Fig. 6.1
Myocardial flow reserve, resting flow, and spectrum of contractile dysfunction. Progressive worsening of contractile function with reductions in myocardial flow reserve and resting flow
When this myocardial “stunning” proceeds to myocardial ischemia at rest, then it is commonly referred to as myocardial “hibernation” in akinetic or even dyskinetic segments (Fig. 6.1) [6, 9–11]. This accord with observations in an animal experimental model of hibernating myocardium that signify reductions in resting flow or perfusion in viable myocardium may be appreciated as a consequence rather than a cause of adaptive myocardial remodeling [12–14]. The decrease of resting myocardial perfusion or flow in the hibernating-stunning myocardium has been suggested to reflect the development of metabolic alterations, including a downregulation of mitochondrial oxygen consumption, or an adaption to the dysbalance between oxygen demand and supply to avoid structural disintegration and/or cellular death of the myocytes and tissue fibrosis. In a clinical investigation with PET perfusion and viability assessment, Zhang et al. [15] could unravel that reduced myocardial perfusion at rest was accompanied by a commensurate decrease in absolute myocardial blood flow (MBF) and on average less severe in viable than nonviable myocardium. Yet, there was a considerable variety in perfusion and MBF values between individuals and myocardial regions, so that the severity in reduced resting flow could not fully differentiate between viable and nonviable myocardium (Fig. 6.2).
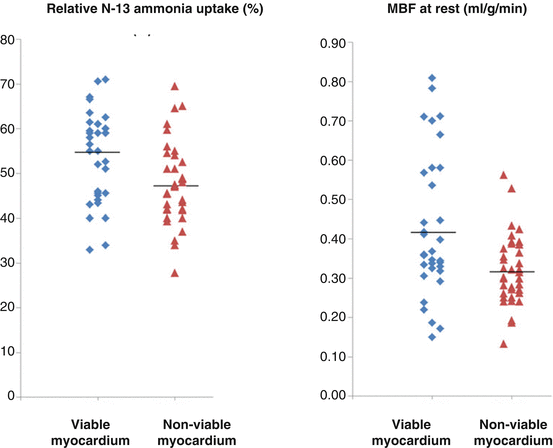
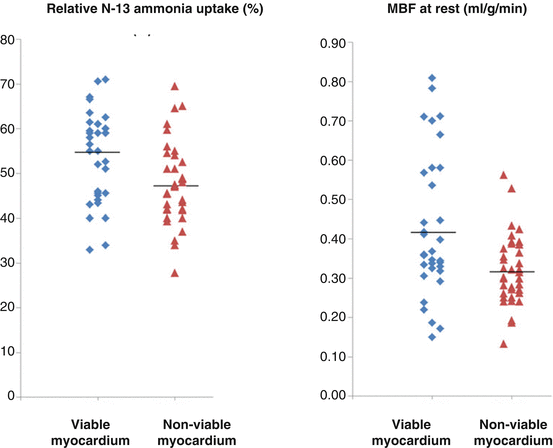
Fig. 6.2
Perfusion and myocardial blood flow (MBF) in viable and nonviable myocardium. Relative reductions in perfusion and MBF at rest in viable myocardial regions and nonviable myocardial regions. Of note, a considerable overlap of flow values between the two kinds of regions (With kind permission from Ref [15])
Of particular interest, the relative FDG uptake in the viable regions was linearly related to the resting MBF, while this was not observed in the nonviable regions (Fig. 6.3). Such observations put forth that the higher the amount of viable myocardium, the higher is the resting MBF to adequately meet increases in oxygen demand. In addition, the extraction of FDG and, thus, of glucose in the viable myocardium correlated inversely with the myocardial flow reserve (MFR) (Fig. 6.4). The more severe the reduction of MFR, the highest was the FDG extraction and, therefore, the myocardial glucose utilization. This strongly outlines that a severe impairment of the MFR rather than a stenosis-related, downstream reduction in resting coronary flow as a major determinant of myocardial viability. This consideration also agrees with an experimental animal model of myocardial hibernation, where FDG uptake was highest in myocardial territories with MFRs of less than 1.5 [16]. Severe reductions of MFR, therefore, are an important determinant for the stimulation of an adaptive myocardial process consistent with myocardial hibernation [16]. Such an initial adaptive process, however, may ultimately advance to myocardial cell death and tissue fibrosis, if coronary flow is not restored in a timely fashion [17–19].
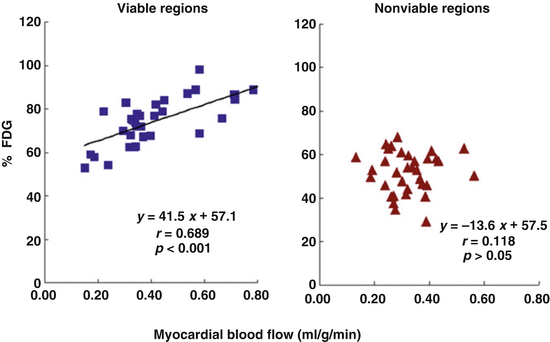
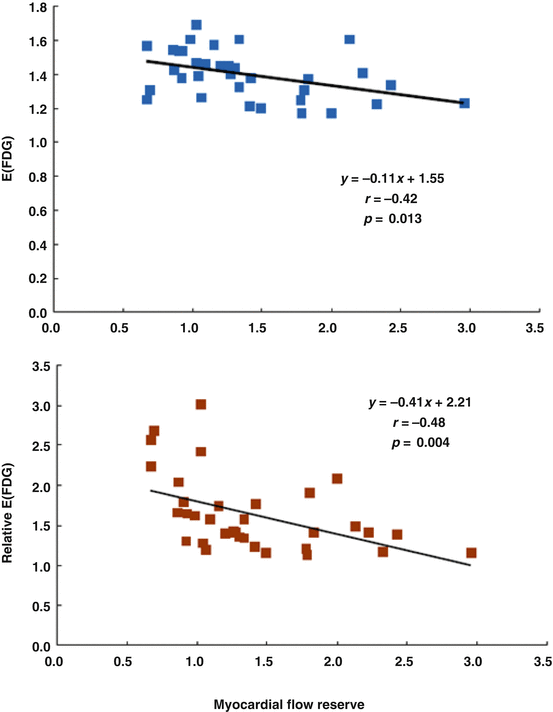
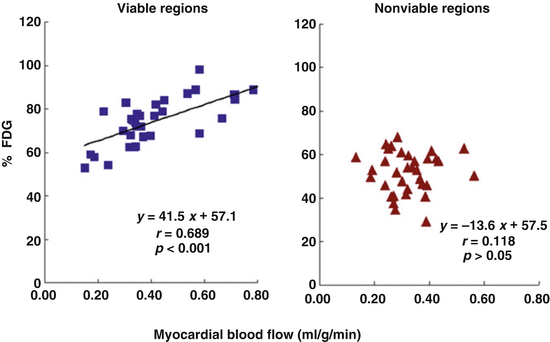
Fig. 6.3
Relative FDG uptake in comparison to regional myocardial blood flow (MBF) at rest (% FDG, relative regional FDG uptake). A coupling between FDG uptake and MBF can be appreciated in viable regions, while this is not the case for nonviable regions (With kind permission from Ref. [15])
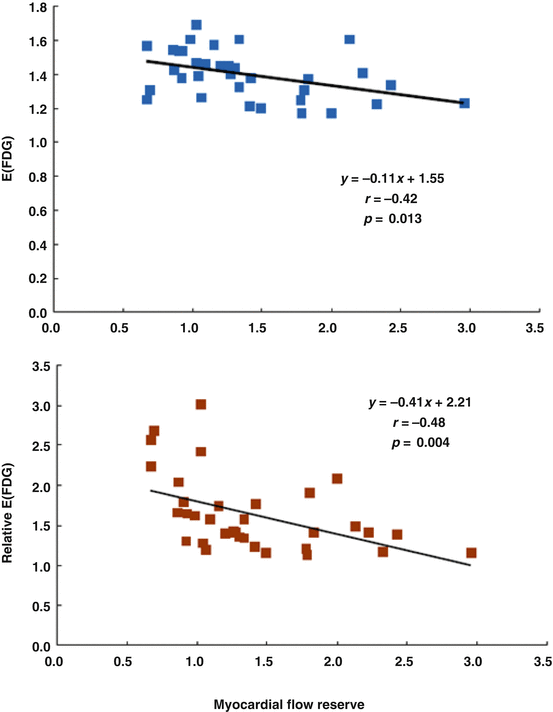
Fig. 6.4
Relationship between myocardial flow reserve (MFR) and extraction of FDG in viable regions. E(FDG), index of the regional FDG extraction as the ratio of relative FDG over relative 13N-ammonia tissue concentrations; relative E(FDG) as shown on the ordinate of the figure, FDG extraction in viable myocardium normalized to E(FDG) in remote myocardium. As it can be appreciated, there is an inverse relationship between extraction of FDG and MFR in areas of viable myocardium (With kind permission from Ref. [15])
