Fig. 3.1
Illustration of the free diffusion of water molecules in pure water over time interval 2T. The random walks of a selection of molecules are illustrated with red lines. The progression of a small group of molecules highlighted in red is shown in (b–d)
If we were to view this process in three dimensions, we would observe that a labeled cluster of water molecules would spread out in a roughly spherical profile. In free diffusion the progress of molecules is unobstructed by obstacles and is therefore independent of direction. Diffusion that is independent of direction also has a special term: “isotropic diffusion.” Things get more interesting when we consider the effect of the presence of boundaries on the propagation of water. If diffusing water molecules encounter boundaries, such as cell membranes in nervous tissue, the presence of such boundaries will cause a departure from the isotropic, free diffusion we observe in a glass of water. DTI gives us a powerful tool to examine this departure from free diffusion properties, which can give us insight into the geometric properties of the boundaries and allow us to infer details about tissue structure.
In water at room temperature, the average distance moved of a water molecule in one second is around 100 μm, or 1/10th of a millimeter. In 50 ms, which is a time of the order of magnitude relevant to DTI sequences, the average displacement of a water molecule is about 25 μm. This length scale is of a similar magnitude to that of many cellular structures of central nervous system tissue. Hence if we probe the motion of water molecules at such timescales with diffusion tensor imaging, we can probe the geometric structure of the tissue of the central nervous system at the cellular level from outside the body.
Hindered/Restricted/Free Diffusion
We have already covered the concept of “free diffusion,” the diffusion of water molecules unimpeded by the presence of any boundaries. However, in the presence of boundaries, the diffusion properties of water are different. There are two more types of diffusion, which commonly occur in biological tissue: restricted diffusion and hindered diffusion.
Restricted Diffusion
Restricted diffusion occurs when water molecules are constrained by impermeable boundaries, which impedes the progress of water molecules beyond a certain maximum displacement in a particular direction. In the context of biological tissue, this kind of diffusion occurs for water molecules trapped inside a cell boundary, otherwise known as the “intracellular” region . The motion of water molecules within this region is restricted to within a certain range unless they can permeate the cell membrane. In this regime, the diffusion properties of water are largely governed by the geometric properties of the constraining membrane.
Hindered Diffusion
Hindered diffusion occurs when the progress of water molecules as they diffuse is impeded by obstacles, but not completely confined by a continuous boundary as in the case of restricted diffusion. Hence the mean displacement of a set of water molecules is reduced overall in any direction in which boundaries are encountered. The net result is that the average displacement of water molecules is reduced in directions, which impinge on boundaries. In biological tissue, this type of diffusion is exhibited by water present in the interstitial space between cells, otherwise known as the “extracellular” region .
In Fig. 3.2, we can see the difference between molecules exhibiting restricted diffusion and molecules exhibiting hindered diffusion. The red molecules inside the boundaries are restricted and cannot exceed a certain maximum displacement as they are restricted from doing so by the cell boundary. The motion of a single water molecule is exemplified by the red path. The blue molecules, however, can diffuse in any direction. Their progress is impeded, but not restricted, by the presence of the boundaries. A typical path of a “hindered” water molecule is exemplified by the dark blue path. Given enough time, these “hindered” molecules could reach any distance; however, their progress is impeded by the presence of the boundaries. Therefore we see a departure from free diffusion properties, which is dependant on the geometry of the boundaries.
