Stephen J. Nicholls and Tim Crowe (eds.)Contemporary CardiologyImaging Coronary Atherosclerosis201410.1007/978-1-4939-0572-0_11
© Springer Science+Business Media New York 2014
11. Coronary Calcification: Roles in Risk Prediction and Monitoring Therapies
(1)
Department of Medicine/Cardiology, Los Angeles Biomedical Research Institute at Harbor-UCLA Medical Center, 1124 West Carson Street, Torrance, CA 90502, USA
Abstract
Cardiovascular disease is the leading cause of mortality in the USA and worldwide. Traditional risk factor assessment may not fully account for the coronary risk and underestimate the prediction of risk even in patients with established risk factors for atherosclerosis. Coronary artery calcium (CAC) represents calcified atherosclerosis in the coronary arteries. It has been shown to be the strongest predictor of adverse future cardiovascular events and provides incremental information to the traditional risk factors. It has been incorporated into the ACC/AHA guidelines for risk assessment of asymptomatic intermediate risk (10–20 % 10-year risk), low to intermediate risk (6–10 % 10-year risk), and diabetic individuals. CAC can be used to reclassify individuals based on traditional risk factor assessment and provide opportunity to better strategize the therapeutic options for these subjects. Baseline CAC represents a single point in time on atherosclerosis time curve whereas progression of CAC over time presents the slope of that curve. CAC progression has also been identified as a risk for future cardiovascular events. However, the role of CAC progression as a primary end point for following up statin treatment effect may not be appropriate due to micro-calcifications caused by the statin treatments. There are certain non-statin treatments such as nifedipine, aged garlic extract, and unopposed estrogen replacement that have shown promising results in reducing the progression of CAC.
Introduction
Coronary artery calcium (CAC) represents calcified atherosclerosis in the coronary arteries which is believed to represent a dynamic marker of coronary risk assessment subject to influence by environmental factors and therapeutic interventions. The traditional cardiovascular risk assessment may underestimate the prediction of cardiovascular disease (CVD) risk and many individuals still suffer events in the absence of established risk factors for atherosclerosis [1]. CAC has been shown to be the strongest independent predictor of future adverse cardiovascular events and also provides incremental information to the traditional cardiovascular risk factors assessment. It can be used to risk stratify asymptomatic individuals, improve the risk prediction provided by Framingham risk score (FRS), and follow the effect of various therapeutic interventions on coronary atherosclerosis.
CAC as a Risk Stratification Tool
There is tremendous evidence available that supports the role of CAC as the best risk stratifier for asymptomatic individuals. Based on literature evidence, CAC has been incorporated into the ACC/AHA guidelines for screening of asymptomatic individuals for CVD. CAC has been advocated as a screening tool for risk assessment of asymptomatic adults at intermediate risk (10–20 % 10-year risk), low to intermediate risk (6–10 % 10-year risk), and patients with diabetes [2]. CAC has been persistently shown to have superior independent and incremental predictor of CVD compared with traditional risk factors [3–13]. The Multi-Ethnic Study of Atherosclerosis (MESA) sponsored by the National Heart, Lung, and Blood Institute was a prospective population based study of four different ethnic groups (whites, Hispanics, Asians, and African Americans) which provided a detailed insight into the role of CAC in risk assessment. Detrano et al. [4] showed that adjusted risk for coronary events increased by a factor of 7.73 among subjects with CAC scores between 101 and 300 and by a factor of 9.67 with CAC score above 300 when compared with CAC score 0 in 6,772 MESA participants. Across four ethnic groups, a doubling of CAC increased the risk of major coronary event by 15–35 % and the risk of any coronary event by 18–39 %. CAC provided an incremental value for prediction of major coronary events when added to the standard risk factors (0.79 vs. 0.83 area under the curve for risk factors alone vs. risk factors plus CAC, p = 0.006). Budoff et al. [5] showed CAC to be an independent predictor of mortality after controlling for age, gender, ethnicity, and cardiac risk factors (model chi-square = 2,017, p < 0.0001), in a registry of 25,253 