Understanding the complex anatomy and development of the hip is crucial for diagnosing and categorizing femoral neck stress fractures in athletes. These fractures result from repetitive mechanical loading, affecting trabecular structure. Various risk factors include hormonal imbalances and hip geometry. Although radiographs are initial diagnostic tools, MR imaging is the gold standard, distinguishing between stress reactions and fractures. Treatment involves surgical fixation, whereas nonsurgical methods for less severe fractures have good results. Overall, comprehensive knowledge is essential for effective diagnosis and correct early management of femoral neck stress fractures.
Key points
- •
Understanding the detailed anatomy of the hip is crucial for diagnosing and classifying femoral neck stress fractures in athletes.
- •
Femoral neck stress fractures result from repetitive mechanical forces that alter the internal architecture of the proximal femur.
- •
MR imaging has become the gold standard for diagnosing stress fractures due to its high sensitivity and specificity.
- •
Multiple classification systems exist based on biomechanical characteristics, fracture line extension, and presence of joint effusion.
- •
Fractures involving more than 50% of the femoral neck or lateral trabeculae should undergo surgical fixation to prevent serious complications such as fracture progression, fragment displacement, and osteonecrosis.
Introduction
Hip pain in athletes presents a diagnostic challenge, with femoral neck stress fractures (FNSFs) emerging as a significant concern due to their potential for debilitating complications. Understanding the intricate anatomy and embryology of the hip joint is crucial for accurate diagnosis and classification of FNSF. Biomechanical forces within the proximal femur play a pivotal role, with disruptions in tensile trabeculae predisposing individuals to fracture progression. Advanced imaging modalities, particularly MR imaging, offer superior sensitivity in detecting FNSF. Classification systems aid in stratifying fracture severity, guiding treatment decisions, and estimating return-to-sport timelines. Despite diagnostic advancements, differential diagnosis remains challenging, necessitating careful consideration of overlapping conditions. Timely intervention, including surgical fixation for high-risk fractures, is essential to prevent complications and ensure optimal outcomes for athletes. A comprehensive understanding of hip anatomy, pathophysiology, and imaging modalities is essential to ensure effective management of FNSF in athletes.
Anatomy and embryology
The hip is a “ball- and -socket” diarthrodial joint, formed by the spherical head of the proximal femur and the concave articular cavity of the acetabulum, which is in turn formed by the junction of the ilium, ischium, and pubic bones of the pelvis.
Hip embryologic formations begin at 4 weeks of gestational age with the appearance of limb buds, which are protrusions of the ventrolateral wall of the embryo. By 8 weeks, the embryonic differentiation of the hip, knee, ankle, and feet occurs, along with the appearance of the proximal ossification center, the joint capsule, synovial lining, and the basic triangular configuration of the acetabulum. By 11 weeks, the infantile morphology of the hip has been achieved, maintaining a flexed position and beginning the period of growth and maturation, which continues until the 16th week when the hip joint space is completely formed.
In the postnatal period, the acetabular cartilage complex is formed by three main ossification centers that appear between 8 and 9 years of age. Eventually, by the age of 17 to 18 years, these centers will form the anterior acetabular wall from the pubis, the superior acetabulum from the iliac bone, and the posterior acetabulum from the ischium. , It is important to highlight that it is the femoral head that leads the acetabulum to the correct depth and shape, with the labrum forming the outer margin of the acetabulum. The proximal femur has three growth plates, including the longitudinal growth plate, the greater trochanter, and the femoral neck isthmus, supporting the growth in length and width of the proximal femur.
The main components of the hip joint are the femoral head and the acetabulum, but a particular role is played by a third component, the labrum, which is triangular fibrocartilage extending the area of the acetabular surface up to 28%. The labrum also possesses shock-absorbing properties and seals the fluid within the joint. A fourth component is the thick joint capsule, which extends from the acetabulum to the intertrochanteric line, reinforced by the iliofemoral ligament (the strongest), the pubofemoral ligament, and the ischiofemoral ligament ( Fig. 1 ).