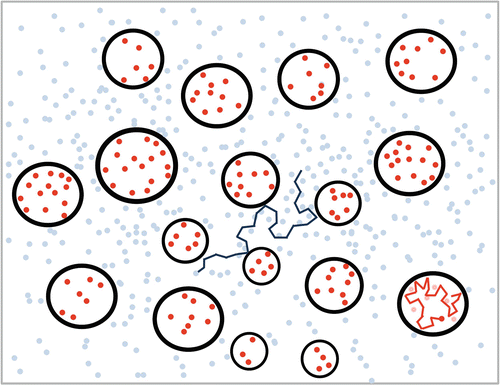
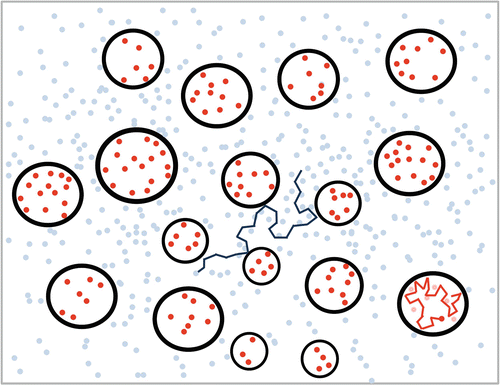
Fig. 3.2
Illustration of hindered and restricted water inside and around boundaries , such as cell membranes. The red dots represent molecules which are restricted by the presence of the boundaries while the blue dots represent molecules which are hindered by the presence of the boundaries
Of particular interest in DTI is the effect of specifically shaped boundaries. It is easy to see from the previously mentioned examples and illustrations how water molecules would behave in and around a spherical boundary, but it is also interesting to consider how a diffusing water molecule would behave inside a cylindrical boundary as in Fig. 3.3. The cylindrical boundary presents an obstacle to diffusion in the direction perpendicular to its axis, while presenting no obstacle to diffusion along its axis. Therefore the diffusion properties of water in and around cylindrical boundaries become highly dependent on the orientation of the cylindrical boundaries. Water diffuses a lot more readily along the axis of a set of packed cylinders than perpendicular to it. This is a phenomenon that becomes highly important in DTI when studying neural tissue, as we will see when we examine the cellular structure of tissue in the next section. There is a special term for this kind of directionally dependent diffusion: “anisotropic diffusion.” The terms “anisotropic” and “isotropic” are regularly used in DTI terminology, so it’s worth taking note of what they mean.
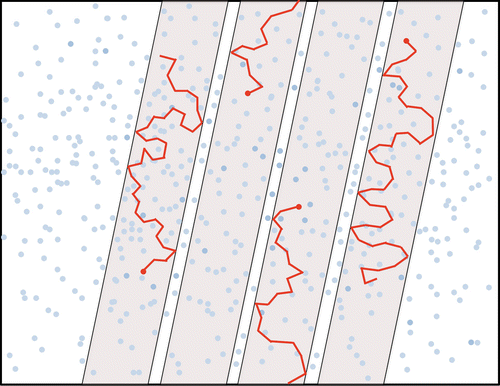
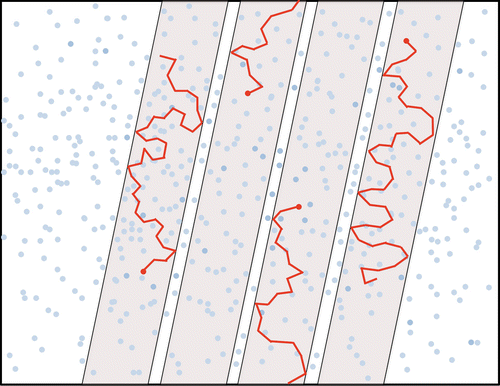
Fig. 3.3
Illustration of water molecules restricted inside a cylindrical boundary . The red lines represent the paths taken by a small selection of molecules trapped inside the cylinders
Microstructural Tissue Properties at the Scale of Diffusion MR Measurements
In biological tissue, such as that of the central nervous system, the components of the cells that make up the tissue create boundaries to diffusion. The various properties of cellular structures such as the cell membrane, the viscosity of the material within the cell, and the presence of subcellular structures such as mitochondria, nuclei, and microfibrils have an effect on the diffusion properties of water in and around the cell and this has an impact on the measurements we take in DTI. In the following, we will examine the cellular composition of central nervous system tissue.
The fundamental building blocks of the brain’s processing network are neurons . Neurons are electrically excitable cells and are typically divided into two major parts, the soma or “body” of the neuron and the neuritic protrusions: dendrites and an axon [6].
The main cellular structures are illustrated in Fig. 3.4. The body of the neuron, the soma, varies in size and shape throughout the central nervous system, typical sizes range from 5 to 100 μm. The soma contains the cell nucleus and other cell structures such as Nissl bodies, mitochondria, and microfibrils.