Stunned and/or hibernating myocardium may completely or partially recover its function again in a substantial number of patients, in whom coronary flow is restored by interventional or surgical revascularization as evidenced by numerous clinical investigations [9, 10, 20, 21]. Imaging of this so-called “hibernating-stunning” or ischemically compromised but viable and dysfunctional myocardium has evolved as a critical step of the clinical assessment of ischemic heart failure patients [22–25]. In this respect, “myocardial viability” is commonly referred to as impairment in regional myocardial contractile function (akinesia or dyskinesia) that is potentially reversible if revascularized [26–28]. Although there is a variety of nuclear cardiology techniques for the detection of myocardial viability in ischemic heart failure patients, such as 201thallium (210TI) imaging using a rest-redistribution or stress-rest-reinjection protocols [29], rest-redistribution 201TI imaging as part of a dual-isotope protocol with a technetium-99m perfusion tracer [30], or imaging with a technetium-99m tracer at rest after the administration of nitroglycerin [31], fluorine-18-labeled fluorodeoxyglucose (FDG)-PET has evolved as the standard of reference for the identification of viable myocardium in patients with ischemic cardiomyopathy [6, 21, 28]. Employing FDG-PET for the detection of viable myocardium in cardiomyopathy patients, the mean sensitivity and specificity are reported to be 92 and 63 %, respectively [28, 32]. Viable and ischemic jeopardized myocardium, however, is preferentially identified with PET alone or in concert with SPECT perfusion imaging [21, 28]. The concurrent assessment of myocardial perfusion and viability affords the identification of a “match” (nonviable myocardium: reduced blood flow with concurrent reduction in glucose utilization) and “mismatch” (viable myocardium: reduced blood flow combined with enhanced glucose utilization) patterns (Fig. 6.5a, b) [33]. The latter perfusion-viability “mismatch” pattern is commonly regarded as the hallmark of myocardial hibernation [10, 34].
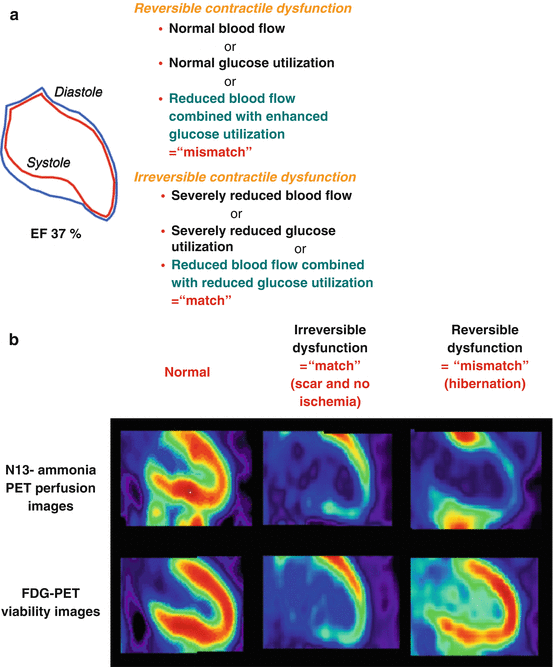
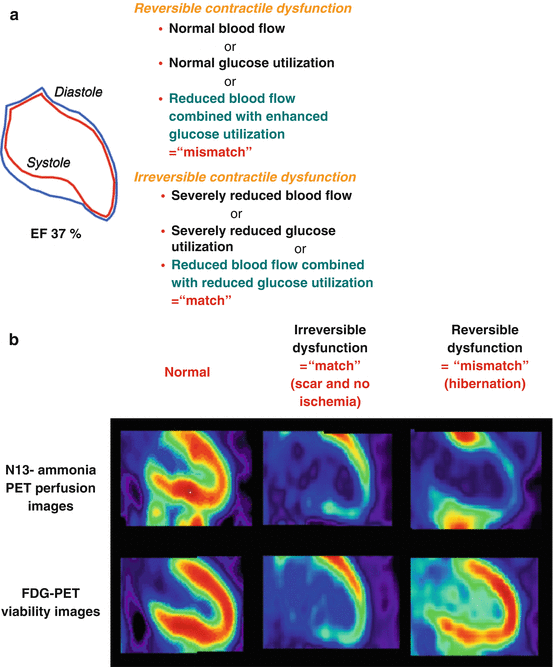
Fig. 6.5
(a) Contractile function, flow, and FDG (glucose) uptake. Reversible and irreversible contractile dysfunction in akinetic myocardial segments as defined by SPECT and/or PET assessment of myocardial perfusion and metabolism. (b) Reversible and irreversible contractile function. Examples of normal perfusion and FDG uptake (left panel), “match” finding with concordant reduction in perfusion and metabolism in the anteroapical and inferior wall, indicative of non-transmural and transmural scar without ischemia (middle panel), and “mismatch” finding with reduced resting perfusion anteroapical, apical, and inferoapical and preserved metabolism, suggestive of classical hibernating myocardium (With kind permission from Ref. [33])
A pooled analysis of 17 investigations (including SPECT perfusion and FDG-PET) signified an increased diagnostic performance with a positive predictive value of 76 % (range 52–100) and a negative predictive value of 82 % (range 67–100 %) [32, 35]. The presence and extent of myocardial viability with FDG-PET have been closely related to various clinical outcome parameters [6, 21].
In the clinical scenario, cardiac FDG-PET, or nowadays PET/CT, should be applied in cardiomyopathy patients with a LVEF ≤35 %, who present a stress-rest fixed perfusion defect of ≥4 and akinetic segments of the left ventricle as determined with SPECT or PET/CT perfusion imaging. Adding FDG-PET/CT may unmask the following four conditions divided into two groups such as:
“Match” findings between perfusion and viability assessment, indicative for:
(a)
Transmural necrosis
(b)
Non-transmural necrosis and no ischemic component
Or “mismatch” findings, denoting:
(c)
Non-transmural necrosis with viable but ischemic compromised myocardium
(d)
Complete viable and ischemic compromised myocardium
In about 20–40 % of these patients [32, 36], FDG-PET/CT is likely to detect sufficient amount of viable myocardium unmasking viable but ischemic compromised myocardium (c and d). This so-called hibernating-stunning myocardium, if large enough (≥4 segments), principally may benefit from restoration of myocardial flow or perfusion in terms of recovery of left-ventricular function and clinical outcome (Fig. 6.6a–c) [6, 23, 32]. If such patient presents also suitable coronary anatomy and clinical condition, he is commonly referred for coronary revascularization (Fig. 6.7). Conversely, in the absence of hibernating-stunning myocardium, the patient will undergo optimal medical heart failure treatment. If over time, however, a further worsening of left-ventricular function despite optimal medical heart failure treatment ensues, heart transplantation may be considered.
