The prognosis for patients diagnosed with primary central nervous system tumors, such as gliomas, remains generally poor. Improved treatment (standard therapies and novel strategies) is needed. Glioma surgery is a key part of standard treatment and has established roles in providing tissue for diagnosis and in tumor debulking. Several techniques to increase safe surgical resection have been investigated. In addition, novel surgical modalities introducing therapeutic agents locally are increasingly common. This article reviews recent glioma surgery clinical trials, focusing on outcome studies, novel surgical techniques, and local therapeutic agent delivery.
Central nervous system tumors represent a continued medical and surgical challenge. Primary intra-axial brain tumors, more specifically gliomas, have an annual incidence of approximately 14 per 100,000. Glioblastoma is the most frequent and malignant histologic subtype (World Health Organization [WHO] grade IV). Surgery has an established role in the diagnosis and treatment of brain tumors and forms a key part of standard therapy along with radiation and chemotherapy. Outcomes for most brain tumor patients, however, remain humbling and continued efforts to improve and refine brain tumor treatment (including surgery) are needed. This article focuses on clinical trials in primary intra-axial brain tumor surgery, including a review of outcome data with standard surgical maneuvers, techniques to maximize safe surgical resection, and trials of surgically delivered therapeutics.
Standard neurosurgical techniques
Standard neurosurgical techniques in brain tumor management can be divided into those primarily directed at diagnosis (ie, techniques for biopsy) and those directed at tumor debulking (ie, techniques for craniotomy and resection). This section reviews clinical trials of these standard techniques, including outcome studies.
Frame-Based Stereotactic Biopsy
Stereotactic biopsy can be defined as biopsy of intracranial lesions through a burr hole using stereotactic target coordinates determined on prebiopsy imaging. Stereotactic biopsy techniques were first developed using rigid frames attached to a patient’s skull. A rigid fiducial marker box is attached to the frame base before imaging (CT or MR imaging), thus defining the stereotactic space to determine the target, entry point, depth, and trajectory based on X, Y, and Z coordinates and a Cartesian system. The fiducial marker box is replaced by a stereotactic guide system attached to the frame that directs a biopsy needle from the entry point to the target along the selected trajectory to the depth calculated by the system ( Fig. 1 ). Frame-based stereotactic needle biopsy can be performed with local or general anesthetic. The technique is generally safe and has a high chance of obtaining diagnostic material. Diagnostic yields are 91% to 98.4% with transient morbidity of 2.9% to 9%, permanent morbidity of 1.5% to 5%, and mortality of approximately 1% in modern series. Small, deep lesions in eloquent brain are associated with higher rates of morbidity and nondiagnosis. In addition, the number of separate trajectories needed to obtain diagnostic material has been associated with increased morbidity. Finally, there remains the potential for sampling error with stereotactic biopsy. In a series of patients at the MD Anderson Cancer Center who underwent craniotomy and tumor resection after previously undergoing stereotactic biopsy for glioma, the final pathologic diagnosis changed in 38% once further tissue was available. This was largely accounted for by differences in tumor grade.
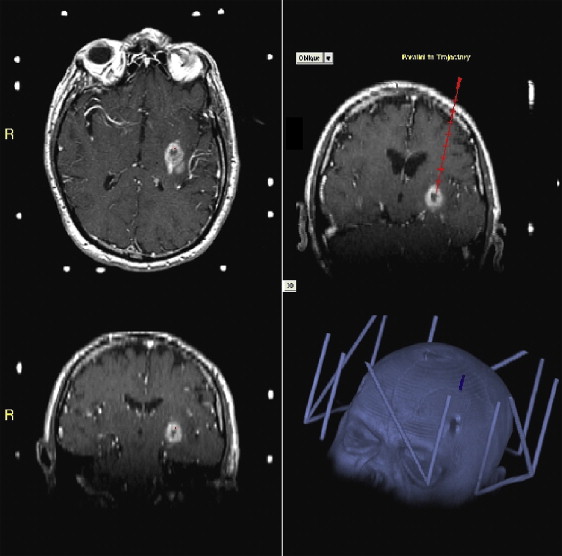
Frameless Stereotactic Needle Biopsy
Frameless stereotactic biopsy performed using an intraoperative neuronavigation system, such as Stealth, BrainLab, or ISG Wand, is increasingly common. Preoperative imaging (MR imaging or CT) is obtained after placing multiple adhesive fiducial markers on the patient’s scalp. This information is transferred to an intraoperative computer connected to a binocular sensing device (eg, infrared camera) that can register the preoperative imaging in stereotactic space using the fiducial markers ( Fig. 2 ). Alternatively, some systems register preoperative imaging using bony landmarks. Once the imaging and patient are registered, a viewing wand or other tool can be used to demonstrate the underlying anatomy in 3-D. An entry point, trajectory, depth, and target can then be determined for the biopsy. A guide system attached to the skull or head holder keeps the biopsy instrument steady, allowing careful depth measurement and strict adherence to the proposed trajectory. Frameless stereotactic needle biopsy is often performed under general anesthetic.