asymptomatic individuals. CAC was shown to have significant incremental value compared with risk factors resulting in a higher concordance index (0.61 vs. 0.81; p < 0.0001). Risk-adjusted relative risk ratios for CAC scores 11–100, 101–299, 300–399, 400–699, 700–999, and >1,000 were 2.2-, 4.5-, 6.4-, 9.2-, 10.4-, and 12.5-fold when compared with CAC score 0, respectively (p < 0.0001). Greenland et al. [6] performed a prospective observational population-based study on 1,461 asymptomatic adults with coronary risk factors who underwent CAC score and followed prospectively for up to 8.5 years for nonfatal myocardial infarctions or coronary heart disease (CHD) deaths. Compared with CAC score of zero, a CAC score of more than 300 was significant predictor (hazard ratio HR, 3.9; 95 % confidence interval (CI) 2.1–7.3; p < 0.001) of nonfatal myocardial infarctions or CHD deaths. Across FRS categories (0–9 %, 10–15 %, 16–20 %, and ≥21 %), CACS was predictive of risk among patients with an FRS higher than 10 % (p < 0.001) but not with an FRS less than 10 %. FRS plus CAC resulted in a significantly greater mean area under the curve for the receiver operating characteristics curve compared with FRS alone (0.68 vs. 0.63; p < 0.001). Similarly, data from ACC/AHA clinical consensus document comprising 27,622 asymptomatic subjects showed that patients with CAC scores between 400–1,000 and >1,000 had 3–5 year rates of cardiac deaths or myocardial infarctions of 4.6 and 7.1 % resulting in a relative risk ratios of 7.2 (95 % CI: 5.2–9.9; p < 0.001) and 10.8 (95 % CI: 4.2–27.7; p < 0.0001) compared with patients with CAC score 0 [14].
Pencina et al. [15] introduced the concept of net reclassification improvement (NRI) which measures the extent to which people with and without events are appropriately reclassified into clinically accepted higher or lower risk categories with the addition of a new marker. CAC has been shown to reclassify the risk assessment based on FRS and National Cholesterol Education Adult Treatment Panel (ATP) III guidelines into either higher or lower risk categories based on CAC score information [13, 16–18]. Erbel et al. performed a prospective population-based study on 4,129 subjects without overt CAD at baseline and followed them for 5 years. Risk reclassification into to either low-risk category or high-risk category using CAC <100 and CAC ≥400 yielded an NRI of 21.7 % (p = 0.0002) and 30.6 % (p < 0.0001) for the FRS, respectively. Integrated discrimination improvement using FRS variables and CAC was 1.52 % (p < 0.0001). There was significant improvement in the area under the curve from 0.681 to 0.749 (p < 0.003) and from 0.653 to 0.755 (p = 0.0001) by adding CAC scores to the FRS and National Cholesterol Education Panel ATP III categories, respectively. Polonsky et al. [16] showed that a model involving CAC in addition to traditional risk factors (age, gender, tobacco use, systolic blood pressure, antihypertensive medication use, total and high-density lipoprotein cholesterol, and race/ethnicity) resulted in significant improvement in risk prediction compared to traditional risk factors alone (NRI = 0.25, 95 % CI: 10.16–0.34, p < 0.001). A model involving CAC classified 77 % of the cohort into lowest or highest risk categories compared with 69 % without CAC.
Blaha et al. [19] used the entry criteria for JUPITER trial [20] (low-density lipoprotein (LDL) <130 mg/dL) and highly sensitive C-reactive protein (hsCRP) concentration ≥2 mg/L in MESA population (950 subjects) to compare the risk prediction value of CAC across ranges of low- and high hsCRP values and calculated 5-year number needed to treat by applying the benefit recorded in JUPITER trial to the events within each CAC strata. For CHD, the predicted 5-year number needed to treat were 549 for CAC score 0, 94 for scores 1–100, and 24 for scores greater than 100. For CVD, the number needed to treat was 124, 54, and 19, respectively. hsCRP was not associated with either disease after multivariable adjustment. For rosuvastatin, the number needed to treat to prevent the occurrence of adverse cardiovascular event for an average 5-year treatment period is 25 [20, 21]. CAC can also be used to estimate an arterial age in adults [22]. Estimated arterial age is obtained as a simple linear function of log-transformed CAC. Framingham risk calculated using arterial age is more predictive of short-term incident coronary events than Framingham risk based on observed age (area under the ROC curve 0.75 for Framingham risk based on observed age, 0.79 using arterial age, p = 0.006).
