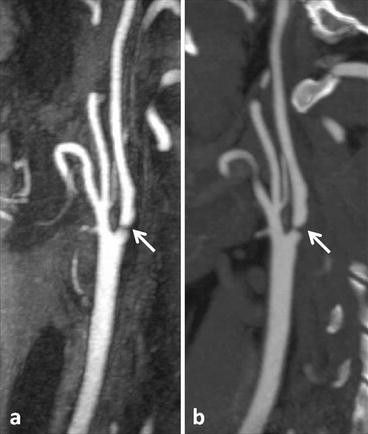
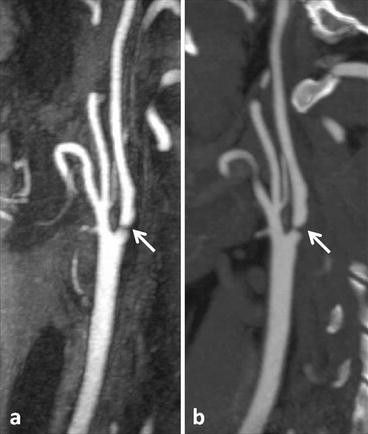
Fig. 3.2
(a) Contrast-enhanced MRA performed with 3D T1-weighted sequences after injection of contrast medium shows the presence of a tight stenosis at the base of the internal carotid artery (arrow). CTA findings (b) are superimposable to that of MRA (arrow)
It is very important to combine acquisition parameters and contrast injection protocols with adequate techniques of K-space sampling: the most effective approach is the elliptic-centric sampling [8], through which the central portion of the K-space (containing data on the image contrast resolution) is first sampled using the highest concentration of contrast medium into the vessel, while the peripheral portion (containing data on the spatial resolution) is sampled during more delayed acquisition, where the concentration of the contrast agent begins to decline leading to a progressive loss of signal and contrast resolution within the vascular structures. The integration of all these parameters has been simplified by the introduction of parallel imaging techniques and the use of integrated multichannel coils, whose combined use makes possible the acquisition of sequences with high spatial resolution in significantly reduced times. However, the technical progress in the field of MRA has not remained confined to the equipment and the development of new sequences: thanks to a wide experience in different experimental protocols developed during the past decade, the first contrast agent belonging to the family of compound blood pool has recently been approved and made available for trading. This innovative new class of contrast agents has been designed with the intent of combining profiles of R1 relaxivities higher than those of conventional compounds with specific binding properties to plasma proteins, making possible an extended stay within the vascular bed. These features allow to bring the adaptation of the acquisition protocols beyond the current limits imposed by first-pass imaging which is linked to the MRA (Fig. 3.3): taking advantage of the prolonged vascular enhancement reached during the equilibrium phase of contrast medium, 3D sequences with sub-millimeter voxel can be acquired virtually without time limits, obtaining a spatial resolution equal or even greater than the latest generation multislice CT [9]. Currently the spread of blood-pool compounds is limited and its clinical experience is reduced [10]; anyway, thanks to the potential increase in the quality and in diagnostic accuracy for vascular studies, it is desirable its wide diffusion. Recently, high-resolution sequences acquired during the equilibrium phase have been performed using an interstitial contrast agent (gadobenate dimeglumine) that differs from conventional gadolinium contrast agents because of its interaction with serum albumin. The consequent decreased T1 relaxation time and increased R1 relaxivity of gadobenate dimeglumine are sufficient to permit reliable acquisition of SS images of the carotid vasculature subsequent to routine FP image acquisition [11]. Finally, it cannot be neglected to mention the importance of 4D techniques, or time-resolved imaging, in MRA innovative applications and clinical indications: these techniques, known by commercial acronyms TRICKS (GE), TWIST (Siemens), 4D-TRACK (Philips), DRKS (Toshiba), combine the high spatial resolution of 3D datasets acquired with gradient-echo sequences and the ability to repeatedly examine the same vascular segment in very short time intervals, thus obtaining a dynamic view of contrast medium flowing within the vessels. In carotid MR imaging the application of 4D techniques is very useful in the evaluation of hemodynamic features in the vascular malformations, arteriovenous fistulas, and dissection imaging. Other interesting technical issues in MRA of the carotid arteries have been widely investigated, while remaining mostly confined to the clinical fields of research studies.