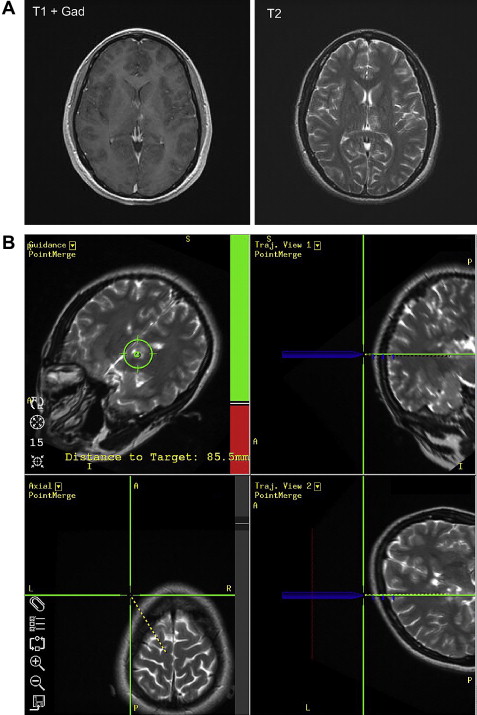
Frameless stereotactic biopsy has been extensively reported in recent years, with diagnostic yields of 85% to 91% and permanent morbidity rates of 3% to 6%. Studies with imaging phantoms have suggested that frameless and frame-based stereotactic biopsy accuracy are comparable. Fiducial markers or surface landmarks used to register images for frameless systems may shift, however, during positioning for surgery. This is not an issue for rigid frame-based systems. Thus, some investigators advocate continued reliance on frame-based biopsy for small or deep lesions. Furthermore, there are potential advantages to frame-based biopsies in terms of reduced use of anesthesia, operating room, and hospital resources, although this may vary with individual practice patterns. In contrast, some investigators have concluded that frameless stereotactic biopsy is comparable with frame bases with regard to diagnostic yield and complications but has distinct advantages. Although a recent series from the Johns Hopkins Hospital found no significant differences in diagnostic yield or complications, more than one needle trajectory was required more frequently to obtain diagnostic tissue from cortical lesions with frame-based compared with frameless stereotaxy. In other studies, increased number of needle trajectories has been strongly associated with increased morbidity. The Johns Hopkins investigators concluded that frameless stereotaxy may represent a more efficient means of obtaining biopsy specimens of cortical lesions but is otherwise similar to the frame-based technique.
Craniotomy for Surgical Resection
Craniotomy and resection has an established role in managing intra-axial primary central nervous system tumors to establish diagnosis and reduce mass effect. Resection is also often used for cytoreduction. Its value in this case, however, remains controversial. This section reviews outcome data for patients undergoing craniotomy and resection of diffuse intra-axial gliomas.
Cytoreductive Surgery for Malignant Gliomas
Malignant gliomas (WHO grade III or IV) include anaplastic (grade III) astrocytomas (AAs), oligodendrogliomas, and mixed oligoastrocytomas as well as glioblastoma multiforme (GBM) (grade IV). Craniotomy and resection of tumor is clearly indicated as a lifesaving measure in patients with raised intracranial pressure and impending herniation. Similarly, smaller tumors centered in deep gray matter (basal ganglia or thalamus) are often biopsied without resection due to the high morbidity associated with attempted surgical resection. Most malignant glioma patients fall in-between these extremes. For these patients, most neurosurgeons generally advocate maximal safe resection. There remains controversy, however, regarding this due to the lack of class I evidence demonstrating a survival advantage for patients under going resection for cytoreduction.