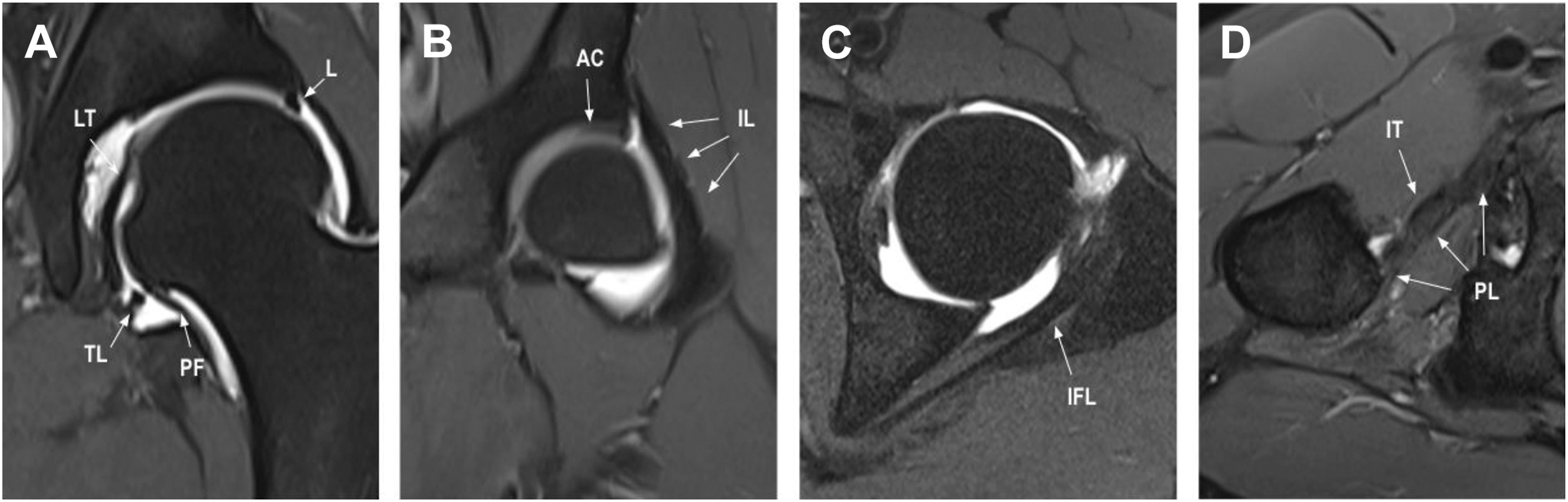
Pathophysiology
The internal architecture of the proximal femur exhibits intricate organization in response to static and dynamic external forces. It displays a distinct and widely recognized trabecular pattern that plays a crucial role in the pathophysiology of stress fractures and their patterns. The internal architecture comprises two primary components: the tensile and compressive trabeculae ( Fig. 2 ).
