Vessel
Day 1 (mean weight: 2.2 kg)
Three years (mean weight: 20 kg)
Weight-adapted growth per kg weight gain
Ascendingaorta (mm)
8.4
14
0.43
Aortic arch(mm)
6.8
14.4
NA
Aortic isthmus (mm)
4.7
8.3
NA
Main pulmonary artery (mm)
7.5
14
0.5
Right pulmonary artery (mm)
4.3
8.6
0.33
Left pulmonary artery (mm)
4.2
8.8
0.35
23.2.2 Heart Rate and Premedication for Coronary Artery Imaging
The resting heart rates of infants, children, and adolescents are higher than those of adults, and there is more heart rate variability with respiratory changes (sinus arrhythmia, Table 23.2). The image acquisition window of current-generation CT scanners is as low as 75 ms, allowing coronary imaging even at higher heart rates. Coronary artery imaging is recommended in pediatric patients for evaluation of potentially malignant coronary artery anomalies, after surgical manipulation or reimplantation, and for evaluation of stenosis or coronary pathology in patients with a history of Kawasaki disease, systemic inflammatory disease, or Williams Beuren syndrome (aortic or pulmonary artery stenosis).
Table 23.2
Age-related heart rates in children
Age (years) | Heart rate (beats per min) |
---|---|
<1 | 110–160 |
1–2 | 100–150 |
2–5 | 95–140 |
5–12 | 80–120 |
>12 | 60–100 |
Beta blockade and other medication protocols have been used safely in healthy children to decrease the heart rate and improve the quality of coronary imaging. For patients with chronic heart failure or in those who are critically ill, beta blockade may be clinically contraindicated (Chap. 6). The use of premedication must be tailored to individual patients and their current clinical status.
23.2.3 Respiratory and Motion Artifacts
The newest-generation volumetric or high-pitch scanners can acquire the scan range of a pediatric thorax in one heart beat, or a fraction of a second, which has reduced anesthesia and intubation needs in young patients. Many scans can be performed during free breathing without loss of image quality due to motion artifacts. For some older-generation scanners with image acquisition over several seconds, intubation or sedation may be required to obtain a scan without respiratory motion artifacts. The use of anesthesia and sedation will be dependent on the scanner available at each institution and the amount of detail required from the CT scan.
23.2.4 Intravenous Access and Contrast Agent Injection
Many types of peripheral access can be used for cardiac CT scans in patients with congenital anomalies including peripherally inserted central catheter (PICC) lines, umbilical venous lines, peripheral intravenous (IV) lines, and central venous lines. In the presence of intracardiac shunting, elimination of air bubbles in the injection is particularly important to avoid potential arterial emboli. Low flow rates are recommended when using a 24- or 22-gauge IV access. Careful observation of the IV site during a saline injection is recommended to confirm patency before the contrast agent is injected. Safe power injection through 24–18 gauge IV lines has been reported in pediatric patients. The contrast injection rate through a 24-gauge IV line is typically 0.5–1.0 ml/s and up to 1.5–2 ml/s through a 22-gauge IV access (Table 23.3).
Table 23.3
Recommended contrast agent injection protocols in children
Age (years) | Preparation |
---|---|
<1 | 24–22 G venous line as proximal as possible |
0.5–1.0 ml/s for 24 G (<100 psi) | |
1–1.5 ml/s for 22 G | |
1–2 ml/kg total contrast volume | |
1–2 | 22 G venous line as proximal as possible |
1–2 ml/s, psi < 150 | |
1–2 ml/kg total contrast volume | |
>2–5 | 22 G venous line |
2 ml/s | |
1–2 ml/kg total contrast volume | |
>5 | 18–20 G venous line |
2–5 ml/s | |
1–2 ml/kg total contrast volume |
23.2.5 IV Line Placement and Injection Protocols
The total contrast agent volume used for pediatric CT angiography is typically 1–2 ml/kg. For pulmonary or arterial angiography without intracardiac mixing, the IV line may be placed in any extremity and an automatic trigger used for image acquisition. A biphasic injection protocol can be used for simultaneous opacification of right- and left-sided cardiac structures.