The authors reviewed the literature on surgery for malignant gliomas published between 1987 and 2007. A PubMed search was performed using combinations of the key words, “malignant”, “anaplastic”, “high-grade”, “glioma”, “astrocytoma”, “oligodendroglioma”, glioblastoma multiforme, “surgery”, and “outcome.” In addition, further key publications were identified by cross-references in the resulting articles. Studies were excluded if they did not contain information on outcome with varying degrees of resection, did not determine extent of resection on the basis of postoperative imaging, or did not include formal statistical evaluation. Similarly, studies including children or patients with grade I or II tumors were excluded unless these populations were analyzed separately. Forty-two articles were identified that met the criteria for inclusion. Twenty-eight studies, involving 5874 patients, were identified that found that more extensive resection of malignant gliomas was associated with significantly prolonged survival. Fourteen studies, involving 2122 patients, did not find any significant association.
It is not possible to pool data meaningfully from these disparate studies. Nevertheless, a trend to prolonged survival with more extensive resection is seen when the available data are collated. Several important sources of bias need to be considered, however. The majority of these studies are retrospective and, among prospective studies, only 2 were randomized. One of these was a limited study of 23 patients. Actual survival times often not recorded. Only 4 studies used formal computer-based volumetric assessment to determine extent of resection. Finally, postsurgical treatment (radiation therapy and chemotherapy) varied widely in these studies.
Over the past decade, several investigators have addressed these concerns with varying degrees of success. In 2001, Sawaya and colleagues reported results from their single-institution prospective series, demonstrating that survival was correlated with extent of resection. In 2003, Laws and colleagues reported the landmark findings of the Glioma Outcomes Project (a prospective outcome study in 788 malignant glioma patients from 55 centers across North America). In this study, mean survival for newly diagnosed GBM patients undergoing craniotomy and resection was 49 weeks compared with 21 weeks for those undergoing biopsy. In 2006, Stummer and colleagues published some of the most compelling data supporting cytoreductive surgery to date. In a multicenter randomized controlled trial, patients receiving fluorescence-guided surgery with 5-aminolevulinic acid (5-ALA) (discussed later) had an increased likelihood of gross total resection of enhancing tumor (65% vs 36%, P <.0001). These patients also had a prolonged 6-month progression-free survival when compared with those patients who had their tumor resected using white light (40% vs 21%, P = .0003). Although this study was not powered to detect significant differences in overall survival, a trend was seen in this direction.
Cytoreductive Surgery for WHO Grade II Gliomas
WHO grade II gliomas (diffuse infiltrating astrocytomas, oligodendrogliomas, and mixed oligoastrocytomas) are related to and often progress to become malignant gliomas. Managing patients with grade II gliomas has been controversial. As with malignant gliomas, most neurosurgeons advocate maximal safe resection, based in part on several nonrandomized studies that show trends to improved survival and improved response to adjuvant therapy with more extensive resection. The definition of maximal safe resection, however, differs widely among neurosurgeons.
The authors reviewed the literature from the past 20 years using a similar strategy to that described previously for malignant gliomas. A PubMed search was performed using combinations of the key words, “low-grade”, “grade I”, “diffuse”, “glioma”, “astrocytoma”, “oligodendroglioma”, “oligoastrocytoma”, “surgery”, and “outcome”. In addition, further key publications were identified by cross-references in the resulting articles. Studies without information on outcome with varying degrees of resection, where extent of resection was not determined on the basis of postoperative imaging or where formal statistical evaluation was lacking, were excluded. Similarly, studies including children or patients with grade I, III, or IV tumors were excluded unless these populations were analyzed separately. In addition, studies with short median follow-up (<3 years) were excluded in recognition of the longer median survival seen in most patients with grade II gliomas. The authors identified 10 articles meeting criteria for inclusion. Eight studies, involving 1115 patients, found a significantly positive association between extent of resection and survival. Two studies, involving 1075 patients, did not demonstrate any significant association.
