The field of interventional radiology is facing a growing demand for percutaneous procedures targeting smaller and more complex lesions. Percutaneous medical robots have proven to increase efficiency and accuracy and can address these issues. This review is intended to provide an overview of the functionality and components of these robotic systems for operators learning to use them. We begin by discussing the functions of robots in percutaneous interventions and how they operate. After this discussion, greater focus is then placed on the technical components of robots which help achieve these functions.
Introduction
Percutaneous interventions comprise many of the core procedures performed by interventional radiologists. As the field grows, the demand for these procedures grows, as does the complexity and requirement to target smaller lesions. Precision cancer medicine is improving with novel immunotherapies, creating an increased demand for image-guided biopsies and a requirement to target smaller and harder-to-reach lesions. The introduction of newer imaging navigational systems such as cone-beam CT and fusion MRI have improved lesion targeting. However, these advances have allowed targeting smaller and more complex lesions which are at the limits of human dexterity and accuracy. Microwave ablation (MWA) has also grown in demand, and larger tumors or tumors near blood vessels susceptible to heat sink require composite treatment with multiple needle placements or careful positioning. Both examples serve to demonstrate a demand for more complex interventional radiology (IR) procedures, which percutaneous robots can address. Robotics have demonstrated improved technique efficacy in ablations, improved needle accuracy, and reduced procedure time. ,
There are multiple robots currently approved for percutaneous interventions. These can generally be divided into non-vascular and endovascular robots. The first percutaneous robot approved by the FDA is the Maxio (Perfint Healthcare, Israel). Other non-vascular robots with FDA clearance include: Epione (Quantum Surgical, France), AcuBot (Hopkins/Georgetown, USA), Micromate and Micromate Navi + (Interventional Systems, Austria), iSYS1 (Medizintechnik GmbH, Austria), and the XACT robot (XACT Robotics, Isreal). These systems have proven success in various phantom, animal, and human studies. Endovascular robots face the challenge of a loss of haptic feedback, limiting their current use in practice. Endovascular robots approved for use by the FDA for peripheral vascular interventions include the Magellan (Hansen Medical Inc., USA) and CorPath GRX (Corindus, Siemens Healthineers, USA). The research on these two systems has been mostly limited to small case series and prospective studies but has proven successful efficacy and safety in interventions such as aortic stent grafting, fenestrated endovascular aneurysm repair (FEVAR), and embolization.
The use of percutaneous robots aims to improve needle accuracy using a safe trajectory and decrease radiation exposure to the physician and the patient. However, interventional radiologists have a unique challenge, as the field is highly technology-driven and therefore rapidly evolving, necessitating continuous adaptation to the latest advancements. Since the introduction of medical robots in the last decades, there has been an increasing number of robotic systems used in interventional radiology. , This review serves to provide a breakdown of the components of robotic systems used for percutaneous interventions and their role in robotic procedures. By providing this breakdown, we aim to equip operators learning to use robotic systems. In this review, we will explore the roles of robotic systems in percutaneous interventions and focus on the technical components that enable these functions. Throughout the review, we will emphasize the information through examples from percutaneous robots currently used in practice.
Overview of Robot Functionality
The functionality of robots is tied to the level of autonomy, but procedures for fully autonomous robots can generally be broken down into several steps: pre-procedural path; robotic registration of the physical workspace, imaging, and tools; navigation of tools; trajectory adjustment; and verification of successful procedure completion. The first step of a percutaneous procedure is operative planning and defining a needle trajectory. Robots have currently been integrated with computed tomography (CT) and magnetic resonance imaging (MRI), but ultrasound robots are not widely used in IR. Therefore, this first step involves a pre-procedural image. Currently, trajectory planning software exists and has been integrated with cone-beam CT. However, current robots have not been integrated with trajectory planning software to have this level of autonomy, and the trajectory tends to be set by physician.
After planning, the robot must then orient itself through the process of registration, by which robots match the physical working space to pre-procedural and live images. This allows alignment of the robot’s coordinate system to the patient’s anatomy. Procedural tools, or end-effectors, may also be registered to allow proper navigation and manipulation. The process of registration is key to robotic interventions and may be used throughout the procedure. Therefore, it becomes useful for procedural planning, intraprocedural orientation and navigation, or for post-procedural assessment of the intervention. In general, registration typically occurs through rigid or nonrigid (deformable) methods. Rigid registration utilizes solid anatomical points and can account for the rotation or movement of a patient, whereas non-rigid registration for deformation of soft tissue and organs. Therefore, deformable registration is more flexible and has been proven to be more accurate compared to rigid registration but increases procedural time. ,
For successful needle placement, more autonomous robots must also have the ability to navigate the needle, which is done through the use of a navigational system. Navigation tools have proven to improve needle accuracy while reducing procedural time and radiation dose. Throughout the needle navigation, needle positioning must be verified and adjusted; additionally, the target may move in relation to the trajectory due to organ deformation or patient motion (such as breathing).