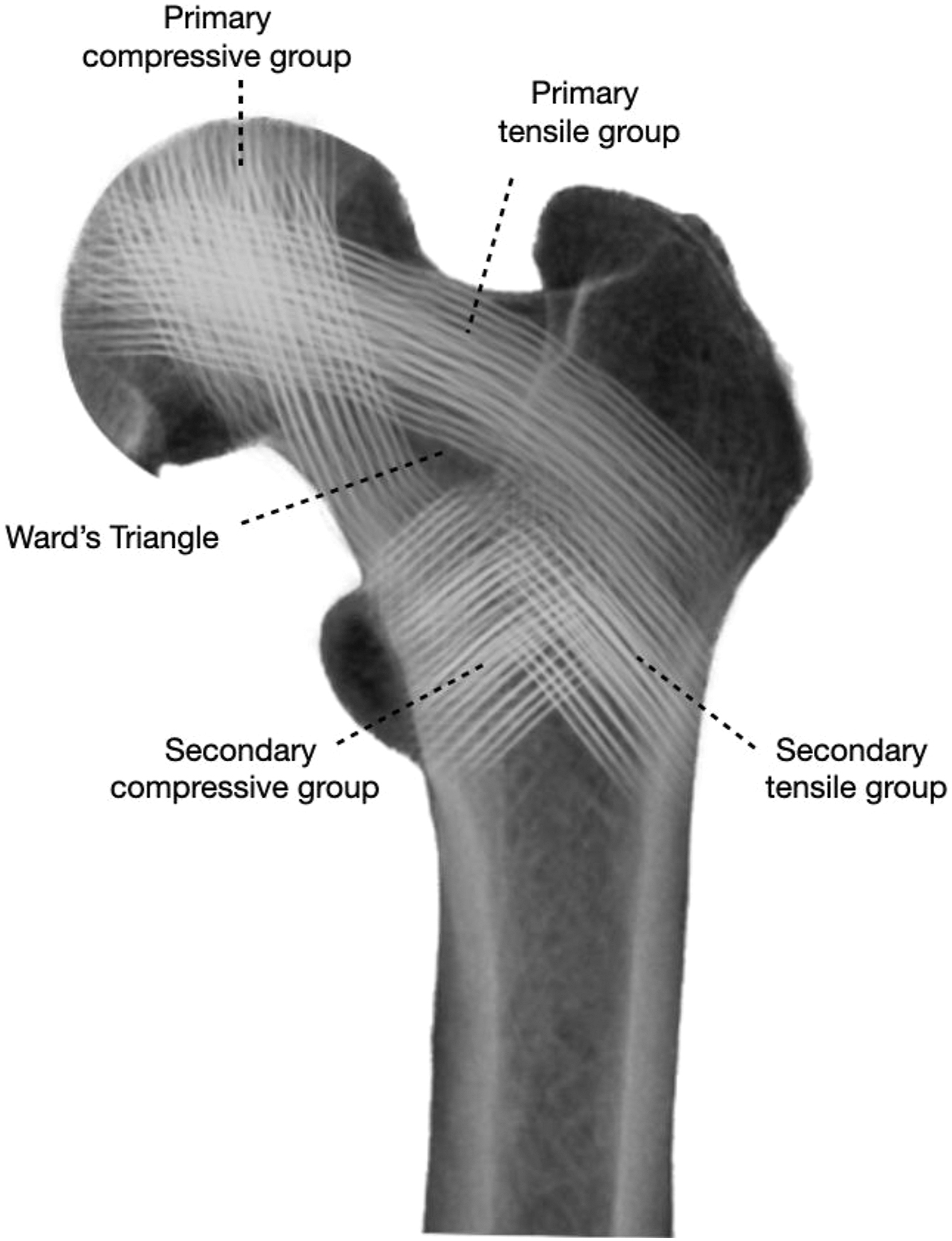
Tensile trabeculae, including principal and secondary trabeculae, mimics the archlike morphology of the femoral neck; disruptions in this mechanism can lead to tension fractures. The medial and lateral compressive trabeculae are oriented more vertically, forming a “V.” Disruptions in this component lead to compression fractures within the medial aspect of the femoral neck. Changes in the applied forces to the femur can induce alterations in trabecular distribution, ultimately resulting in the weakening of specific regions over time.
A stress fracture is defined as a fracture of the bone caused by repeated, rather than sudden, mechanical loading. Before cortical break, there is focal microtrabecular disruption and weakening known as stress injury. Loading forces activate osteoclastic activity, and rest leads to osteoblastic activity, maintaining the homeostasis of bone resorption and formation. When repetitive overloading exceeds rest periods, an imbalance of osteolytic activity appears, leading to bone weakening and microtrabecular disruption that eventually ends in cortical break. Stress fractures of the femur present differently based on age, bone density, and muscle health. In elderly patients with low bone density, these fractures received the name of insufficiency fractures , which usually occur with nonrepetitive loading. Disrupted trabeculae are typically on the tensile groups, carrying a greater risk of fracture progression.