As with high-grade gliomas, the lack of randomized controlled trials precludes meta-analysis of data from these studies. Nevertheless, collated data from these studies suggest a trend to prolonged survival with greater extent of resection. Furthermore, the magnitude of this effect is much greater than that seen with malignant gliomas. Five-year survival for grade II glioma patients is improved from 40% to 71% with simple biopsy to 80% to 98% with gross total resection, suggesting that resection could be associated with prolonged survival of several years compared with biopsy. This is in contrast to GBM patients where median survival after biopsy was 15 to 33 weeks and after gross total resection was 45 to 167 weeks, suggesting resection was often associated with prolonged survival of several months compared with biopsy. Two recent articles are particularly valuable. Smith and colleagues used true computer-assisted volumetric analysis to evaluate the prognostic effect of extent of resection in a retrospective study of 216 adult patients undergoing initial resection of hemispheric low-grade gliomas. Patients with at least 90% extent of resection had 5- and 8-year overall survival rates of 97% and 91%, respectively, whereas patients with less than 90% extent of resection had 5- and 8-year overall survival rates of 76% and 60%, respectively. They concluded that improved outcome among adult patients with hemispheric low-grade glioma is predicted by greater extent of resection. Similarly, Shaw and colleagues examined recurrence in 111 adults less than 40 years of age with cerebral low-grade glioma who underwent a neurosurgeon-determined gross total resection. Overall survival rates at 2 and 5 years were 99% and 93% and progression-free survival rates at 2 and 5 years were 82% and 48%. Three prognostic factors predicted significantly poorer progression-free survival: (1) preoperative tumor diameter greater than or equal to 4 cm; (2) astrocytoma/oligoastrocytoma, histologic type; and (3) residual tumor greater than or equal to 1 cm according to MR imaging. They concluded that young adult patients with low-grade glioma who undergo a neurosurgeon-determined gross total resection still have a greater than 50% risk of tumor progression 5 years postoperatively and should be closely followed and considered for adjuvant treatment.
The grade II glioma studies were subject to the same biases as the high-grade glioma studies. All the studies reported a statistical analysis of association between extent of resection and survival but only 78% actually reported specific 5-year survival percentages. Five-year survival statistics were missing from 1 of the 2 studies that failed to show an association between survival and extent of resection. Coincidentally, this was the largest study that met criteria for inclusion (n = 993). Only 3 studies used volumetric assessments of residual tumor whereas the remainder relied on 2-D estimates. All the studies were retrospective or based on post hoc analysis of data collected prospectively in trials designed to answer questions other than the influence of resection on survival. Postsurgical treatment varied between and within studies. Finally, all the studies were nonrandomized, making them subject to considerable selection bias. Despite these potential biases, most studies demonstrate a compelling association between greater extent of resection and prolonged quality survival for many years. As a result, it is reasonable to strongly advocate early maximal safe resection for patients with newly diagnosed grade II gliomas.
Techniques to maximize safe surgical resection
Advanced Imaging in Brain Tumor Surgery
Imaging studies going beyond purely anatomic data are increasingly incorporated into planning for brain tumor surgery. This imaging generally falls into 2 categories: (1) physiologic imaging (magnetic resonance spectroscopy [MRS], cerebral perfusion, and positron emission tomography [PET]), which can be used to provide information about the nature and extent of the tumor itself; and (2) functional imaging (functional MR imaging, diffusion tensor imaging, PET, and magnetoencephalography), which can provide information about adjacent eloquent brain. This section reviews recent clinical studies about physiologic and functional imaging in brain tumor surgery.