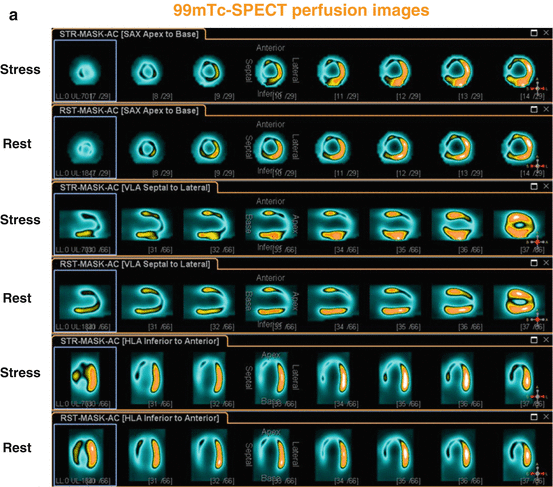
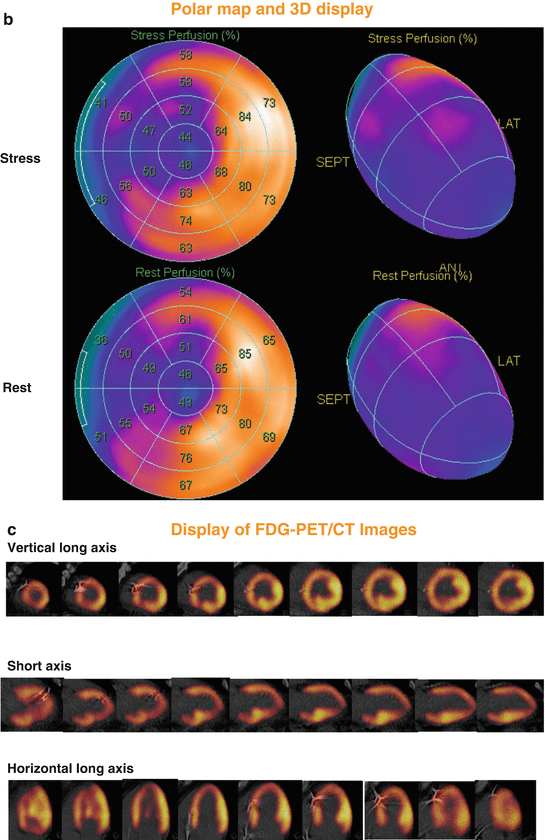
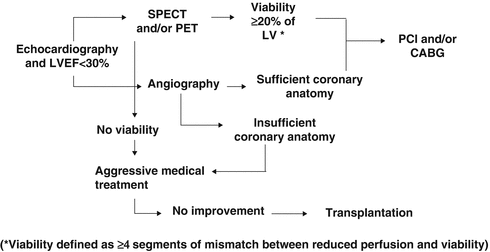
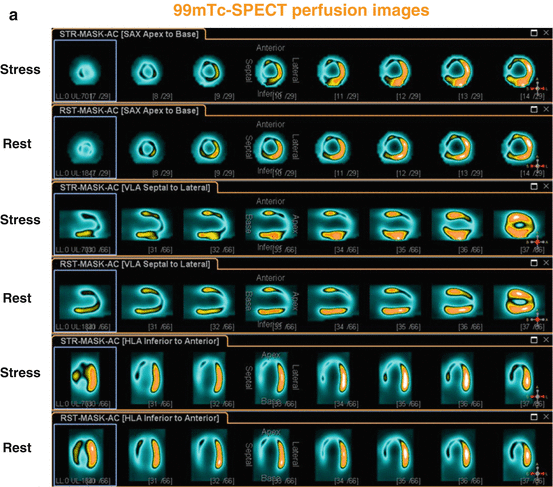
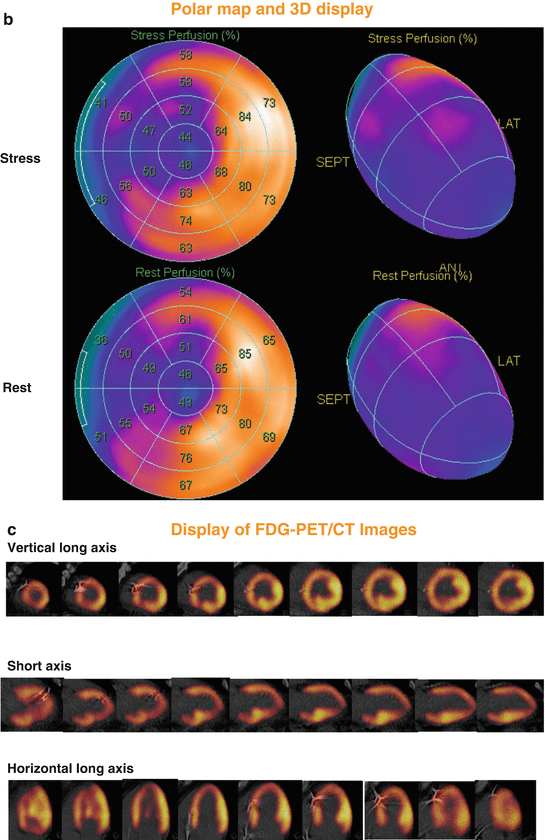
Fig. 6.6
99mTc-SPECT/CT perfusion images in a 56-year-old man presenting with recent non-ST-elevation myocardial infarction and stable coronary artery disease. Short, vertical, and horizontal long axis demonstrate a widely fixed and extended perfusion defect in the anterior, septal, apical, and infero-septal wall (a), corresponding polar map and three-dimensional display of 99mTc-SPECT/CT perfusion images (b), with a “mismatch” (widely preserved although diminished FDG uptake on PET/CT images) (c), consistent with hibernating myocardium in the LAD and RCA territory. Invasive coronary angiography demonstrated two proximal LAD lesions with ≈ 90 %, LCx with ≈ 40–50 %, and RCA with ≈ 90 % stenosis diameter. The patient underwent a percutaneous coronary intervention with dilation of stenosis and stent implantation in LAD and RCA lesions. Echocardiographic follow-up examination after 3 months demonstrated a marked improvement of global left-ventricular ejection fraction from 25 to 40 % ( 99m Tc technetium-99, CT computed tomography, FDG, fluorine-18-labeled fluorodeoxyglucose, LCx left circumflex coronary artery, LAD left anterior descending coronary artery, PET positron emission tomography, RCA right coronary artery, SPECT single-photon emission computed tomography) (With kind permission from Ref. [33])
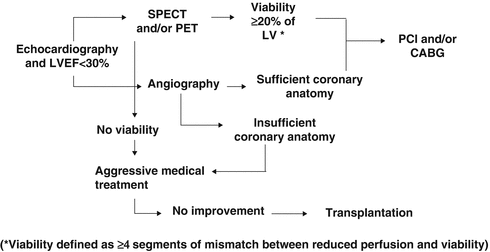
Fig. 6.7
Algorithm to stratify patients with ischemic cardiomyopathy for treatment options. Diagnostic and decision-making process in patients with ischemic cardiomyopathy. (CABG coronary artery bypass grafting, LV left ventricle, LVEF left-ventricular ejection fraction, PCI percutaneous coronary intervention, PET positron emission tomography, SPECT single-photon emission computed tomography) (With kind permission from Ref. [33])
Regarding the functional recovery of ischemic compromised myocardium in heart failure patients, observational investigations have demonstrated an improvement of regional and global left-ventricular ejection fraction (LVEF) after successful revascularization of viable myocardial segments as identified by FDG-PET [10, 21, 32, 37, 38]. In particular, the preoperative extent of a flow-metabolism mismatch was closely related to the magnitude of improvement in post-revascularization heart failure symptoms (r = 0.65, p < 0.001) (Fig. 6.8) [38].