The risk for future adverse cardiovascular events increases with increasing CAC scores; however, the absence of CAC presents a very unique situation which is associated with very low-risk status for the individual (10 year event rate of ~1 %) [11, 12]. Blaha et al. [11] showed that there were 104 deaths (0.52 % event rate) among patients with CAC score zero (19,898 patients) compared with patients with CAC score >10 (3.96 % event rate). The annualized all-cause mortality rates for CAC score zero and CAC >10 were 0.87 and 7.48 deaths/1,000 person-years. CAC score of zero separates the very low-risk group from subjects that are at relatively higher risk for future adverse cardiovascular events whether the amount of CAC is minimal or significant. Budoff et al. [23] performed an analysis in a multiethnic population of MESA study where they showed that the CAC score of zero (3,415 individuals) was associated with 17 CHD events (0.5 %) compared with 11 in those with CAC of 1–10 (2 % event rate). In age- and gender-adjusted analysis, the presence of minimal CAC (1–10) was associated with an estimated threefold higher risk of a hard CHD event (HR: 3.23; 95 % CI: 1.17–8.95) which remained robust even after adjustment for traditional cardiovascular risk factors and carotid intima-media thickness. Raggi et al. [24] followed 632 patients for a mean of 32 ± 7 months for the development of acute myocardial infarction or cardiac death. The annualized event rate in patients with CAC score 0 was 0.11 % compared with 4.8 % in patients with CAC score >400. Greenland et al. [14] showed that asymptomatic patients with CAC score zero had a low event rate (0.4 %) over the 3–5 years period in ACC/AHA clinical consensus document. Similarly, in MESA study, subjects with CAC score 0 had an exceptionally low CVD event rate in an ethnically diverse subject population [4]. Due to extremely low event rate in subjects with CAC score of zero, it is being used to exclude CAD as the cause of chest pain in low-risk patients in NICE clinical guidelines in Britain [25].
Traditional risk factor assessment has been shown to underestimate coronary risk in certain population groups especially women and young adults [26–28]. As a result, many of these patients may not receive aggressive medical management for their coronary risk factors. CAC may help to refine the risk stratification and treatment strategies of these patient populations who are otherwise categorized as low risk based on FRS categorization. Among 3,601 women in MESA study [29], 90 % of women were in the low-risk category according to FRS categorization after excluding women with diabetes and older than 79 years of age. In this group, >30 % of women were found to have CAC score >0 whereas the prevalence of significant CAC (≥300) was 4 %. The hazard ratio of CHD in women with CAC score >0 was 6.5 (95 % CI: 2.6–16.4) compared with women with CAC score of zero. Berry et al. studied incidence and progression of subclinical atherosclerosis in young adults ≤50 years of age in subjects enrolled in Coronary Artery Risk Development in Young Adults (CARDIA) and MESA study [30]. They divided the patients into groups based on their 10 year and lifelong risk for CVD into low 10-year (<10 %)/low lifetime risk (<39 %), low 10-year (<10 %)/high lifetime risk (≥39 %), and high 10-year risk (≥10 %) or diagnosed diabetes. They found that individuals with low 10-year but high lifetime risk have a greater subclinical atherosclerotic burden on carotid IMT and CAC and greater incidence of atherosclerotic progression compared to individuals with low 10-year and low lifetime risk. In light of these and other data, current practice guidelines suggest physicians consider current risk factor burden within the context of long-term or lifetime risk for CVD [31–33].
CAC has also been shown to have superior predictive value compared to other markers of coronary risk assessment such as carotid intima-media thickness and C-reactive protein [3, 34]. MESA study showed that the hazard of CVD increased 2.1-fold for log-transformed CAC vs. 1.3-fold for maximum IMT for each standard deviation increment of log-transformed CAC and maximum IMT. An ROC analysis showed CAC to be a better predictor of incident CVD than IMT [34]. Similarly, CAC has been shown to perform better than C-reactive protein and highly correlate to future CVD in a population-based study of 4,903 asymptomatic individuals [3].
