Fig. 19.1
TBI in a 57-year-old man, injured in a car accident. GCS on admission was 11/15 Noncontrast CT of the brain upon admission (a) and MRI of the brain on day 7 after the injury, including fat-saturated turbo FLAIR (b) and susceptibility weighted imaging (SWI) (c) were performed. The coup side is indicated by a left parieto-occipital subgaleal hematoma and infiltration of the subcutaneous fat (arrow). On the contre-coup side, there are hemorrhagic contusions in the right frontal and temporal lobes. In addition, there is a small right parietal subdural hematoma, but the midline structures are not displaced. The MRI scan on day 7 reveals hemorrhagic contusions in the right temporal and right frontal lobes, surrounded by edema. On the SWI scan, the hemorrhagic nature of these contre-coup contusions is clearly seen, and there is evidence of multiple other hemorrhagic foci in the brain parenchyma, as well as small amounts of intraventricular blood in the trigone and occipital horns of the lateral ventricles
Specific MRI sequences, i.e., susceptibility weighted imaging (SWI) and diffusion weighted imaging (DWI), are more sensitive for detecting structural changes in the brain, particularly smaller lesions, such as microhemorrhages, diffuse axonal injury (DAI), and traumatic axonal injury (TAI) (Fig. 19.2) [7]. Diffusion tensor imaging (DTI) provides valuable additional information about white matter lesions and structural damage, and is therefore particularly suited to characterize the presence and degree of injury and understand pathophysiological mechanisms in neurotrauma.
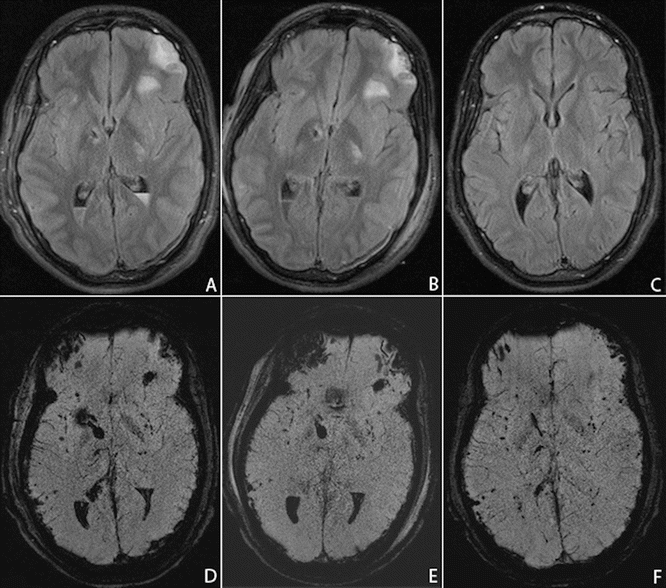
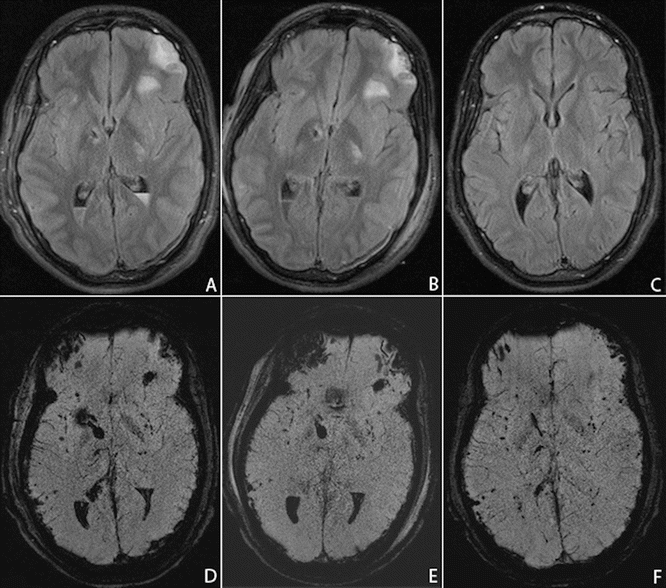
Fig. 19.2