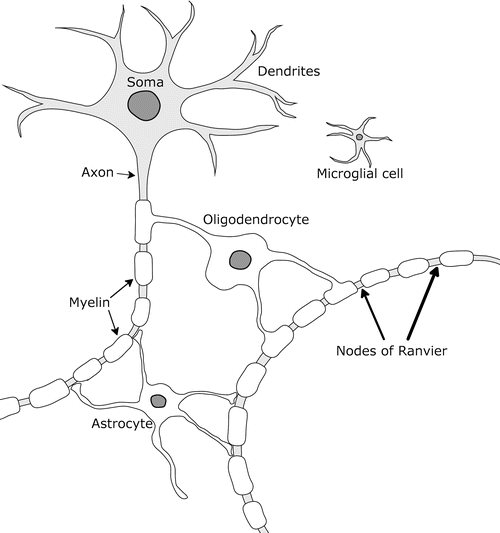
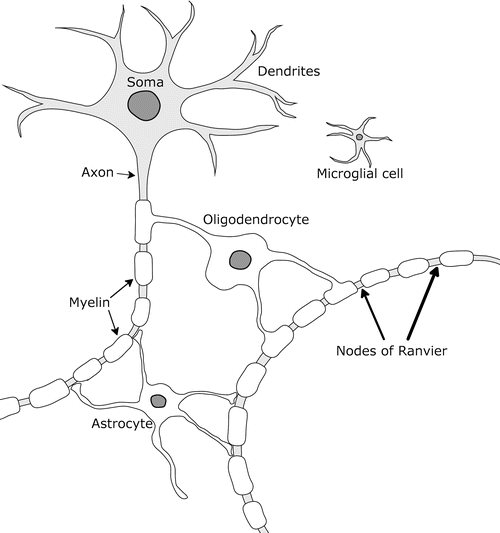
Fig. 3.4
Simple illustration of cellular components of neural tissue including the soma, axons, and glia
The soma has numerous protrusions collectively termed “neurites .” Neurites fall into two types: dendrites and axons. Dendrites are small, short, branched projections which taper towards the end. They facilitate short range exchange of electrical and chemical signals between neighboring neurons and axons. Most neurons typically also have a single axon. The axon is an elongated protrusion of the nucleus which facilitates long range connection with other neurons and there is only one axonal protrusion of each neuron. The longest axons in the bodies of mammals, such as the sciatic nerve in humans, can be many centimeters or even over a meter in length. They can vary in diameter between around 0.2 and 20 μm in the brain [7].
Many axons throughout the central nervous system are covered by a fatty layer known as the myelin sheath. The myelin sheath exhibits periodic gaps called Nodes of Ranvier, which are extremely important for the transmittance of electrical excitation along the axon. Charge builds up at each node and hops to the next one in rapid succession, facilitating fast, efficient communication of electrical excitation or “action potentials” along the axon. The myelin sheath serves to both facilitate the propagation of action potentials and insulate each axon from interference from action potentials traveling in other axons and electrically charged ions in the surroundings. The myelin sheath is composed of several layers, which are formed by the winding of the protrusion of an oligodendrocyte cell around the axon. Each oligodendrocyte can serve many axons. The myelin, made of a multilayered wrapping of a protrusion of an oligodendrocyte cell, is illustrated in Fig. 3.5.
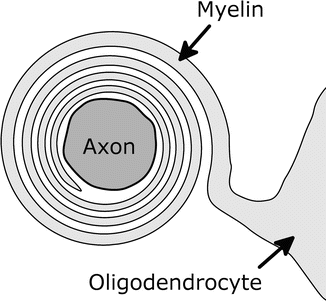
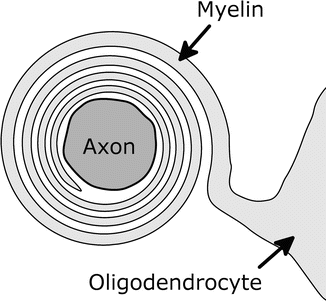
Fig. 3.5
Illustration of the multilayered myelin sheath formed from a protrusion of an oligodendrocyte
There are other types of cells that exist around neurons. The aforementioned oligodendrocyte cells are numerous and serve to provide the insulating myelin sheath to axons. Microglia are small cells which vary widely in size and shape and serve as the immune defence for the central nervous system. They act as macrophages do elsewhere in the body, i.e., identifying and clearing plaques, damaged neurons, and infectious agents. Astrocytes are star-shaped cells that serve a number of maintenance and metabolic functions. They are the most abundant cell type in the brain.