CAC is a noninvasive measure of atherosclerosis; however, its use is associated with certain radiation exposure and medical cost. Rozanski et al. [35] studied the direct impact of CAC on future CAD risk and downstream medical cost compared to that of the conventional medical practice. They performed a prospective randomized trial on 2,137 volunteers that either undergo CAC scanning or did not undergo CAC scanning before risk factor counseling. The primary end point of the trial was 4-year change in CAD risk factors and FRS. Compared with no scan group, the scan group showed a net favorable change in systolic blood pressure (p = 0.02), LDL cholesterol (p = 0.04), waist circumference for those with increased abdominal girth (p = 0.01), and tendency to weight loss among overweight subjects (p = 0.07). FRS remained static in the scan group compared with no scan group (0.002 ± 4.9 vs. 0.7 ± 5.1, p = 0.003). Within the scan group, increasing baseline CAC score was associated with a dose–response improvement in systolic and diastolic blood pressure (p < 0.001), total cholesterol (p < 0.001), LDL cholesterol (p < 0.001), triglycerides (p < 0.001), weight (p < 0.001), and FRS (p = 0.003). Downstream medical testing and costs in the scan group were comparable to those of the no-scan group; however, it was dependent on the level of CAC score. Shaw et al. prospectively evaluated the procedural cost and resource consumption patterns in the EISNER study (Early Identification of Subclinical Atherosclerosis by Noninvasive Imaging Research) study after CAC measurement during the 4-year follow-up period using Medicare reimbursement rates. They showed that downstream frequency of medical tests and costs was markedly differential, rising progressively with increasing CAC scores which represented only a small proportion of the study (8.2 % having CAC scores ≥400). The radiation exposure associated with CAC scans has been significantly reduced, now comparable to 1–2 mammograms (~1 mSv) [36, 37] while providing important information about the subject coronary risk and cardiac anatomy.
Predictive Value of Serial CAC Score Progression
Atherosclerosis is a dynamic process which may be affected by traditional risk factors, environmental factors, and therapeutic interventions. Baseline CAC represents as a single point in time on atherosclerosis time curve, whereas progression presents the slope of that curve. CAC progression may provide insight into ongoing atherosclerotic disease activity. Therefore, CAC progression might represent a better marker of disease activity, a better predictor of future adverse cardiovascular events and can be used to evaluate the effect of various therapeutic interventions. However, there are certain inherent limitations to the use of CAC progression before it can be used as a clinical measure for risk assessment and evaluating therapeutic efficacy. This may be due to the method of measurement (Agatston scoring may not represent true change in coronary plaque due to its stepwise nature of the density factor), interscan variability, and the method to determine CAC progression (absolute change, percent change, or mathematical transformation of CAC scores). Measurement of CAC progression highly depends on accurate reproducibility of CAC scores [38, 39]. In general, interscan variability increases as the level of baseline CAC increases and can introduce a bias in the evaluation of CAC progression [40]. Thus, high baseline CAC can introduce overestimation of the actual progression of atherosclerosis measured as a change in absolute CAC compared with those with low baseline CAC. Hokanson et al. [40] suggested that using a progression of square root-transformed calcium volume score ≥2.5 mm [3] (the SQRT method) can show a significant change in CAC progression. The SQRT method corrects for the bias in the progression of CAC introduced by high levels of baseline CAC and provides a stable estimate of interscan variability across the ranges of coronary calcium. Budoff et al. showed that CAC progression was significantly associated with mortality even after adjusting for baseline CAC, age, sex, time between scans, hypertension, hypercholesterolemia, diabetes, family history, and smoking (hazard ratio = 3.32, 95 % CI: 2.62–4.20, p < 0.0001) in 4,609 consecutive asymptomatic individuals undergoing two scans. Adjusted models showed that the presence of CAC at baseline and associated significant progression of CAC was a significant predictor of future mortality (HR: 5.33, 95 % CI: 3.74–7.60, p < 0.0001) [41]. This study also evaluated various methods of CAC progression estimation and their performance in predicting future mortality [absolute score difference, percent annualized difference between baseline and follow-up scans, and the difference between square root of baseline and square root of follow-up CAC score >2.5 (the SQRT method)]. The SQRT method was found superior followed by a percent increase in CAC score (>15 % yearly increase) in predicting mortality. Berry et al. [30] showed that among 2,988 study participants in CARDIA study with low 10-year risk of a cardiac event, those with a high lifetime risk had significantly greater annual CAC progression (22.3 % vs. 15.4 % in men; 8.7 % vs. 5.3 % in women) compared with those with a low lifetime risk. Similar results were observed in the 1,076 MESA participants in this study. Raggi et al. [42] in an observational study related the occurrence of acute myocardial infarction to CAC progression in 817 asymptomatic individuals referred for sequential electron beam tomographic imaging (average interval 2.2 ± 1.3 years). The yearly mean absolute calcium volume score change in 45 patients who had myocardial infarction was 147 compared with 63 patients without events. In another study with 495 asymptomatic subjects undergoing sequential CAC scans and statin therapy after the initial scan, Raggi et al. showed that subjects having myocardial infarction had a greater annual change of CAC scores compared with those who did not have an event (42 ± 23 % vs. 17 ± 25 %, p = 0.0001), respectively [43]. Relative risk of having myocardial infarction in the presence of CAC progression was 17.2-fold (95 % CI: 4.1–71.2) higher than without CAC progression (p < 0.0001). The follow-up CAC score (p = 0.034) as well as a score change >15 % per year (p < 0.001) were shown to be independent predictors of time to MI. The St. Francis Heart Study [3] prospectively evaluated the prognostic accuracy of CAC progression in the prediction of cardiac events in 4,613 adults between 50 and 70 years of age. Follow-up was over 4.3 years, and events occurred in 119 subjects (2.6 %). The median increase in CAC score was significantly higher in those with cardiac events compared with those without events (247 vs. 4) respectively.