TBI in a 19-year-old man, injured in a motor vehicle accident. The patient was admitted to the emergency department with a GCS of 9/15. MR imaging was performed on day 7 (a, d), day 12 (b, e), and after 6 months (c, f), using fat-saturated turbo FLAIR (a, b, c) and susceptibility weighted imaging (SWI) (d, e, f). The MRI scan on day 7 (a, d) reveals left frontal and bilateral capsulo-lenticular contusions, as well as intraventricular hemorrhage and multiple scattered hemorrhagic foci. A prominent susceptibility artifact in the right frontal region is caused by the ventricular shunt. Five days later, on day 12, the parenchymal lesions are unchanged, but there is a slight decrease in the amount of intraventricular hemorrhage. After 6 months (c, f) the intracerebral lesions have regressed on the FLAIR image, but there remain multifocal, punctate microhemorrhagic foci on the SWI sequence. In addition, a mild cortical atrophy has occurred
MR imaging can visualize abnormalities, consistent with axonal injury in up to 30–50 % of patients, in whom CT showed no apparent structural damage (Fig. 19.3) [8–10]. It is important to detect such lesions since TAI is a major cause of cognitive impairment and disability after TBI and thus a determining factor for outcome [11–18]. For example, in mild TBI patients with impaired executive function, reduced FA values were seen in the dorsolateral prefrontal cortex (DLPFC ) [19]. A study by the NICER consortium demonstrated that microstructural changes were observed up to 5 years after severe TBI [20].
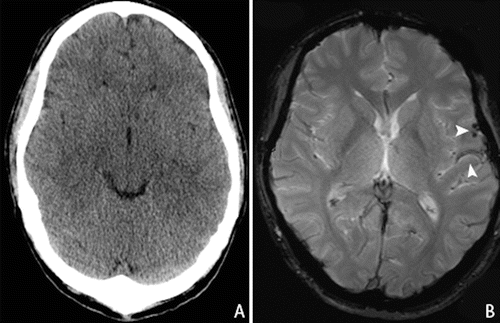
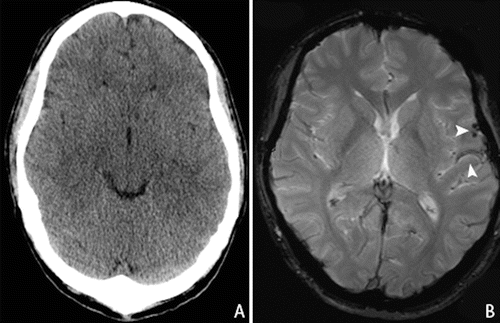
Fig. 19.3
Mild TBI in a 35-years-old patient who fell from the stairs, with GCS of 14/15 (E4M6V4) on admission. CT scan (a) upon admission showed no intracranial abnormalities. MR imaging—gradient echo T2 sequence—(b) 6 weeks after initial injury shows remnants of subarachnoid hemorrhage in the left parieto-temporal lobe (arrow)
This chapter will elaborate on the role of diffusion tensor imaging in traumatic brain injury for research and clinical practice.