23.2.6 Scanning Protocol
Every pediatric CT scan must be adjusted to deliver the lowest radiation dose to obtain a diagnostic image according to the “as low as reasonably achievable” (ALARA) principle. Familiarity with the doses delivered with different scan modes is necessary and allows choosing the scan mode with the least risk for each specific indication. CT scanner output should be adjusted to the patient’s size. Using dose reduction techniques allows mSv or sub-mSv imaging for many pediatric indications, including coronary artery imaging.
For anatomic scans, high-pitch or the volumetric scan mode may be used on latest-generation scanners. On older-generation scanners, anatomic scanning is most commonly performed without ECG triggering.
Coronary artery and functional imaging require ECG triggering on all scanner platforms. Several different scan modes are available for coronary artery imaging. The lowest dose technique deemed adequate for the individual patient’s heart rate should be used.
For pediatric cardiovascular CT, no general recommendation for kV and mAs settings can be provided. For dose comparison, the dose length product (DLP) should be used. In our experience, a DLP as low as 4–8 mGy*cm (70 or 80 kV) is sufficient for imaging a baby weighing 3–4 kg. Only a minor increase in DLP is necessary with increasing weight, as the thoracic diameter stays rather constant during the first years of life. The most common indication for CT angiography in the neonatal CHD population is for evaluation of aortic or pulmonary artery pathology or for characterization of complex systemic and pulmonary venous anomalies prior to intervention. Many postoperative patients will have metallic implants and devices that degrade image quality of MRI. CT is also valuable for coronary artery assessment in patients with a history of Kawasaki disease aneurysm and for assessment of bileaflet mechanical valve function.
23.3 Atrial and Ventricular Septal Defects
The most common congenital anomalies are atrial (7%) and ventricular septal (31%) wall defects (Fig. 23.1). However, the role of CT in patients who are referred for preoperative evaluation of ventricular or atrial septal wall defects is limited. Echocardiography and MRI are the main diagnostic modalities for these lesions.
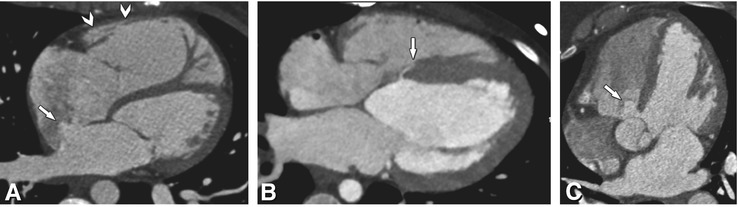
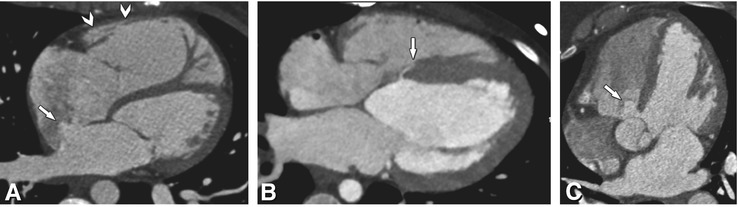
Fig. 23.1