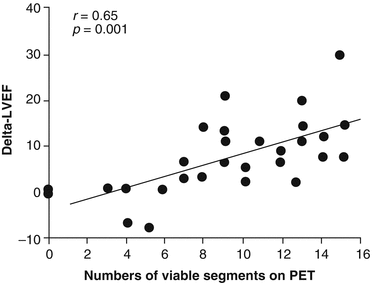
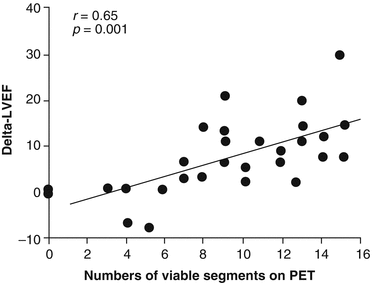
Fig. 6.8
Relationship between the extent of a blood flow-metabolism “mismatch” by PET and the change in the left-ventricular ejection fraction (LVEF) after coronary revascularization. As it can be appreciated the extent of the blood flow-metabolism mismatch is paralleled by the increase in LVEF after revascularization (With kind permission from Ref. [38])
Receiver operating curve (ROC) analysis signified that a viability extent of ≥18 %, associated with a sensitivity of 76 % and a specificity of 78 %, had the greatest clinical benefit in improvement of functional status [38]. While the minimum required amount of viable myocardium necessary for a functional recovery after coronary revascularization remains controversial [6], the term “functionally” significant amount of viable myocardium commonly signifies more than 20 % (≥4 out of 17 segments) of the left ventricle. This amount of viable but ischemic jeopardized myocardium is commonly regarded as “functionally” significant, which may recover contractile function after restoration of coronary blood flow [39]. When applying this threshold of 20 % of viable myocardium, functionally significant viability can be anticipated in 25 % of patients with ischemic CMP, who might benefit from coronary revascularization [36]. Apart that restoration of coronary flow to areas of a sufficient amount viable myocardium may improve regional left-ventricular function in areas, it also beneficially affects the remodeling process of the left ventricle [6, 17, 28].
Conversely, when dysfunctional but viable myocardium of less than 20 % of the left-ventricular myocardium is identified, then coronary revascularization may still lead to a significant improvement in clinical outcome or prognosis in these ischemic cardiomyopathy patients, while functional recovery is less likely [23]. As reported in two prospective trials [39, 40], even in the presence of a minimum of only 5 or 7.6 % of viable myocardium, respectively, an improved clinical outcome after revascularization may ensue. This is in agreement with a more recently post hoc analysis of the prospective randomized trial, PET and Recovery Following Revascularization-2 (PARR-2) trial. It was reported [41] that a cutoff of ≥7 % perfusion-viability mismatch indicated that ischemic cardiomyopathy patients would benefit from coronary revascularization at least prognostically.
Since most clinical investigations assessing the association between revascularization of viable myocardium and improvement in left-ventricular function, symptoms and prognosis in ischemic cardiomyopathy patients were performed prospectively in an observational fashion or in a retrospective analysis, a certain evaluation bias may apply. Notably, it is likely that the decision-making process to perform coronary artery bypass grafting (CABG) and/or percutaneous intervention (PCI) was driven by the results of imaging testing to identify viable myocardium. Further it remains uncertain whether adjustment for pivotal baseline variables was appropriate and whether patients who were not referred for CABG received adequate medical therapy for heart failure. To address these issues, the Canadian PARR-2 trial was conducted in a randomized, controlled design in order to evaluate whether the use of FDG-PET in the clinical decision-making process manifested in a better clinical outcome as compared to standard care where PET was not available [42]. In this multicenter trial, patients with a LVEF ≤35 % and suspected ischemic heart disease, who were being considered for coronary revascularization, transplantation, or heart failure work-up, were included for study purpose. In the FDG-PET arm of this trial, the extent and severity of scar and viable but ischemic compromised myocardium were assessed and evaluated in the context of a previously derived model in the prediction of left-ventricular recovery after revascularization. Employing the results of this model and the interpretation of PET images, the physician and surgeon decided on whether or not to proceed with coronary revascularization procedures. Interestingly, there was a trend for improved clinical outcome and survival using the PET strategy as compared with standard care, but it did not reach statistical significance between the two groups (Figs. 6.9 and 6.10).
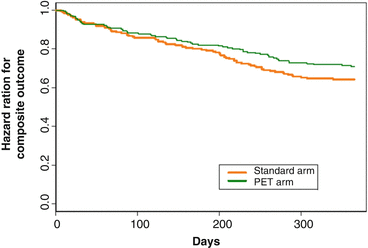
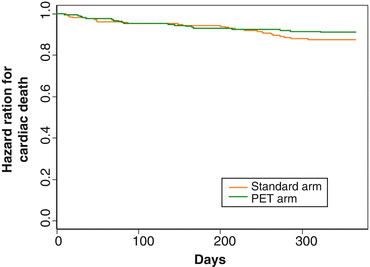
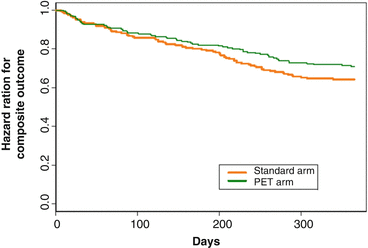
Fig. 6.9
“Survival Curves” (on the Basis of Time to First Occurring Outcome of the Composite Event). Mantel-Haenszel (log-rank) test for differences between 2 survival curves; chi-square =2.1, hazard ratio = 0.78, 95 % CI 0.58–1.1, p = 0.15 (With kind permission from Ref. [42])
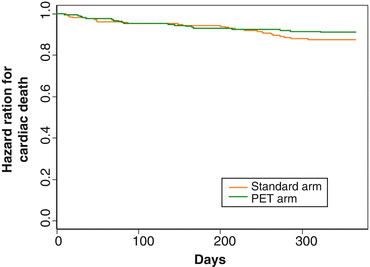
Fig. 6.10
“Survival” Curves (on the Basis of Time to Cardiac Death) for All Individuals. Mantel-Haenszel (log-rank) test for differences between 2 survival curves; chi-square = 1.3, hazard ratio = 0.72, 95 % CI 0.4–1.3, p = 0.25 (With kind permission from Ref. [42])
When performing a post hoc analysis that compared those that adhered to PET recommendations (ADHERE arm), a significantly improved outcome in the PET-guided group than in the one with standard care was noted (Fig. 6.11) [43].