CAC progression has been shown to correlate with all the traditional risk factors; however, the association is not consistent across studies. This may be due to the differences in baseline cohort demographics or the measure of CAC progression utilized [44–53]. Kronmal et al. [44] showed that most traditional cardiovascular risk factors were associated with the risk of developing new incident CAC and an increase in existing calcification in a multiethnic population of 5,756 participants in the MESA study. The incidence of newly detectable CAC averaged 6.6 % per year. There were few factors that were associated with only incident CAC risk such as low- and high-density lipoprotein cholesterol and creatinine. Diabetic patients are more likely to develop CHD and tend to have greater plaque burden than patients without diabetes [54, 55]. Diabetes mellitus was found to have the strongest association of CAC progression for blacks and weakest for Hispanic, with intermediate association for whites and Chinese in the MESA study [44].
Role of CAC in Guiding Therapeutic Interventions
CAC represents the culmination of various processes going on in the body that result in the formation of coronary atherosclerotic plaque. Atherosclerosis is a dynamic process undergoing both progression and regression. It is possible to alter the progression of atherosclerosis by modifying risk factors, lifestyle changes, and therapeutic interventions. On the other hand, CAC can also be used to follow the effect of various therapeutic regimens for coronary artery risk reduction. However, the literature evidence is not consistent for the role of CAC as a primary end point for preventive therapeutic regimens. Arad et al. [56] performed a double-blinded, placebo-controlled randomized clinical trial of atorvastatin 20 mg daily, vitamin C 1 g daily, and vitamin E (alpha-tocopherol) 1,000 U daily, vs. matching placebos in 1,005 asymptomatic, apparently healthy men and women age 50–70 years with coronary calcium scores at or above the 80th percentile for age and gender and followed them for 4.3 years for occurrence of cardiac events. Despite the significant reduction in LDL, there was no significant effect on CAC progression and failed to significantly reduce the primary end point, a composite of all atherosclerotic CVD events. However, events rate was reduced in patients with CAC >400 (8.7 % vs. 15.0 %, p = 0.046). Houslay et al. [57] performed a double blind randomized controlled trial with 48 patients receiving atorvastatin and 54 to placebo and a median follow-up of 24 months. Despite reduction in LDL and C-reactive protein concentrations, there was a significant increase in CAC scores in atorvastatin group compared with the placebo group (26 % vs. 18 %) respectively. Similarly, Terry et al. [58] performed a randomized trial with participants receiving simvastatin 80 mg or matching placebo for 12 months. Despite significant reduction in LDL, total CAC volume increased in the treatment group compared with placebo (9 % vs. 5 %), respectively. Schmermund et al. [59] performed a multicenter randomized clinical trial to evaluate the effect of intensive vs. standard lipid-lowering therapy (80 vs. 10 mg atorvastatin) on CAC progression over 12 months period. The mean progression of CAC volume score was nonsignificantly different between two groups [27 % (95 % CI: 20.8–33.1) in 80 mg atorvastatin group vs. 25 % (95 % CI: 19.1–30.8) in 10 mg atorvastatin group, p = 0.65]. Similarly, in another randomized clinical trial involving postmenopausal women randomized to aggressive (atorvastatin 80 mg/day) vs. moderate (pravastatin 40 mg/day) lipid-lowering therapy. After 12 months of treatment, the median percent increase in total CAC volume score change was similar among women receiving atorvastatin and those receiving pravastatin (15.1 % vs. 14.3 %, p = 0.64), respectively [60].