Atrial and ventricular septal wall defects in three patients. Panel A shows a centrally located secundum atrial septal defect with contrast shunting from the left to right atrium (arrow). Note the right ventricular enlargement in this patient (arrowheads). The patient underwent successful device closure of the defect. Panel B shows a tiny basal muscular ventricular septal defect (arrow). This defect is located just inferior to the perimembranous area; most defects will be located more apically. Note that there was no relevant contrast jet from the left to the right ventricle and the lesion was considered hemodynamically insignificant. Panel C shows a large perimembranous ventricular defect located adjacent to the tricuspid valve just inferior to the aortic valve. Note the resulting positive contrast jet from the left to right ventricle in this patient (arrow)
23.4 Aortic Arch
Congenital anomalies of the aorta are one of the most frequent cardiovascular malformations. They include a wide spectrum of diseases (List 23.1) ranging from patent ductus arteriosus (Fig. 23.2, 5–10% of all CHD) to aortic coarctation (8–10%, Fig. 23.3). The initial diagnosis is usually established by echocardiography. Blood pressure difference between the upper and lower extremity is used to determine the pressure gradient unless there is an aberrant subclavian artery that does not allow a pre-coarctation pressure estimate. Doppler echocardiography is helpful in these cases, and catheterization is rarely required prior to surgical intervention for aortic coarctation at most centers. Noncritical stenosis or critical stenosis with well-developed collateral flow to the descending aorta is often detected in grown-ups who present with arterial hypertension and have blood pressure differences between arms and legs. Clinical indications for follow-up examinations are re-coarctation (<3%, higher when operated on in infancy) and postoperative aneurysms (24% after patch angioplasty) or dissection (5–50% after patch angioplasty, Fig. 23.4). Because it lacks radiation exposure and allows functional assessment (e.g., flow quantification), MRI is the preferred test in suspected coarctation (Fig. 23.5). As patients grow older, MRI may gain an increasing role as the acoustic window for echocardiography becomes poorer with age. CT has an indication in the follow-up of patients with coarctation who have stents, as the intraluminal visualization of stents is excellent (Fig. 23.6). In complex aortic arch abnormalities (i.e., double aortic arch), surgeons prefer to have a three-dimensional display of the anatomic relationship between the trachea and the vascular structures. This information can be nicely provided by CT, even in critically ill children. A potential vascular ring associated with a right aortic arch, an aberrant left subclavian artery, and a left-sided ductal ligamentum are common and may be detected incidentally or cause pulmonary pathology. Evaluation of the airways relative to the vessels can be crucial in determining the clinical significance of the finding.