In young individuals subject to repetitive loading, the compressive trabeculae are typically affected, causing disruption of the medial aspect of the femoral neck, potentially progressing at a 45° angle with an oblique pattern. Although repetitive loading forces, particularly in activities such as running, jumping, and ballet dancing, , are the main etiologic factors, other conditions have shown to increase the risk of stress fractures. These conditions include any condition affecting normal trabecular distribution, such as morphologic variations (coxa vara and femoroacetabular impingement) and hormonal abnormalities, the latter typically observed in military and athlete women with decreased estrogen levels or those taking gonadotropin-releasing hormone analogues or daily oral contraceptive pills. Additional risk factors include lower body weight, poor caloric intake, and tobacco use. Recently discovered predisposing factors include calcitonin receptor rs1801197 and LRP5 rs2277268 polymorphisms. Besides physiologic factors, it has been proved that alterations in hip geometry, such as elevated hip axis length (distance from the pelvic rim to the outer margin of the greater trochanter along the neck axis) and elevated femoral neck shaft angle and femoral neck width, could be an independent risk factor to develop femoral neck fractures.
Imaging
Stress fractures constitute up for 20% of all injuries observed in sports medicine clinics, with hip stress fractures accounting for 3% of all sport-related stress fractures. Radiographs remain the imaging modality of choice for the initial diagnosis of hip stress fractures even though they seem normal in 90% of the initial radiographs and in 50% of repeated radiographs after 4 to 6 weeks. More recent studies using MR imaging demonstrate high specificity (97%) and sensitivity (99%) in diagnosing stress fractures.
Radiographs including an anteroposterior (AP) view of the pelvis and AP and frog-leg lateral views of the affected hip are a widely accessible modality that can reveal other differential diagnoses and provide diagnostic clues that may indicate FNSF. Although they have low sensitivity (15%–35%), subtle periosteal or endosteal cortical reactions, sclerotic lines traversing the compressive trabeculae, radiolucent fracture lines, and poor definition of the affected cortex, known as the gray cortex sign ( Fig. 3 ), aid radiologists in diagnosing femoral neck stress fractures. Computed tomography (CT) has also proved to have less sensitivity and specificity than MR imaging for detection of occult fractures of the femoral neck.