Physiologic imaging
Several imaging modalities have been used to elucidate information about tumor physiology that can be beneficial in surgical planning by highlighting more aggressive areas or further delineating the extent of tumor beyond enhancing margins. For example, proton-based MRS can distinguish tumor (increased choline to N-acetylaspartate [NAA] ratio) from normal tissue (choline:NAA ratio <1) and necrosis (globally decreased metabolites). MRS can identify more malignant areas (greater choline:NAA ratios) within nonenhancing lesions and tumor extension within nonenhancing T2-weighted/fluid-attenuated inversion recovery signal abnormality around an enhancing mass. This information has been used successfully to target stereotactic biopsies and guide extent of resection. Similar information can be obtained from fluorodeoxyglucose PET that demonstrates increased metabolic activity in tumor tissue and decreased metabolic activity in necrosis. Finally, cerebral perfusion studies have been used extensively to identify areas of increased blood flow within tumors. Not only has this been beneficial in identifying more malignant areas within otherwise nonenhancing tumors but also it has been helpful in surgical preparation by identifying areas likely to be more vascular. (See Fig. 3 for examples of physiologic imaging).
Functional imaging
Imaging eloquent areas of brain preoperatively so that their relationships to tumor can be appreciated and they can be avoided at surgery has definite advantages in surgical planning. PET has been used for many years to map eloquent areas of the brain and can be helpful in surgical planning. Functional PET often requires isotopes beyond fluorodeoxyglucose, however, such as 15 O-H 2 O, that are not widely available. As a result, functional MR imaging has recently received more attention. This relies on changes in regional blood flow during task performance to generate a blood oxygenation level–dependent signal, which is an indirect measure of neuronal activity. Similarly, diffusion tensor imaging (DTI) with MR imaging can map subcortical white matter tracts based on local water diffusion characteristics. Functional MR imaging and DTI can be incorporated into preoperative image-guidance systems (discussed later) and have been widely used in clinical neurosurgery for preoperative planning in brain tumor patients. (See Fig. 4 for examples of functional MR imaging and DTI). Ongoing concerns about their sensitivity and specificity, however, suggest that other functional mapping techniques may be necessary. Magnetoencephalography is one such promising technique, based on direct detection of neuronal activity. Small studies using magnetoencephalography in surgical planning for brain tumors have been reported and seem to show a good correlation with intraoperative electrophysiologic mapping. This technology requires significant infrastructure and is not yet widely available.
Image Guidance
Gliomas are diffusely infiltrating by their nature and determining their boundaries at surgery can be difficult. Image guidance using frameless stereotaxy (as described previously for stereotactic biopsies) can be helpful for localizing tumor and for determining extent of resection. This has been described extensively in the past decade. Image-guided resection was associated with improved outcome in 486 newly diagnosed malignant glioma patients in the Glioma Outcomes Project. It was unclear, however, if these improved outcomes were due to the selection of a more favorable patient population (younger and good performance status) or to the image-guidance techniques themselves.
Intraoperative MR Imaging
As most neuronavigation systems are based on preoperative MR imaging, there is potential for significant shift in the surrounding brain as a tumor is debulked. Thus, image-guidance systems may become increasingly inaccurate as tumor resection proceeds. Intraoperative MR imaging is one of the most robust methods to address brain shift during frameless stereotactic-guided tumor resection. Early-generation intraoperative MR imaging scanners tended to be low field strength (eg, 0.2 tesla). Although some investigators maintain that the images produced by such low-field magnets are adequate, many prefer high-field (1.5 tesla or greater) magnets for intraoperative imaging. These systems typically require significant infrastructure with nonferromagnetic instruments but produce images comparable with standard clinical 1.5-tesla MR imaging scanners. Furthermore, they can be used to reregister image-guidance systems. A recent study from the University of California in Los Angeles reported a series documenting extent of resection with varying image guidance and intraoperative MR imaging systems. As expected, extent of resection was generally larger with image guidance than without. Low-field intraoperative or high-field intraoperative MR imaging alone did not improve on this but high-field intraoperative MR imaging with reregistration of a neuronavigation system resulted in the highest degree of resection of any combination of image guidance and intraoperative imaging systems. (See Fig. 5 for an example of imaging obtained with high-field intraoperative MR imaging.)