This brings us to an important distinction to make between the two major tissue types in the brain: grey matter and white matter. Figure 3.6 is a highly simplified illustration of the contrast between grey matter and white matter structure.
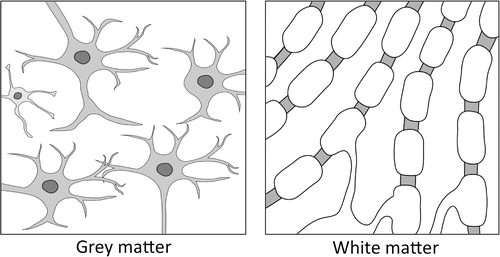
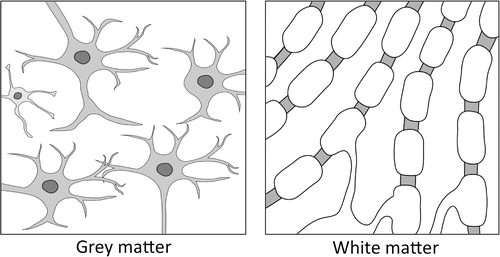
Fig. 3.6
Simple illustration of the contrast between grey matter and white matter
Grey matter is the tissue which can be observed on the surface of the brain; this region is often referred to as the “cerebral cortex.” The cerebral cortex is the outermost layer of neural tissue and is usually 2–4 mm thick in humans. The cerebral cortex is divided up into different areas which are active in cognition, motor, and sensory responses. There are also some deep “subcortical” regions of grey matter, which are located in and around the brainstem and serve many basic purposes, such as cardiac and respiratory regulation, sleep and appetite regulation, and also as relays for connection between multiple cortex regions and the rest of the body. The grey matter mainly consists of the soma, the dendrites, and other cells such as microglia and astrocytes.
The organization of grey matter tissue is highly complex, with many neuron bodies and a complex web of dendrites and glial cells. The diffusion characteristics of water in grey matter are consequently highly complicated, due to complex structural trends in the tissue. As a result of this interpreting DTI measurements in grey matter is very challenging.
White matter is the tissue that lies underneath the cortex and serves to connect neurons in different parts of the brain to form the neural network. It is composed almost entirely of myelinated axons. The name “white matter” comes from the fact that myelin is a white substance; therefore white matter appears white when observed in dissection. The axons in white matter are organized into tight bundles of thousands or millions, which are coherent and tightly packed. Hence diffusion characteristics of water in white matter are often highly directionally dependent. The intracellular water tends to diffuse much more readily along the axis of the axons in a coherent bundle than in any other direction and the extracellular water is also heavily guided by the lattice structure formed by the axons. Intracellular water tends to exhibit restricted diffusion properties, while extracellular water tends to exhibit hindered diffusion properties, both of which were discussed in the previous section. Therefore probing the diffusion characteristics of water in white matter can yield information on the direction of bundles of axons as they traverse the brain. This is of critical importance to DTI as it provides us a very powerful tool to probe the connectivity of the brain .