Components of Percutaneous Robotic Systems
Structural Organization and Mounting
The set-up and organization of robotic components must ensure that the robot can interact safely with the workspace and patient. The set-up of these systems varies from company to company, but Figure 1 displays an example of how the Epione robotic ( Fig. 2 ) system for non-vascular procedures is configured. Endovascular robotic systems generally have two main components, which are the actual robotic station and a control station. The control station can be positioned outside of the procedural room or in a radiation-shielded area to reduce radiation to the physician. These control stations are composed by several screens, sensors, and joysticks to allow control of the bed-side robot. Whether nonvascular or endovascular, the actual procedure is carried out through the robotic arm and tool, known as an end-effector. For a medical robot to perform successfully, the arm must have sufficient degrees of freedom to perform the procedure. Degrees of freedom refers to the number of independent axes of movement, and percutaneous needle insertions require at least 3 degrees. However, percutaneous robots often have more than this; for example, the iSYS1 system possesses 4, XACT robot possesses 5 ( Fig. 3 ), and AcuBot and Epione both possess 6 degrees of freedom. Robots with 6 degrees of freedom are referred to as anthropomorphic in that they mimic a human arm.
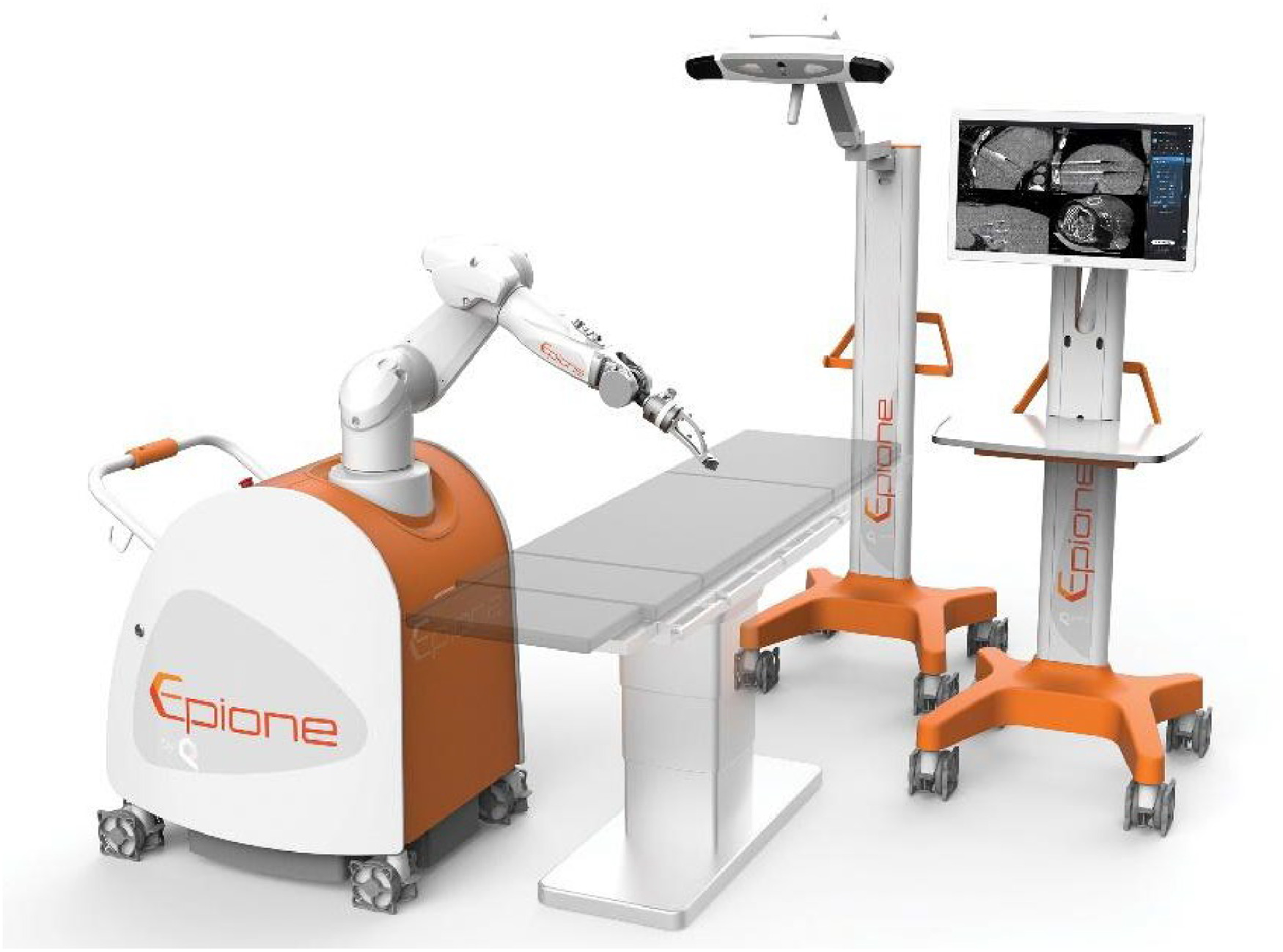
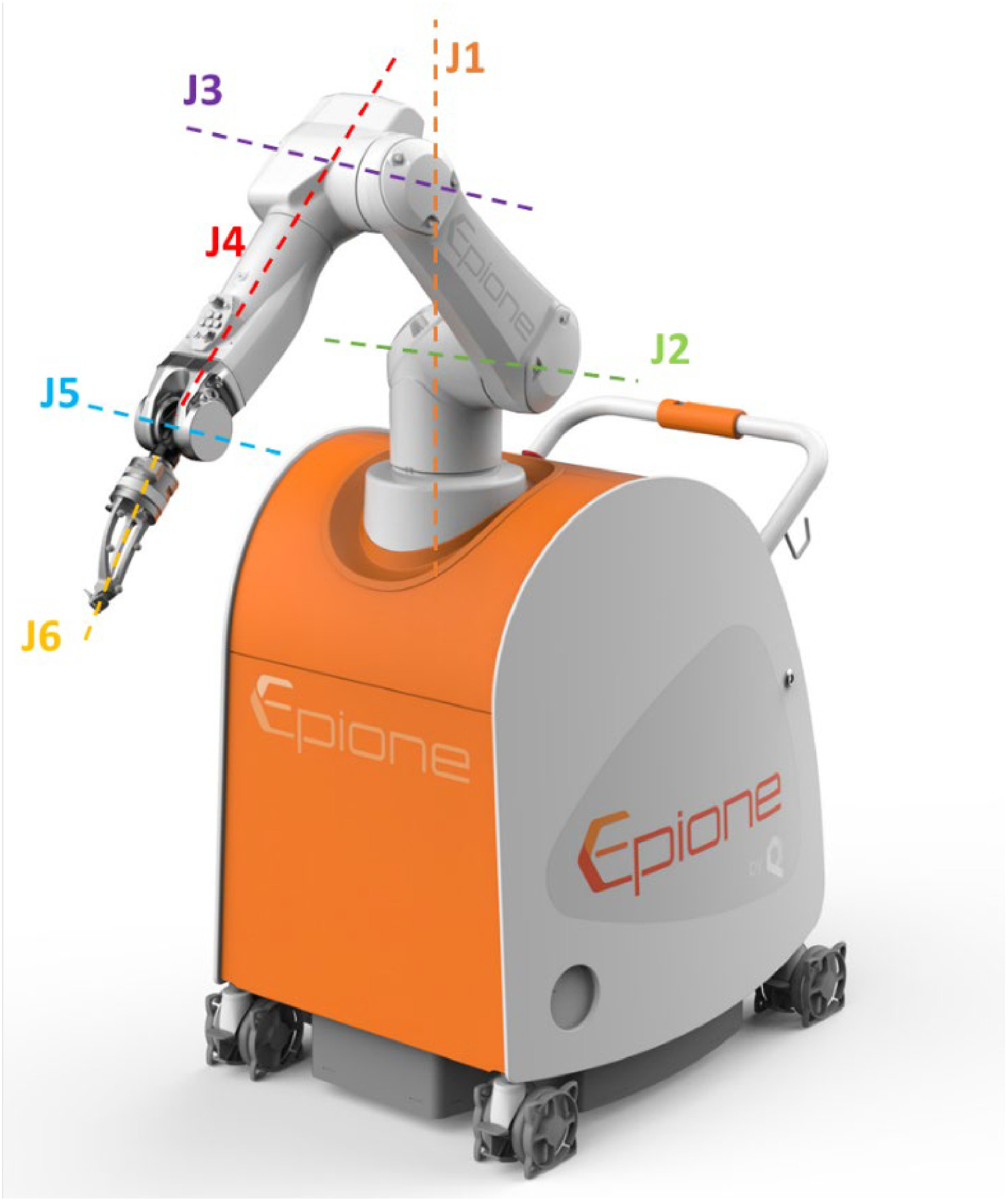
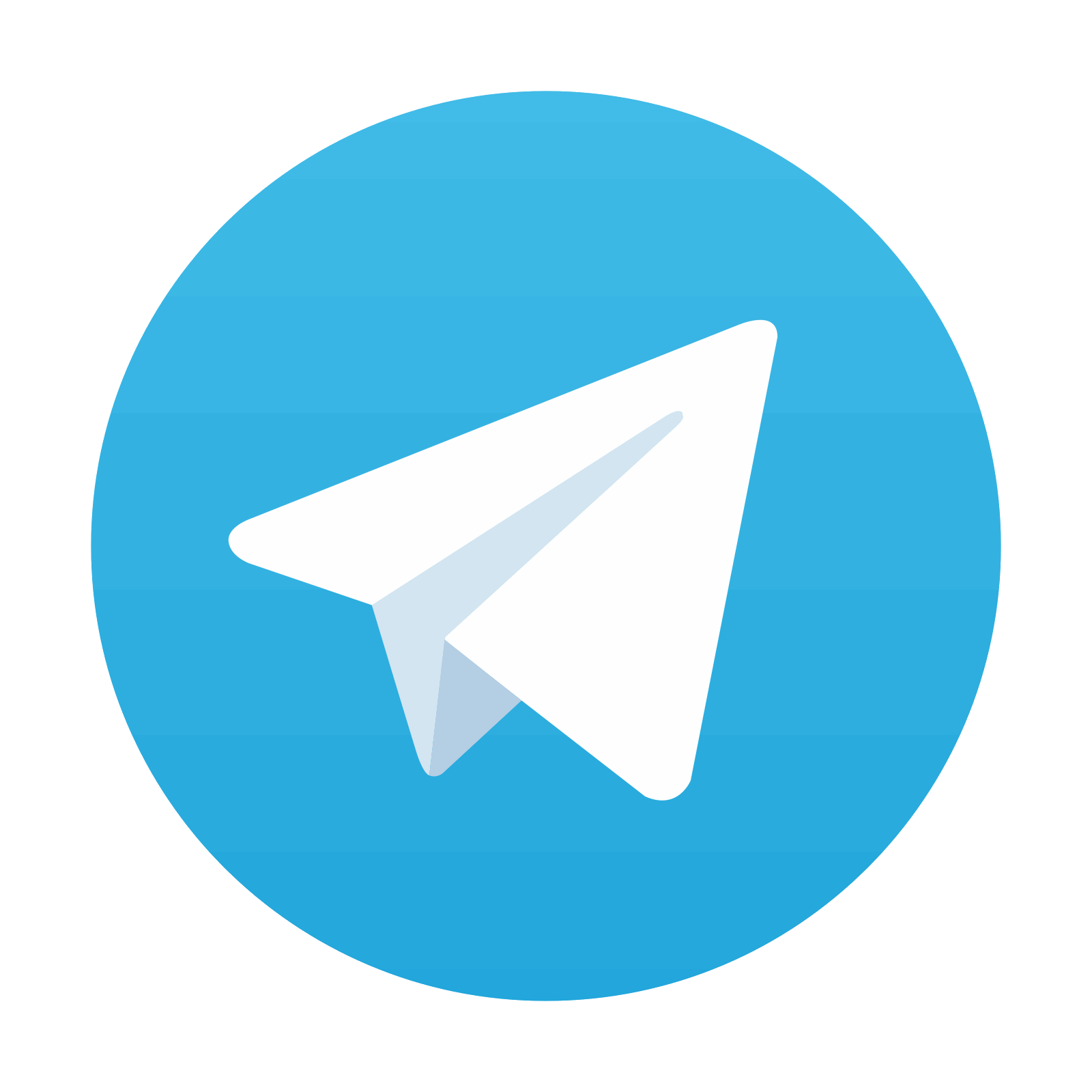
Stay updated, free articles. Join our Telegram channel

Full access? Get Clinical Tree
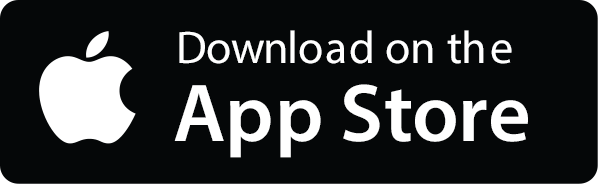
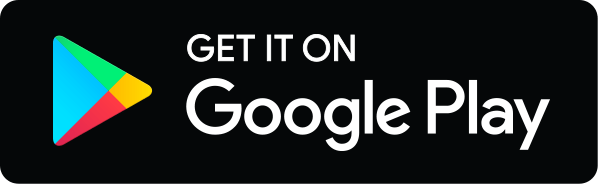