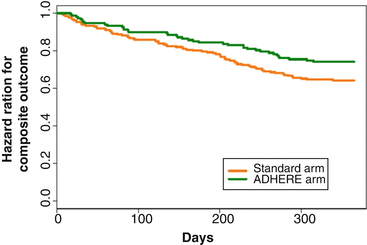
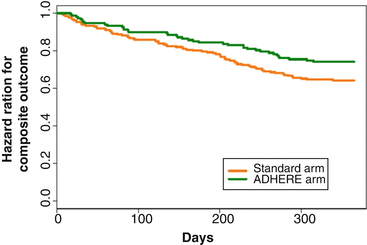
Fig. 6.11
Survival Curves (on the Basis of Time to First Occurring Outcome Out of the Composite Event). The positron emission tomography adherence group versus standard care arm. Mantel-Haenszel (log-rank) test for differences between 2 survival curves; adjusted hazard ratio = 0.62, 95 % CI 0.42–0.93, p = 0.019 (With kind permission from Ref. [42])
Additionally, it was appreciated that there was a benefit of outcome in experienced centers, in which FDG-PET was routinely used for the decision-making process as regards coronary revascularization or optimal medical treatment of heart failure alone in ischemic cardiomyopathy patients [41]. Taken together, the results of the PARR-2 trial, its sub-studies, and previous observational outcome data support the utility of FDG-PET with the “match” and “mismatch” concept between myocardial perfusion and viability in the identification of high-risk ischemic cardiomyopathy patients, who may benefit from coronary revascularization in terms of recovery of left-ventricular function, associated with improved exercise capacity and reduced symptoms, and clinical outcome.
6.2 Considerations to STICH Trial
More recently, the STICH (Surgical Treatment for Ischemic Heart Failure) trial [44] was performed in a randomized and blinded fashion evaluating whether the proof of viable myocardium as determined with single-photon-emission computed tomography (SPECT), dobutamine echocardiography, or both and restoration of coronary flow improved clinical outcome [44]. Overall, 1,212 ischemic heart failure patients were randomly assigned to receive medical therapy alone or medical therapy plus CABG. In 601 of these patients, information on myocardial viability was available [44]. At first sight, the presence of viable myocardium was associated with a greater likelihood of survival in patients with CAD and left-ventricular dysfunction (Fig. 6.12). Yet, this relationship did not hold any more after adjustment for other baseline clinical variables (Fig. 6.13). Unexpectedly, the assessment of myocardial viability with SPECT or dobutamine echocardiography did not prove to be superior in identifying those patients who are likely to benefit in respect to survival from CABG as compared to those who received optimal with medical therapy alone [44].
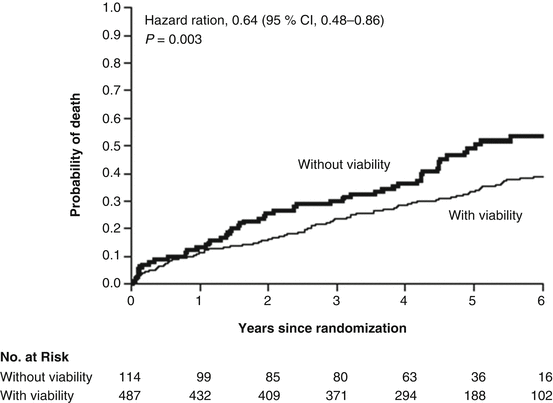
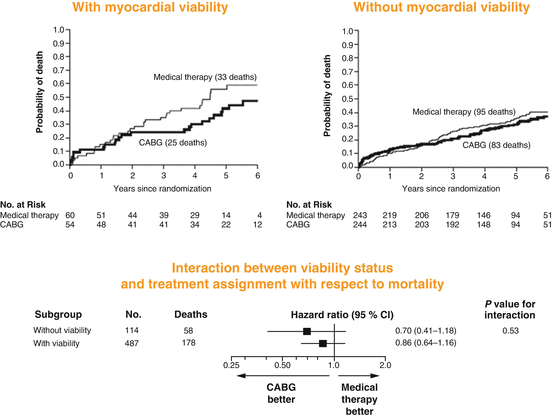
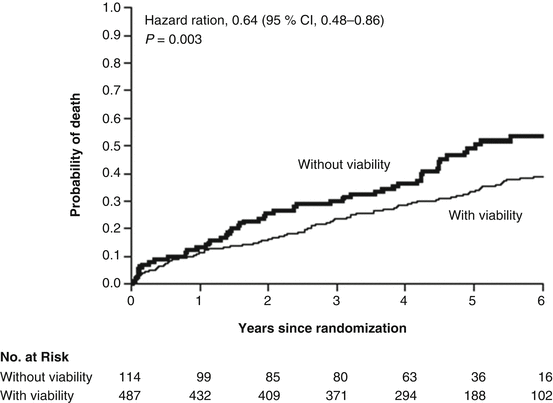
Fig. 6.12
Myocardial Viability and Survival in Ischemic Left-Ventricular Function, the STICH trial. Kaplan-Meier analysis of the probability of death according to the viability status. Comparison not adjusted for other prognostic baseline variables (With kind permission from Ref. [44])
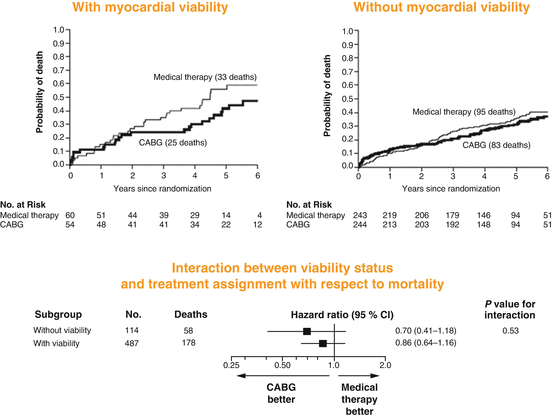
Fig. 6.13
Myocardial Viability and STICH. Kaplan-Meier analysis of survival after adjustment for other prognostic baseline variables such as LVEF, NYHA classification, and renal insufficiency, number of diseased vessels, previous revascularization, and previous myocardial infarction (With kind permission from Ref. [44])
There are certain limitations of the STICH trial worthy of considerations. Patients in the STICH trial had all CAD that was amenable to CABG. Consequently, subgroup analysis of the STICH trial assumes that patients could have been referred either for CABG or medical therapy alone. Given this assumption, many patients with CAD and severely reduced left-ventricular function were not included for study purpose. For example, CAD patients in whom CABG is commonly advised, such as patients with significant left main stem stenosis and/or with severe angina symptoms, were not enrolled. Conversely, patients in whom CABG is commonly denied due to a very high risk of cardiac surgery, limited functional capacity, or reduced likelihood of survival were also not part of the study trial. Another study bias may be seen in a substantial number of patient’s crossing over from medical therapy to CABG due to instability (angina symptoms, cardiac decompensation) in the first year after enrollment. In a post hoc subanalysis, it was appreciated that these patients with ischemic cardiomyopathy, who crossed over to CABG, had a far better outcome than those who had medical therapy alone [45]. Importantly, the observed difference favoring CABG in this subpopulation with clinical instability was independent of the baseline risk of the individual patient. Conversely, there are also a certain number of patients foreseen for CABG that crossed over to medical therapy alone due to patient or family preferences. This may also have confounded the outcome data.
The findings of the STICH trial, however, contrast previous observational investigations or retrospective data analysis in the assessment of myocardial viability in ischemic heart failure patients [6, 9, 10, 21] and thus were not anticipated by many clinicians, which have issued considerable comments and criticism [46–48]. Several factors while several factors, however, may reconcile, at least in part, this controversy in viability assessment, treatment, and clinical outcome of ischemic cardiomyopathy patients, such as (1) the timing of coronary revascularization, (2) absence or presence of ischemic compromised but viable myocardium, (3) stage of myocardial remodeling process, (4) ischemic conditioning, and (5) suboptimal imaging protocols and techniques to determine the presence or absence of ischemic jeopardized but viable myocardium (Table 6.1).