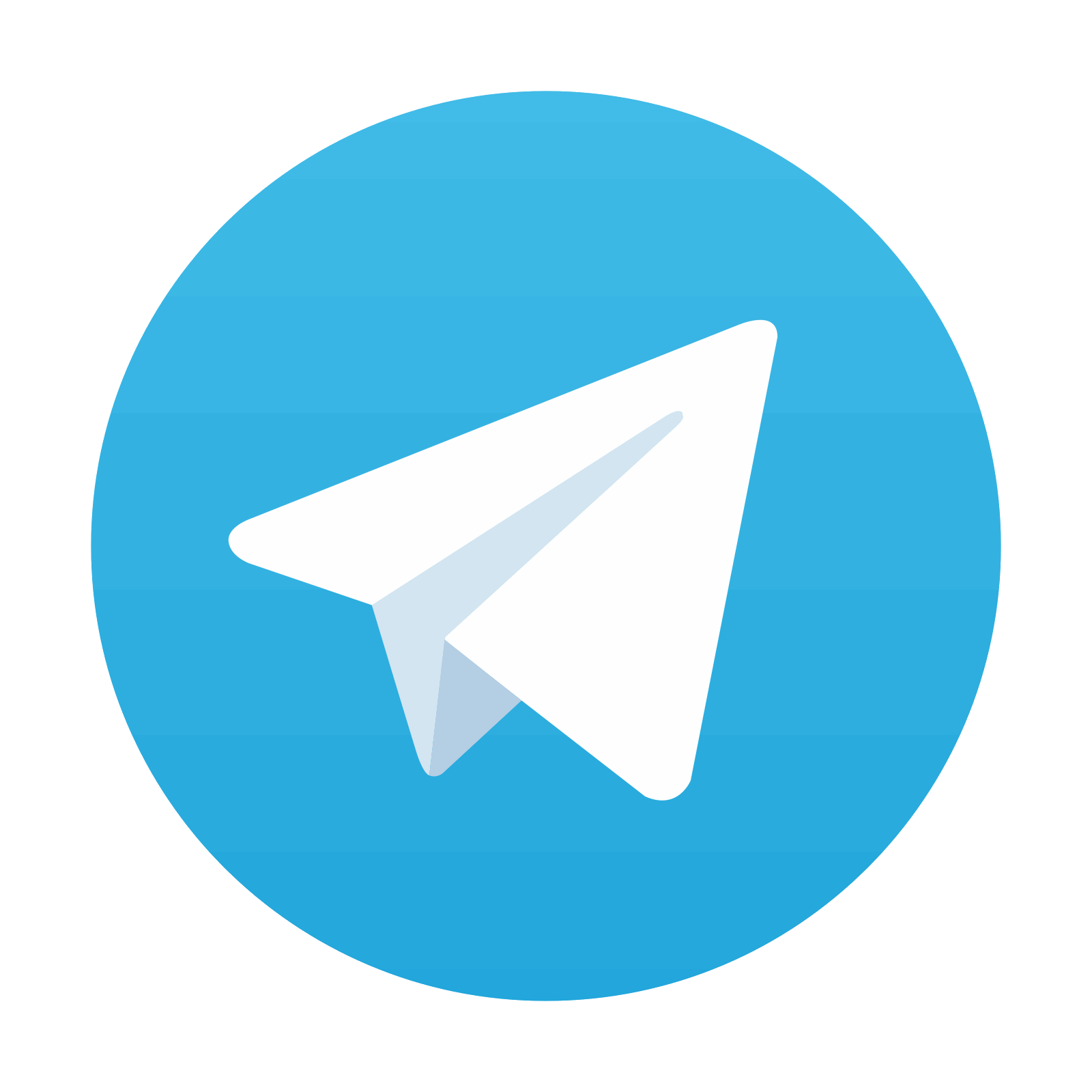
Stay updated, free articles. Join our Telegram channel

Full access? Get Clinical Tree
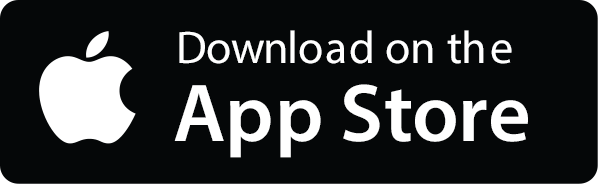
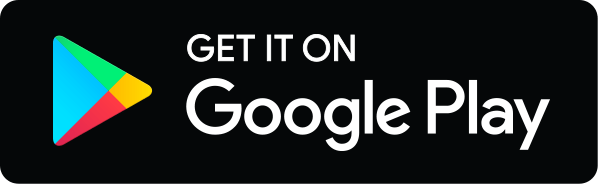