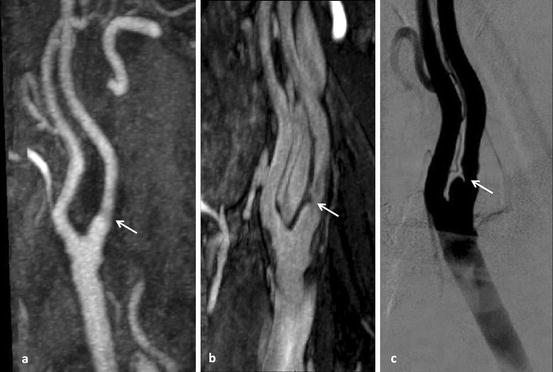
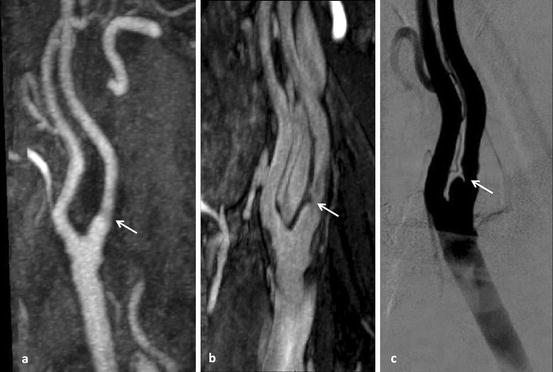
Fig. 3.3
(a) Contrast-enhanced MRA shows mild irregularity of the wall without significant stenosis (arrow); (b) high-resolution sequence acquired during the equilibrium phase shows ulceration of the wall with moderate stenosis (arrow); (c) DSA confirmed both ulceration of the wall and stenosis (arrow)
4.3 Imaging with Phase-Contrast Sequences
Phase-contrast sequences exploit blood protons speed of changing phase that move parallel to the static magnetic field. Through the activation of a pair of bipolar gradients that sequentially diphase and phase again the spins during the acquisition, it is possible to obtain images where pixels signal intensity directly corresponds to the moving speed values inside the anatomical segment in question: by changing the velocity encoding parameters (velocity encoding—VENC) it is possible to have a selective imaging of arterial (VENC factor > 40 cm/s) or venous (VENC factor <20 cm/s) vessels, without any signal derived from stationary tissues, whose protons remain static. In carotid imaging, the application of phase-contrast sequences has limited utility, mainly for long acquisition times and low immediacy in image interpretation in clinical and surgical fields; however, their application has been described in the evaluation of carotid artery stenosis [12] but also in the study of vascular malformations, aneurysms, and dissections, in which the morphological alteration of the vessel wall is frequently associated with changes of the blood flow velocity and flow direction.
4.4 Imaging of Atherosclerotic Plaque with High-Resolution Sequences
Some important histopathological studies have demonstrated a strong correlation between morphostructural changes in carotid atherosclerotic plaques and symptomatic cerebrovascular thromboembolic events [13]: the presence of irregularities of the plaque surface, ulcers, the prevalence of soft component instead of the calcified one, and hemorrhages inside the plaque were described as significant risk factors, independent from the degree of vascular stenosis in facilitating ischemic events (Fig. 3.4). The study of such alterations, also known as “vulnerable plaque”, was proposed and realized with MR technology [14]. The dedicated plaque imaging is not an angiographic study, but rather a morphological evaluation (T1, T2 also with superparamagnetic contrast medium) using very high spatial resolution sequences that in the future could join the conventional MRA: currently the clinical use of this approach is limited because of the technical requirements that are not available on a large scale (high field magnets, dedicated surface coils, and endovascular micro-coils) and of the long acquisition times, often not easily tolerated by patients clinical conditions.