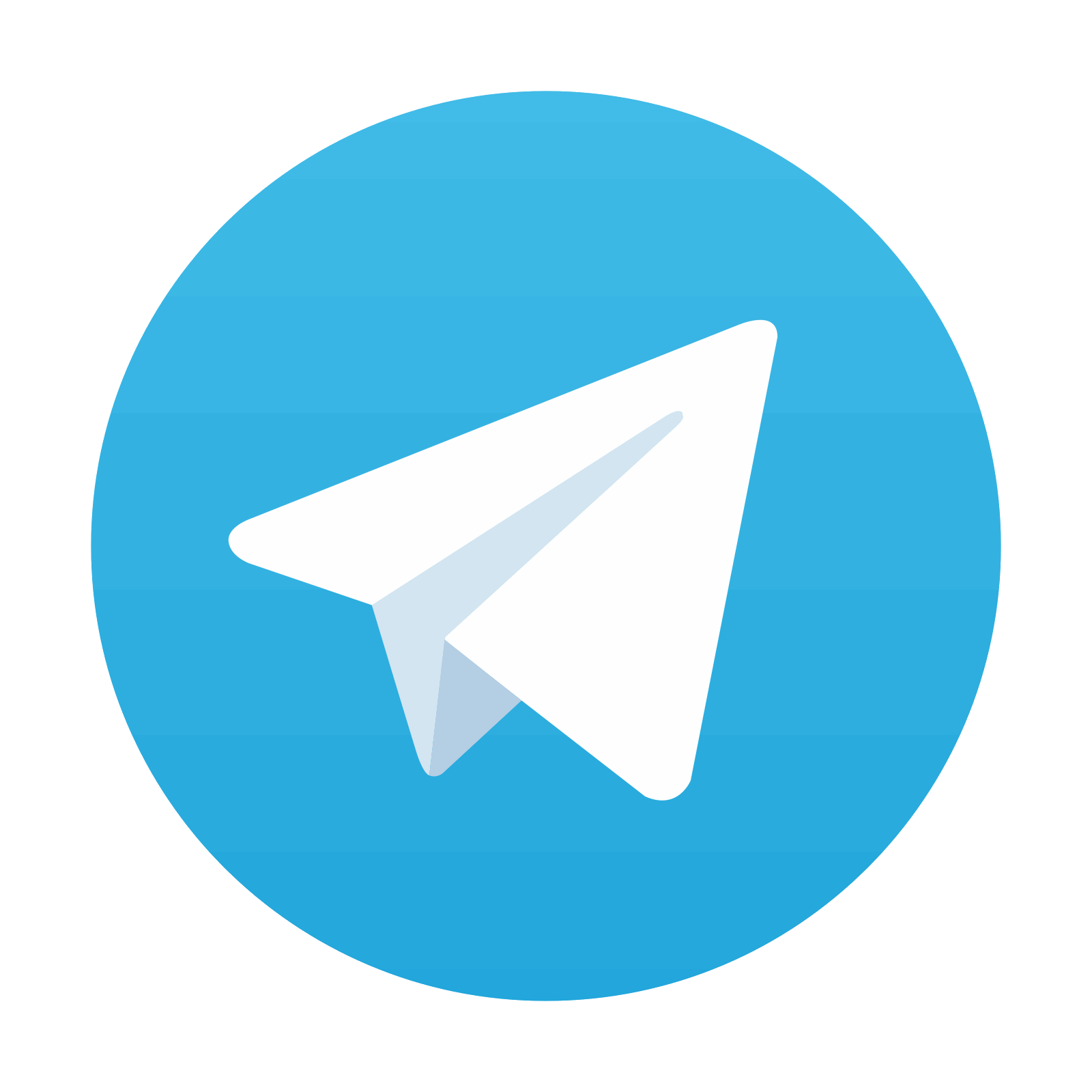
Stay updated, free articles. Join our Telegram channel

Full access? Get Clinical Tree
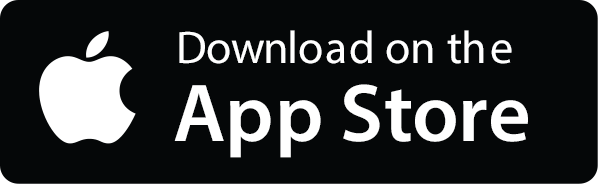
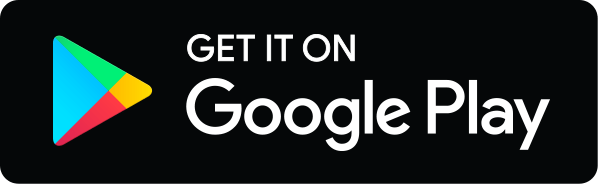