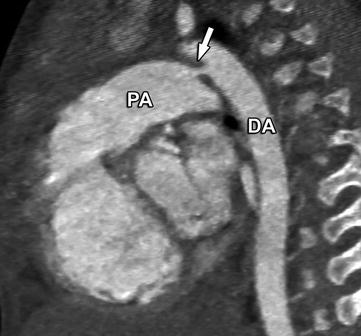
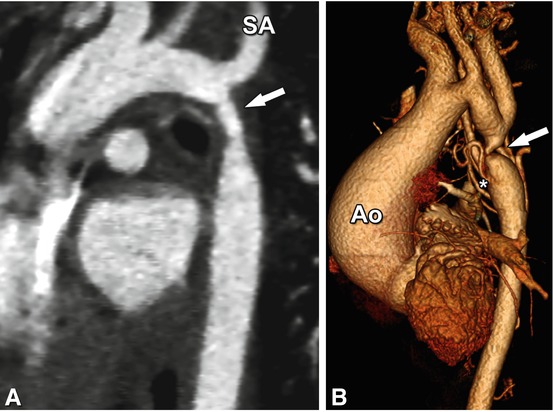
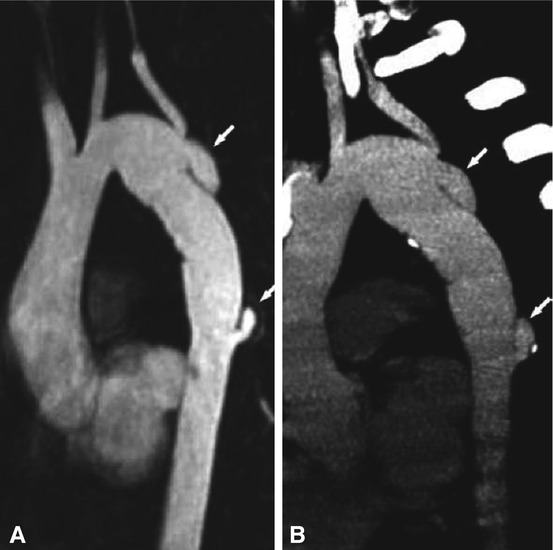
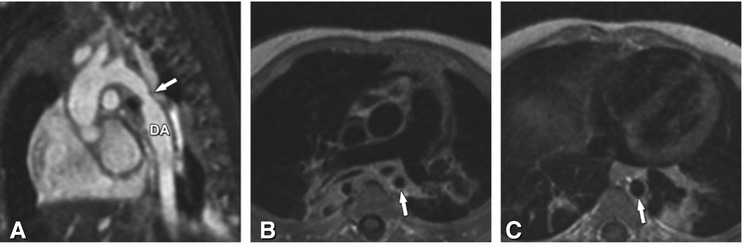
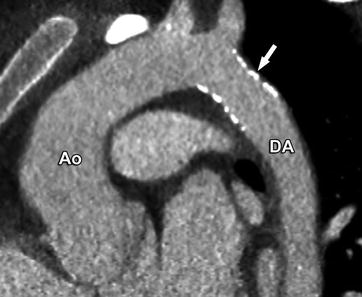
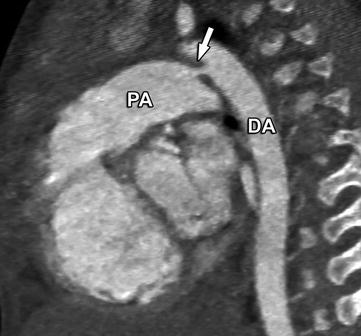
Fig. 23.2
Tiny ductus arteriosus (arrow) between the proximal descending aorta (DA) and the distal pulmonary artery (PA). This was an incidental finding on a CT performed for evaluation of the coronary arteries
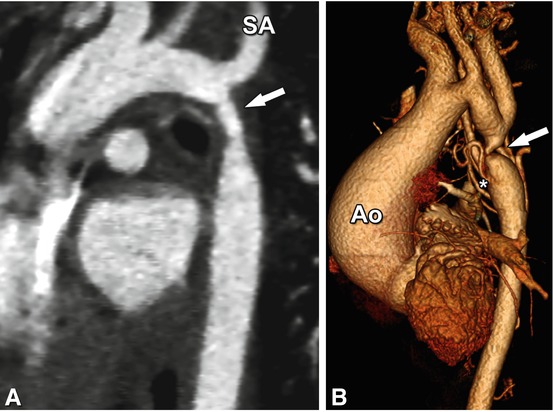
Fig. 23.3
Aortic coarctation in two patients. Panel A is a double-oblique image from a prospectively ECG-triggered CT scan showing coarctation in a 2-year-old patient (arrow) just distal to the takeoff of the left subclavian artery (SA). The high-pitch CT scan was performed during free breathing with an acquisition time of 0.25 s and a dose-length product of 6 Gy.cm. The patient underwent subsequent repair of coarctation with an end-to end anastomosis. Panel B shows a three-dimensional reconstruction of a CT scan performed for evaluation of severe aortic coarctation (arrow) in an 11-year-old patient who presented for evaluation of a murmur. His ascending aorta (Ao) was dilated and the murmur was secondary to aortic insufficiency. He was noted to be hypertensive with a blood pressure gradient of 30 mmHg between the right arm and leg. Note the collateral vessels supplying the descending aorta (asterisk). He underwent surgical repair of aortic coarctation and has since been followed clinically for aortic insufficiency and aortic root dilation
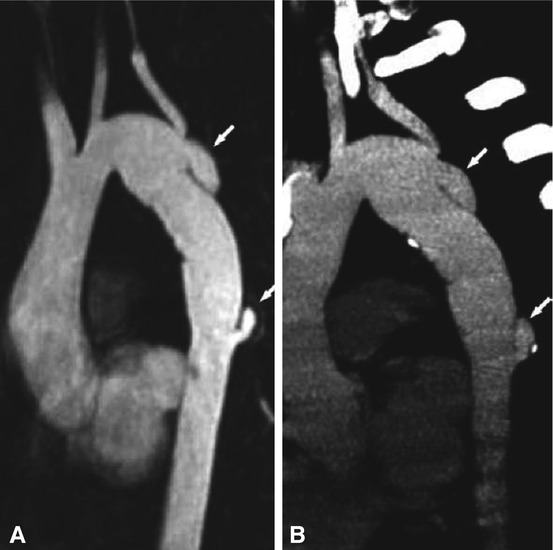
Fig. 23.4
Dissection as a complication after aortic arch operation in a 24-year-old female patient with congenital hypoplasia of the distal aortic arch and patch angioplasty at the age of 2 years. Eight years later a restenosis was diagnosed and a vascular prosthesis (16-mm Hemashield) was implanted. Regular follow-up was performed by MRI. Panel A is an MR angiography after intravenous contrast agent administration (10-mm double-oblique maximum intensity projection) that shows new dissections at the proximal and distal anastomosis of the prosthesis (arrows). For further surgical planning a nongated CT was performed (Panel B, 5-mm maximum intensity projection), which confirmed the MRI findings