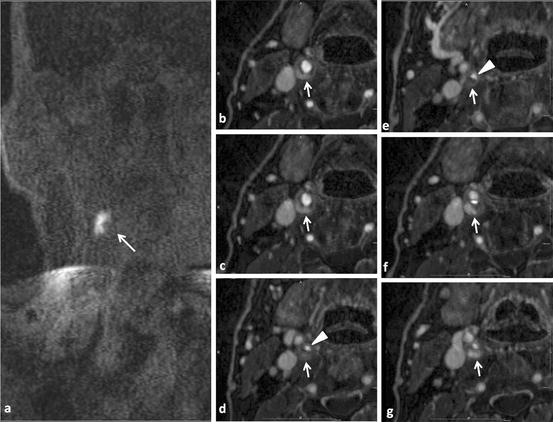
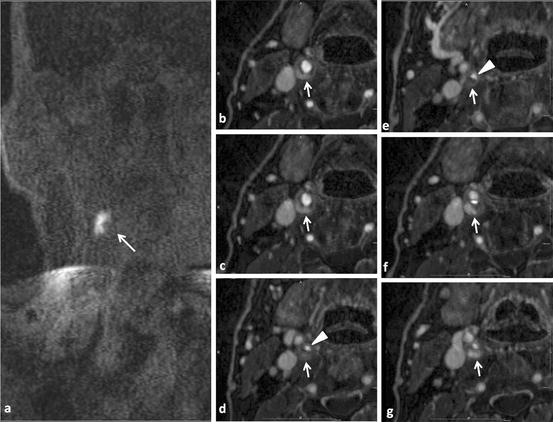
Fig. 3.4
(a) Pre-contrast T1-weighted images show the presence of a hyperintense area in the right cervical region (arrow); (b–g) high-resolution sequences acquired at the equilibrium phase demonstrate the presence of a severe stenosis of the right internal carotid artery (arrowheads in d and e); the enhancement of the soft component (arrows) demonstrates the presence of an hemorrhagic and inflammatory component (arrows)
5 Post-processing
Digital imaging, 3D datasets, and post-processing techniques have become routinely using tools in diagnostic radiology: almost all the methods (most of all CT and MRI) use these techniques to speed up and simplify radiologist work. In MRI post-processing techniques are very useful in vascular imaging, even with the recent development of acquisition protocols that allow obtaining images with isotropic voxels.
Compared to CT, the possibilities and perspectives of application in MRI seem small, but their importance remains high to reduce reporting times and provide immediate and easily interpretable images to clinicians and surgeons. The available approaches are generally divided into:
Section techniques (MPR, curved-MPR, thick-MPR, c-MPR): multiplanar reformatting techniques (MPR) use 3D datasets acquired on a single plane (i.e., coronal) to reproduce two-dimensional images on different orthogonal planes (i.e., axial or sagittal) or curved planes (curved-MPR, in which the entire vessel is “rolled out” on the longitudinal axis) (Fig. 3.5). Generally, the slice thickness obtained with this reconstruction technique does not exceed that of the native slices of the dataset; anyway it is possible to get thicker slices using a technique with partially projective characteristics (thick-MPR). In carotid imaging, section techniques are very important not much in atherosclerotic lesion identification or in panoramic assessment of vascular structures, but especially in small wall irregularities characterization and in the exact evaluation of stenosis degree, in order to have an accurate surgical planning [15].
Fig. 3.5
(a) Contrast-enhanced MRA shows a long plaque localized at carotid bifurcation causing severe stenosis at the origin of the internal carotid artery; (b) curved-MPR allows to roll out the entire vessel on its longitudinal axis and visualize the stenosis from different angles (arrow); (c) high-resolution sequence acquired during the equilibrium phase better demonstrates plaque morphology and stenosis degree (arrow)
Projective techniques (MIP and thin-MIP): MIP images are obtained by projecting all the voxels contained within the dataset having the higher signal intensity on the same plan (approximately 10% of the total information), excluding all others voxels. The optimal application of MIP images is obtained when the structure in question shows greater signal intensity compared to tissues in the background (MRA, MRCP). The main advantage of MIP techniques in carotid MRA is to provide panoramic views and rapid interpretation of the images (simultaneous display extending from aortic arch to the intracranial circulation), but losing fine details (i.e., small wall irregularities, small ulcers) that are masked by voxels with greater intensity.
For this type of evaluations the use of thin-MIP is preferable; similar to the thick-MPR, this technique projected on the same plane data obtained from a limited number of voxels (i.e., those contained in 5, 10, or 15 mm slice thickness) without hide background structures and allowing the evaluation of small changes in the vessel wall. Before the widespread use of software, 3D reconstruction console, and the advent of high-resolution acquisitions with isotropic or semi-isotropic voxels, MIP images were used to measure the degree of stenosis; currently this approach has been replaced by the evaluation with MPR and its variants, for greater accuracy in measurements [16].Stay updated, free articles. Join our Telegram channel
Full access? Get Clinical Tree