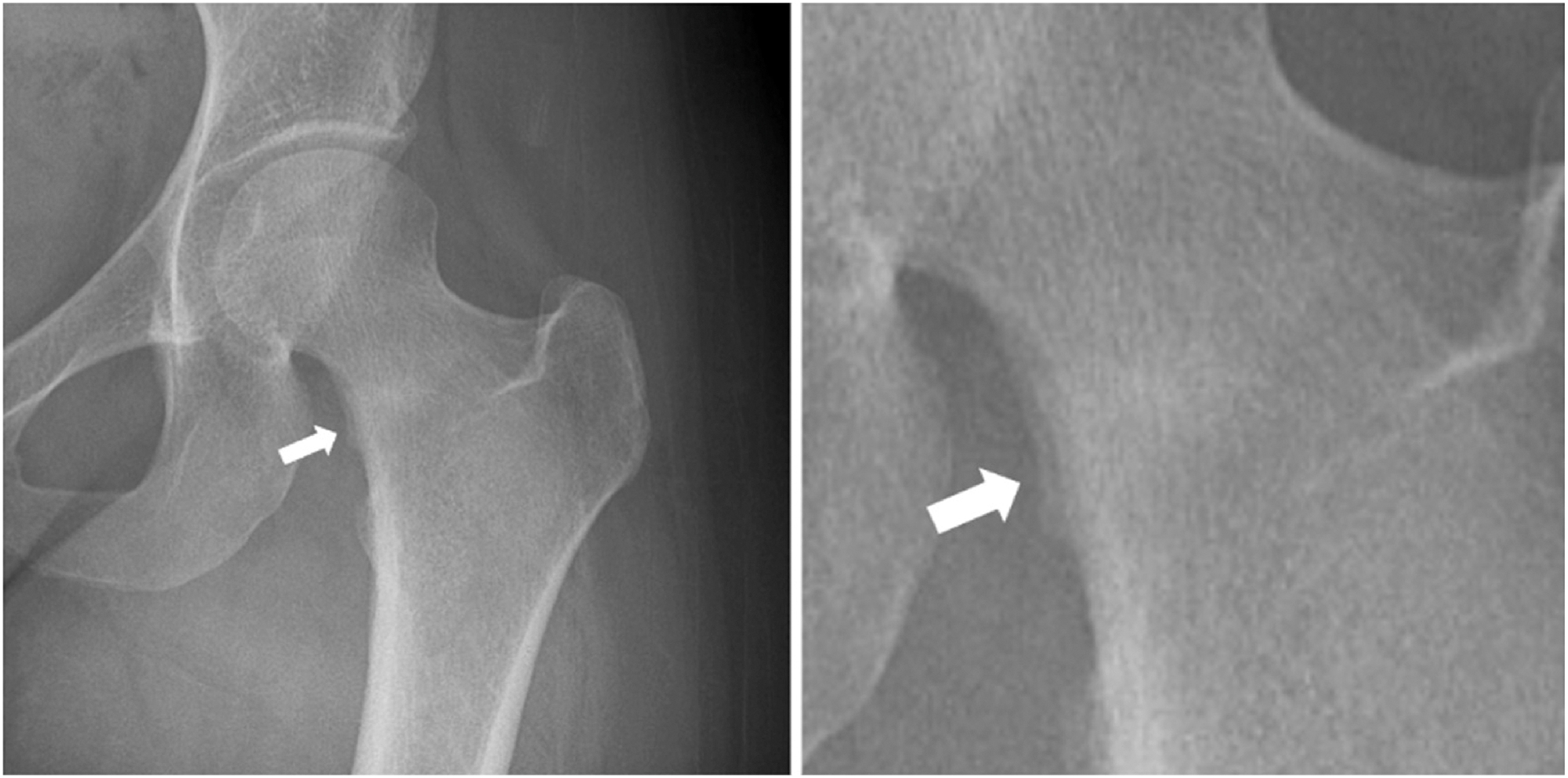
Tc-99m bone scintigraphy was considered the gold standard for identifying FNSF for many years due to a sensitivity of 97.3% and a negative predictive value of 95.6% for the detection and exclusion of both stress reactions and stress fractures. Nevertheless, even when bone scintigraphy became positive 2 to 3 weeks before radiographic changes, it was still a late diagnosis compared with magnetic resonance. Bone scintigraphy also has a relatively high false-positive rate that goes up to 32% and cannot differentiate stress reaction from stress fractures due to the lack of anatomic detail.
Because of the outstanding resolution of the bone marrow in MR imaging, this imaging modality has become the gold standard. Fluid-sensitive sequences are the preferred initial sequences to evaluate bone marrow edema, whereas T1-weighted images can demonstrate the fracture line, preserving the distinction between stress reaction and stress fracture.
Classification
There are multiple classification systems for femoral neck stress fractures, the first one described in 1965 by Devas, using biomechanics to classify FNSFs into (1) compression fractures localized in radiographs and affecting the compression trabecular lines but without clear cortical disruption and (2) transverse fractures described as a fracture line that goes transversely across the femoral neck independently if the affected trabeculae is tensile or compressive.
Later Blickenstaff and Morris described three types of FNSFs, the ones with callus but without a fracture line (type 1), FNSFs that have a fracture line in the calcar across the neck without displacement (type 2), and fractures completely displaced (type 3).
One of the more commonly used grading systems is based on fracture line extension and the presence of joint effusion. The lower grade is when the fracture line extension is less than 50% of the femoral neck without effusion; the next grade is when the fracture line compromise more than 50% of the neck, without joint effusion; and finally the more severe case before a complete fracture is when the fracture line extends more than 50% of the neck and is associated with joint effusion ( Fig. 4 ). It is important to note that this grading system excludes bone edema without a visible fracture line, as such manifestations typically indicate stress reactions as demonstrated in MR imaging.