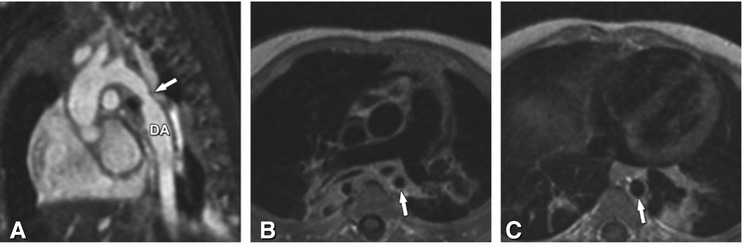
Fig. 23.5
Coarctation of the aorta in a 5-month-old male baby. Panel A is an ECG- and respiratory-gated steady-state free precession three-dimensional sequence (MR angiography) without contrast agent administration and demonstrates focal narrowing (arrow) of the descending aorta (DA). Panel B is a spin-echo MRI sequence with dark-blood pulse preparation, which shows the narrowing of the aorta in axial orientation (arrow), while Panel C shows the normal lumen of the descending aorta (arrow) on the same sequence at the level of the diaphragm. Note that the consolidation in the left lower lung seen on Panel C is due to a prior pneumonia in this patient
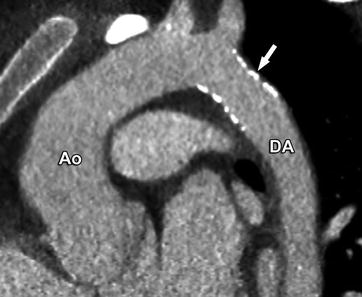
Fig. 23.6
Follow-up CT in an 18-year-old patient after interventions for aortic coarctation. The patient underwent left subclavian flap repair of aortic coarctation as an infant and later required balloon angioplasty of recurrent coarctation with stent placement (arrow). The CT was performed with prospective ECG triggering using 80 kV and a scan range limited to the area of interest. Note the absence of a left subclavian artery from the transverse aortic arch due to the initial repair and excellent visualization of the stent and aortic wall. Ao aorta, DA descending aorta
List 23.1. Aortic anomalies and defects
2.
Anomalies of the ascending aorta and of the aortic arch
(b)
Aortic rings
(i)
Double aortic arch
(ii)
Right-sided aortic arch with aberrant left subclavian artery and left-sided ductus arteriosus
(iii)
Left-sided aortic arch with Lusoria artery
(c)
Interrupted aortic arch
(d)
Truncus arteriosus
3.
4.
Rare anomalies, e.g., aneurysm of the ductus arteriosus
6.
Marfan syndrome (development of aortic root dilatation)
7.
Ulrich-Turner syndrome (associated with aortic coarctation)
23.4.1 Persistent Ductus Arteriosus
During fetal life the ductus arteriosus connects the main pulmonary artery near its bifurcation to the aorta just beyond the origin of the left subclavian artery. The ductus arteriosus usually closes functionally soon after birth through muscular contractions. A persistent ductus arteriosus (PDA) is encountered in approximately 7% of all congenital heart defects. Echocardiography is the modality of choice for assessment of the ductus arteriosus in infants. CT is only performed if a complex malformation is suspected (Figs. 23.2 and 23.7).