Table 6.1
Factors potentially affecting the effect of flow restoration on viable but dysfunctional myocardium
1. Timing of coronary revascularization |
2. Extent and severity of myocardial ischemia in dysfunctional but viable myocardium |
3. Myocardial remodeling |
4. Left-ventricular dilation |
6.3 Timing of Coronary Revascularization
A meta-analysis performed by Allman et al. [21] pooled data from 24 viability studies as determined with 201TI-SPECT imaging, FDG-PET, or dobutamine echocardiography. A total of 3,088 cardiomyopathy patients with a mean LVEF of 32 ± 8 % were followed-up for 25 ± 10 months. In patients with defined myocardial viability, annual mortality rate was 16 % in medically treated patients while only 3.2 % in revascularized patients (Fig. 6.14). This again represents 79.6 % relative reduction in risk of death for revascularized patients (Fig. 6.14). Conversely, for patients without proof of viability, annual mortality was not significantly different between the groups with coronary revascularization as compared to those with medical treatment alone (7.7 % versus 6.2 %). Timely revascularization of viable and ischemic compromised myocardium commonly manifests in an improvement in left-ventricular function and prognosis of the patient, which, however, may be less favorable if coronary revascularization is delayed.
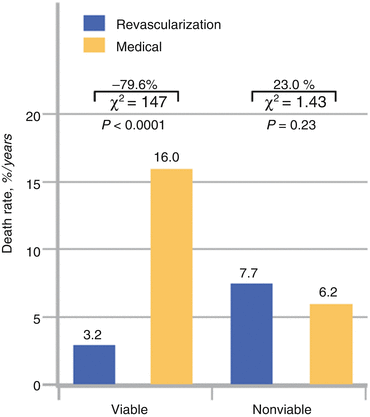
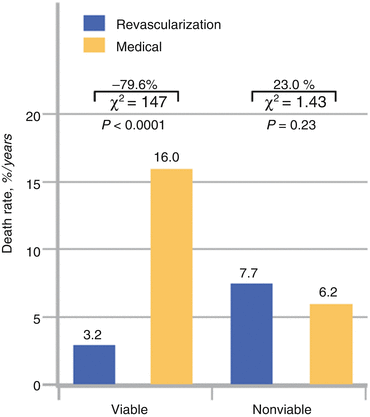
Fig. 6.14
Meta-analysis of pooled data from 24 viability studies. Death rates for patients with and without myocardial viability treated by revascularization or medical therapy (With kind permission from Ref. [21])
Addressing this issue, Tarakji et al. [49] analyzed the survival of 765 consecutive patients (age 64 ± 11 years) with advanced left-ventricular dysfunction (LVEF ≤35 %), who underwent FDG-PET viability study and coronary revascularization over a 5-year follow-up (1997–2002). Early intervention was defined as any cardiac intervention (surgical or percutaneous) within the first 6 months of the PET-FDG study. In the entire study population, 30 % patients (n = 230) underwent early intervention, 25 % (n = 188) had coronary artery bypass grafting, and 5 % (n = 42) had percutaneous revascularization, while 70 % (n = 535) were treated medically. Using 39 demographic and FDG-PET variables, 153 of the 230 patients were propensity matched with 153 patients without early coronary revascularization. And indeed, early revascularization was associated with a markedly lower risk of death (3-year mortality rate of 15 % versus 35 %, propensity adjusted hazard ratio 0.52 (95 % CI 0.33–0.81, p = 0.0004)) (Fig. 6.15). Interestingly, there was no apparent interaction between survival benefit associated with early cardiac intervention and the amount of viable tissue (ischemic and hibernating tissue) identified by PET.
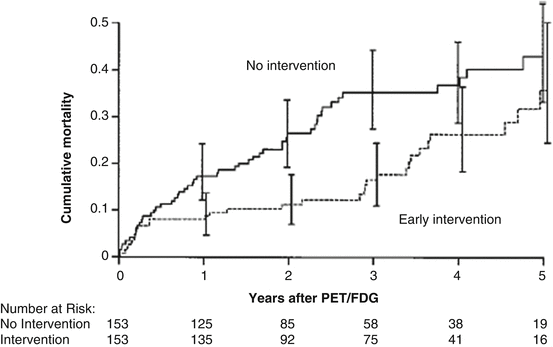
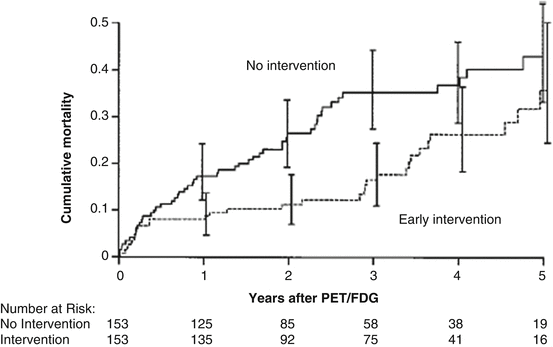
Fig. 6.15
Myocardial viability and early revascularization. Propensity-matched patients. Mortality curves of patients treated medically (no intervention) and patients undergoing early intervention (With kind permission from Ref. [49])
The exact mechanisms by which early coronary revascularization (<6 months) improved the clinical outcome remain uncertain but may be related to reductions in myocardial ischemia, improved myocardial function, regression of hypertrophy, beneficial effects on myocardial remodeling, and stabilization of arrhythmic substrate. Notably, there was no survival difference until 5–6 months of follow-up, which may be related to a relatively high mortality associated with periprocedural complications among those who underwent surgical intervention. These observations also concur with findings reported from Bax et al. [20] investigating whether a delayed coronary revascularization of viable myocardium in ischemic cardiomyopathy patients, as determined with on low-dose dobutamine stress echocardiography (DSE), may less favorably impact clinical outcome. On echocardiography, segmental wall motion was scored according to a 5-point scale: 1 = normal, 2 = mildly hypokinetic, 3 = severely hypokinetic, 4 = akinetic, and 5 = dyskinetic. Subsequently, severely dysfunctional segments (score 3 or more) were evaluated for the presence of viability. The four patterns that were observed in dysfunctional segments during DSE included (1) biphasic response (improvement of wall motion during low dose (5 and 10 μg/kg/min), followed by worsening of wall motion during high-dose dobutamine), (2) sustained improvement (improvement during low- and/or high-dose dobutamine without subsequent deterioration of wall motion), (3) worsening (immediate deterioration of wall motion during dobutamine infusion), and (4) no change (no change in wall motion during DSE). Dysfunctional segments were classified viable when they exhibited any of the patterns except for the “no change” pattern. A patient was considered to have substantial viability in the presence of four or more dysfunctional but viable segments (≥25 % of the left ventricle). Applying these criteria, 85 patients with ischemic cardiomyopathy and substantial viability (≥25 % of the left ventricle) on low-dose dobutamine stress echocardiography were identified as suitable candidates for surgical coronary revascularization. Based on the waiting time for revascularization, patients were divided into two groups: early (≤1 month) and late (>1 month) revascularization. LVEF was assessed before and 9–12 months after revascularization, while follow-up data were acquired up to 2 years after revascularization. As expected [21], early revascularization (20 ± 12 days) of viable myocardium in these patients conferred a high likelihood of an improvement in LVEF (from 28 ± 9 % to 40 ± 12 %) (Fig. 6.16a). Conversely, when coronary revascularization was relatively delayed (85 ± 47 days) in these patients, LVEF virtually remained unchanged (27 ± 10 % to 25 ± 7 %). Notably, delayed coronary revascularization in these patients was associated with a substantial higher mortality rate than in those patients referred for early revascularization of viable myocardium (20 % versus 5 %) (Fig. 6.16b) [20]. As regards the rehospitalization rate for heart failure, it was less in the early – than in the delayed revascularization group (10 % versus 24 %) – although it did not achieve statistical significance (Fig. 6.16b).