Electrophysiologic Mapping
Although preoperative functional imaging can be incorporated into many neuronavigation systems, intraoperative electrophysiologic mapping remains the gold standard for identifying functional cortex and subcortical fiber tracts. This is not a new technique, having been originally developed by Penfield and Boldrey in the 1930s to facilitate epilepsy surgery. It has since been adapted to a variety of neurosurgical indications, including intra-axial tumor resection. It has been suggested that, as long as the resection remains exclusively within the tumor itself, the risk of neurologic injury is low. This is not always possible, however, and data from large prospective series suggest that functional neurons occur within tumors adjacent to functional cortex in up to 8% of cases. Mapping motor cortex and subcortical fibers can be done while patients are awake or asleep (with appropriate anesthetic management) but speech mapping requires awake craniotomy and patient cooperation. As experience has grown with speech mapping in tumor surgery, it has become evident that this can allow aggressive tumor resection in brain areas traditionally felt to be inoperable due to their proximity to critical areas for speech. In a recent prospective series of 250 patients with gliomas, Berger and colleagues reported that critical speech areas were identified in 58%. One week after surgery, speech function was worsened in 22%; it resolved in most patients, however, and only 1.6% of surviving patients at 6 months continued to have increased speech deficits.
Surface cortical electrophysiologic mapping is useful for identifying appropriate safe entry points for surgical resection. It does not identify subcortical fibers, however, that may deviate from their surface origin as they pass. For this, subcortical electrophysiologic mapping is critical, particularly for motor mapping, and uses techniques similar to surface mapping and can be used intermittently as resection proceeds.
Fluorescence-Guided Resection
Fluorescence-guided resection relies on administration of a pharmacologic agent that localizes to tumor but not surrounding brain and fluoresces when exposed to light of the appropriate wavelength. Tumor resection can be guided by the fluorescence seen at surgery, potentially allowing a surgeon to identify tumor tissue that otherwise might not be obvious at craniotomy. Fluorescence-guided surgery for brain tumors has been used most commonly with 5-ALA. 5-ALA is orally available and accumulates in glioma tissue where it is metabolized to protoporphyrin IX and fluoresces pink when exposed to light at 375 to 440 nm. Stummer and colleagues have published results of a multicenter randomized controlled trial comparing 5-ALA fluorescence-guided resection with standard white light resection in 322 newly diagnosed GBM patients. Patients undergoing fluorescence-guided resection were more likely to have a gross total resection (65% vs 36%, P <.0001). They were also more likely to be progression-free at 6 months (40% vs 21%, P = .0003). This was not associated with any significant difference in performance status. Although this study was not powered to detect differences in overall survival, it remains a source of some of the most compelling data supporting cytoreductive surgery in glioblastomas.
Techniques to maximize safe surgical resection
Advanced Imaging in Brain Tumor Surgery
Imaging studies going beyond purely anatomic data are increasingly incorporated into planning for brain tumor surgery. This imaging generally falls into 2 categories: (1) physiologic imaging (magnetic resonance spectroscopy [MRS], cerebral perfusion, and positron emission tomography [PET]), which can be used to provide information about the nature and extent of the tumor itself; and (2) functional imaging (functional MR imaging, diffusion tensor imaging, PET, and magnetoencephalography), which can provide information about adjacent eloquent brain. This section reviews recent clinical studies about physiologic and functional imaging in brain tumor surgery.
Physiologic imaging
Several imaging modalities have been used to elucidate information about tumor physiology that can be beneficial in surgical planning by highlighting more aggressive areas or further delineating the extent of tumor beyond enhancing margins. For example, proton-based MRS can distinguish tumor (increased choline to N-acetylaspartate [NAA] ratio) from normal tissue (choline:NAA ratio <1) and necrosis (globally decreased metabolites). MRS can identify more malignant areas (greater choline:NAA ratios) within nonenhancing lesions and tumor extension within nonenhancing T2-weighted/fluid-attenuated inversion recovery signal abnormality around an enhancing mass. This information has been used successfully to target stereotactic biopsies and guide extent of resection. Similar information can be obtained from fluorodeoxyglucose PET that demonstrates increased metabolic activity in tumor tissue and decreased metabolic activity in necrosis. Finally, cerebral perfusion studies have been used extensively to identify areas of increased blood flow within tumors. Not only has this been beneficial in identifying more malignant areas within otherwise nonenhancing tumors but also it has been helpful in surgical preparation by identifying areas likely to be more vascular. (See Fig. 3 for examples of physiologic imaging).