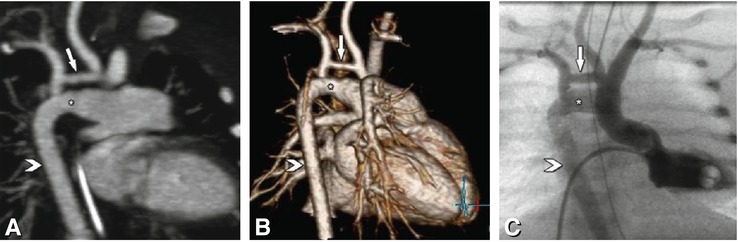
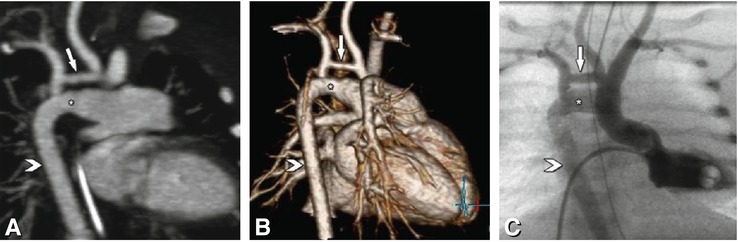
Fig. 23.7
Hypoplastic aortic arch (arrow) and large persistent ductus arteriosus Botalli (asterisk) in a 2-day-old child prenatally diagnosed with a cardiac malformation. On a double oblique maximum intensity projection (Panel A) the large persistent ductus arteriosus and the hypoplastic aortic arch (arrow) are shown. The persistent ductus arteriosus connects the main pulmonary artery and the descending aorta (arrowhead), which is normal. For operative planning the CT images were additionally presented to the surgeon as three-dimensional volume renderings (Panel B). Panel C presents the corresponding image from left ventricular catheterization
23.4.2 Sequestration
Sequestration is a rare anomaly of the tracheobronchial tree. It has an estimated incidence of 0.15–1.7% in the general population. Sequestrations are classified as intralobar or extralobar. The nonfunctioning lung tissue lacks communication with the tracheobronchial tree and receives its supply from the arterial circulation. Intralobar sequestrations are the most common. The blood supply usually comes from the descending thoracic aorta, but in about 20% of cases, it comes from the upper abdominal aorta, celiac artery, or splenic artery. All pulmonary sequestrations should be treated surgically/interventionally because the high blood flow through the lesion can cause heart failure. Surgical procedures include ligation of the afferent vessels and/or resection of the pulmonary malformation.
In pulmonary sequestrations CT is superior to most other techniques, providing excellent information on both the vessels supporting the malformation (arterial and venous) and the structure of the lung parenchyma inside the malformation (Fig. 23.8). The spatial resolution of MR angiography may be too low to achieve adequate visualization of all vascular structures. Also, visualization of the lung parenchyma is difficult using MRI.
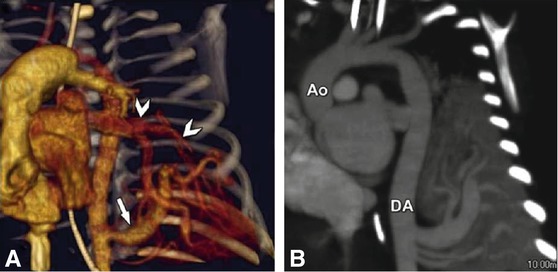
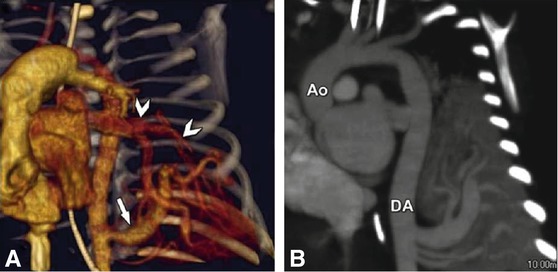
Fig. 23.8
Lung sequestration in a 15-day-old female baby with a prenatally diagnosed anomaly of the left lung. ECG-gated CT was performed using 7 ml contrast agent with 250 mg I/ml at an injection rate of 0.3 ml/s. Panel A shows a volume rendering of the arterial supply (three vessels) of the sequestration from the descending aorta (arrow). Venous drainage is to the left atrium (arrowheads). Panel B is a double-oblique maximum intensity projection along the ascending aorta (Ao) and descending aorta (DA) showing the three arteries supplying the sequestration from the DA. Due to the large size of the arterial supply the patient went to surgery for clipping of the arteries
23.5 Pulmonary Artery Pathology
Echocardiography is excellent for definition of the pulmonary valve and proximal pulmonary arteries, but is inadequate for distal pulmonary artery evaluation. CTA is well suited for detection of an absent pulmonary artery, a pulmonary artery arising anomalously from the aorta (Fig. 23.9) or aortopulmonary collateral arteries, and peripheral stenosis associated with certain genetic syndromes (Fig. 23.10) and for visualization of both the aortic and pulmonary arterial portions of the ductus arteriosus.