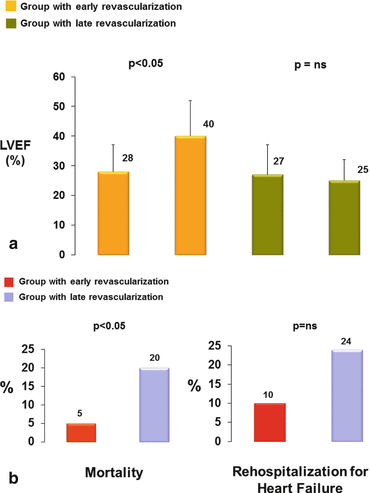
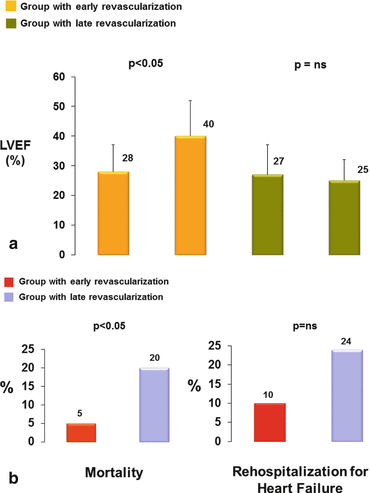
Fig. 6.16
Timing of revascularization, functional recovery, and clinical outcome. Impact of early and late revascularization on left-ventricular ejection fraction (LVEF) (a) and on mortality and rehospitalization rate, respectively (b) (With kind permission from Ref. [20])
Of course, someone may argue that these striking differences of recovery in left-ventricular function and clinical outcome between early and delayed coronary revascularization, respectively, may have been related to the selection criteria in DSE for defining myocardial viability according to the low-dose dobutamine stimulation of contractile function in viable areas. Beanlands et al. [22], therefore, investigated the use of FDG-PET in the assessment of myocardial viability for preoperative risk evaluation. Based on FDG-PET viability imaging, 46 patients with ischemic cardiomyopathy patients and a LVEF of ≤35 % were considered candidates for revascularization, while 35 of these were referred for surgical intervention. According to the median waiting time, patients were divided into two groups: an early group (<35 days; n = 18) and a late group (≥35 days; n = 17) comparable to the echocardiographic investigation [20]. Preoperative mortality rates were significantly higher in the late than in the early group of revascularization (4 of 17 [24 %] versus 0 of 18). During postoperative follow-up (17 ± 7 months), cardiac events were nonsignificantly higher 11 % (2/18) versus 7.8 % (1/13) in the early than in the late group. Conversely, LVEF increased significantly after early revascularization (24 ± 7 % to 29 ± 8 %, p < 0.001, baseline versus 3 months) but not in the late group (27 ± 5 % to 28 ± 6 %, p = ns). Preoperative FDG-PET-determined myocardial viability therefore may identify a high-risk group of patients who may benefit from early revascularization. As current available evidence suggests [20, 22, 49], a long waiting time for coronary revascularization of viable myocardium may be associated with a relatively high mortality rate, which favors an early revascularization of hibernating-stunning myocardium, if clinically feasible. Such observations may give rise to a dilemma for the cardiovascular surgeon, who may prefer to perform a CABG as late as possible in order to find optimal heart conditions associated with lower periprocedural and postoperative morbidity and mortality rate [50], which however may be at the expense of a lower gain in left-ventricular function, improved clinical outcome, and prognosis but needing further investigations.
In view of these clinical observations [20, 22, 49], the less impressive findings of the PARR-2 and STICH trial may not be so unexpected [42, 44]. In these two randomized viability and revascularization trials, patients with acute coronary syndrome (ACS) within 4 weeks or 3 months, respectively, were not included for study purpose. The targeted study populations comprised patients with stable and mostly known ischemic cardiomyopathy. For example, in both the PARR-2 and STICH trial, 80 % of heart failure patients had had previous myocardial infarction, while all patients presented different stages of angina symptoms or dyspnea according to the Canadian Cardiovascular Society Angina and NYHA classification, respectively. Most of these patients therefore can be assumed to have had already relatively long-standing ischemic heart disease with advanced cardiac remodeling and interstitial fibrosis [17, 18, 51], which may have prevented or impaired the recovery of left-ventricular function and improvement in cardiovascular prognosis after delayed rather than early CABG. Furthermore, in both the PARR-2 and STICH trial, no detailed information on the time interval between viability assessment and coronary revascularization is provided, respectively. As regards the STICH trial [44], ischemic cardiomyopathy patients were recruited for study analysis if a viability study had been done within 90 days. This again would suggest that also cardiomyopathy patients were included, in whom viability assessment was done 2–3 months before coronary revascularization. Thus, coronary revascularization was rather performed in a delayed fashion lowering the likelihood of functional recovery of viable myocardium and improvement in clinical outcome in these cardiomyopathy patients. Considering this, it may be no surprise that both the PARR-2 and STICH trial [42, 44] did not report a significant reduction in cardiac events in patients with ischemic left-ventricular dysfunction, when viability assessment with various imaging techniques was compared to standard medical care of heart failure, as coronary revascularization was delayed. Thus, the failure of viability assessment to identify those patients with a survival benefit from interventional or surgical revascularization procedures as compared to medical treatment of heart failure in two randomized trials [42, 44] is likely to be related, at least in part, to a relatively delayed coronary revascularization in ischemic cardiomyopathy patients as several investigations suggest [20, 49, 52].
6.4 Extent and Severity of Myocardial Ischemia
As regards the STICH trial, the extent of viable but ischemic jeopardized dysfunctional (hibernating) myocardium is not known [44]. Thus, it is equally possible that a certain percentage of patients with prove of ≥11 viable myocardial segments did not have or have only mildly ischemic compromised myocardium at rest and stress (fixed perfusion defect), so-called “hibernating-stunning” myocardium as evidenced by “mismatch” findings between myocardial perfusion and viability assessment with FDG-PET (Fig. 6.6) [10]. Rest and/or stress-induced myocardial ischemia may be prevented or significantly diminished by the induction of collaterals through a myocardial hypoxic stimulus in patients with chronic ischemic cardiomyopathy, which strives to balance reduced flows during times of increased metabolic demand in myocardial regions subtended by high-grade epicardial narrowing or even occluded vessels [53–55]. It also must be considered that any medical therapy which aims and improves the vasodilator capacity of the coronary circulation, such as HMG-CoA reductase or angiotensin-converting enzyme inhibitors, may in fact prevent or reduce clinically manifest myocardial ischemia in patients with CAD [56]. In some instances, even the absence of myocardial ischemia in patients having suffered non-transmural myocardial infarction in a region subtended to an occluded artery may be noted. The absence of significant amount of a resting perfusion defect or myocardial ischemia in the infarcted territory due to collateral flow with residual viability subtended to an occluded artery might explain, in part, why the interventional reopening of the occluded infarct-related artery did not lead to a significant reduction in the occurrence of death, reinfarction, or hospitalization for class IV heart failure over a 3-year but also over 6-year mean follow-up compared with optimal medical therapy alone, respectively [57, 58]. Thus, it appears that in these high-risk patients with dysfunctional but viable myocardium, the extent and amount of resting perfusion defect or ischemia is critical for the prediction of recovery in left-ventricular function and improvement in clinical outcome after revascularization. This important aspect is implied in the “match” and “mismatch” concept between perfusion and viability assessment with PET as outlined before [33]. While a “match” finding signifies a concordant reduction in perfusion and metabolism without ischemic component, a “mismatch” observations denotes a more pronounced reduction in perfusion or resting ischemia in preserved areas of viable myocardium, which reflects hibernating-stunning myocardium. Only ischemic jeopardized viable or hibernating-stunning myocardium will truly benefit from coronary revascularization both functionally and prognostically (Fig. 6.17) [6, 10, 20, 21, 39, 41, 59].
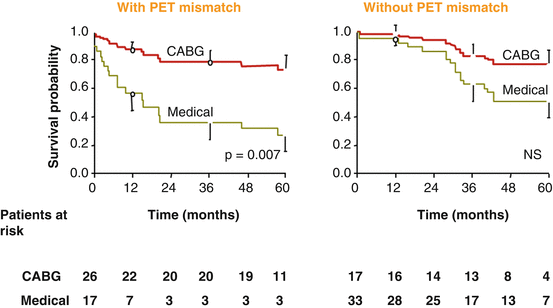
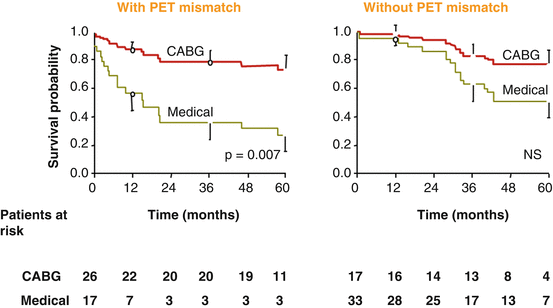
Fig. 6.17
Survival curves of ischemic cardiomyopathy patients undergoing coronary revascularization. Five-year survival data for patients with ischemic cardiomyopathy according to the proof of blood flow-metabolism mismatches and matches and according to treatment option, respectively. Note the high cardiac mortality in patients with blood flow-metabolism mismatches (left panel) on medical treatment and the significantly improved 5-year survival in a comparable group of patients submitted to surgical revascularization (With kind permission from Ref. [38])
This consideration also agrees with previous investigation of Beanlands et al. [60] using 201TI-SPECT for the assessment of perfusion and viability in 23 patients with occluded arteries after myocardial infarction. Also here, the identification and characterization of the extent of resting perfusion defects in dysfunctional but viable myocardium with 201TI myocardial scintigraphy proved to be useful to identify those patients who will most benefit from reopening of the occluded vessel [60]. As in the STICH trial only those patients without acute coronary syndrome within the last 3 months were considered for coronary revascularization, it is equally possible that a certain number of patients with demonstrated myocardial viability on SPECT images or low-dose dobutamine echocardiography did not have advanced ischemic state at rest due to the development of collateral flow and medically improved myocardial flow reserve. Coronary revascularization in these patients without or with only mildly ischemic jeopardized myocardium, therefore, may not have conferred the expected benefit in improvement of left-ventricular function and prognosis as someone might have expected.
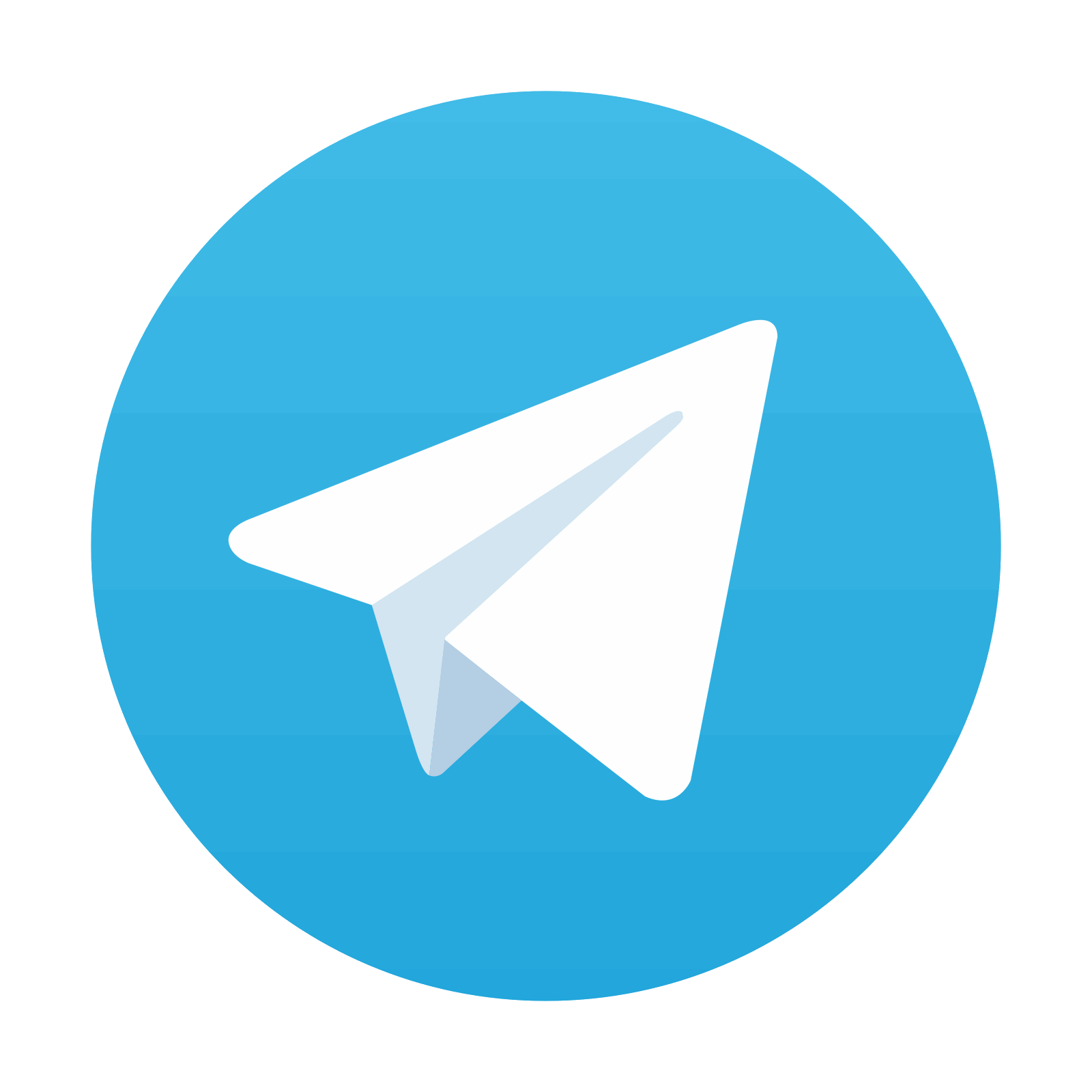
Stay updated, free articles. Join our Telegram channel

Full access? Get Clinical Tree
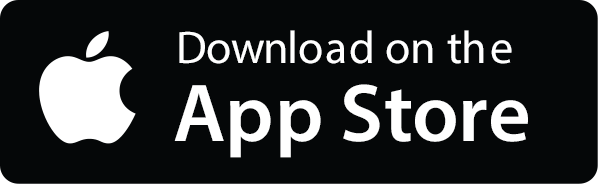
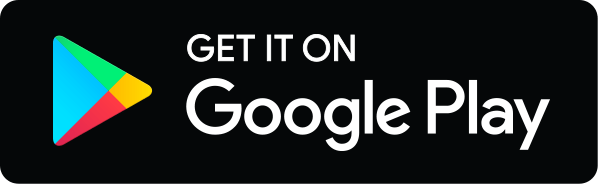