Role of DTI in TBI
Pathophysiology
The pathophysiology of TBI is highly complex and involves multiple pathophysiologic processes. Importantly, TBI should not be seen as an event, but as a progressive disease, in which further damage may occur over hours, days, weeks, months, or even years. Secondary damage is preventable and potentially treatable. Detection, quantification, and tracking of such secondary damage is thus of paramount importance. Secondary damage may include brain swelling due to vascular engorgement or brain edema, which may be intracellular (cytotoxic) or vasogenic (extracellular) . Cerebral ischemia, considered one of the most common problems after TBI, can occur locally (in the penumbra of a contusion) or more generalized and may be exacerbated by systemic insults, such as low blood pressure or inadequate blood oxygenation. Traumatic axonal injury , formerly considered a mechanical disruption of axons, has now been shown to result from metabolic failure of axonal transport mechanisms. Both hypo- and hypermetabolism may occur at different stages after injury. Disturbances of the mitochondrial transition pore are thought to be a main cause of mitochondrial failure after TBI. Inflammatory cascades are activated, some of which may be protective, others—when in excess—detrimental. Much insight into these mechanisms can be gained from MR Imaging. DWI and apparent diffusion coefficient (ADC) mapping can provide information about ischemia and edema, whilst MR spectroscopy can provide insight into metabolic derangements.
DTI in Diagnosis, Characterization , and Classification of TBI
DTI can provide important indirect information about neuronal integrity, continuity, and connectivity of neural pathways , even when traditional MRI sequences appear normal. The diffusion tensor characterizes the magnitude of water diffusion (ADC and mean diffusivity, MD), its directional nonuniformity (fractional anisotropy, radial and axial diffusivity, FA, RD, AD), and its orientation (the tensor eigenvectors). The exact mechanisms of the nature of water diffusion in both grey and white matter are not completely understood. However the organization of tissue structure including the presence of myelin, microtubules, and organelles, as well as the contribution of intra- and extracellular water (edema formation) play a significant role. This is illustrated in a study by Newcombe et al. [21] in subjects with moderate to severe head injury where MRI was performed at a median of 32 h after injury. Results showed a decreased FA in the white matter, which was attributed predominantly to increased radial diffusivity, consistent with edema.
In 2012, Shenton et al. published a review of MRI and DTI focusing on mild traumatic brain injury. This article gives a good overview of studies performed on mild TBI, with all analyzed data showing some degree of subtle brain damage in mild TBI. The authors noted a wide range of variability in DTI studies and difficulties in data interpretation, due to the use of different scanning protocols and timings at DTI.
A meta-analysis of 28 studies by Aoki et al. [22] in mild TBI showed that the posterior part of the corpus callosum is more vulnerable to damage compared to the anterior part. Compared to healthy volunteers, there was a significant decrease in FA and increase in mean diffusivity in the corpus callosum in TBI patients.
In a study by Rutgers et al. [23] on 21 patients with mild TBI, in whom MRI was performed at an average of 5.5 months after injury, the cerebral lobar white matter showed regions with reduced FA values in almost 62 % of the study population. Further decrease of FA was observed in 23.6 % of the patients in the cingulum or the corpus callosum. Since abnormalities in the internal capsule, fornix, brain stem, and cerebellum were not frequently seen in their case study of mild TBI, the authors suggest these lesions may be more associated with severe TBI.
Kraus et al. [24] performed a DTI study in chronic traumatic brain injury patients with mild, moderate, and severe injury. The study included a total of 20 patients with mild and 17 patients with moderate/severe TBI as well as 18 healthy controls. DTI was performed at least 6 months after injury (average of 107 months after injury), using both whole brain analysis and region of interest analysis. Results showed an increased radial and axial diffusivity in patients with moderate and severe TBI in both whole brain and ROIs, indicative of potential myelin and axonal damage .
Notably, differences in the effect of TBI severity on AD and RD may reflect different underlying pathophysiological mechanisms related to the degree of tissue injury.
Recently, Van der Eerden and the Neuroimaging for Coma Emergence and Recovery (NICER) consortium [25] published the results of a multicenter study investigating DTI changes in cardiac arrest and TBI patients, using 19 predetermined ROIs. They found a decrease in axial diffusivity in cardiac arrest patients and increase in radial diffusivity in severe TBI patients. In the cardiac arrest patients, abnormalities are mostly seen in the cerebral hemispheres. For the TBI patients the abnormalities were found in both the central brain structures and cerebral hemispheres. The changes in axial diffusivity could be related to primary axonal damage due to energy depletion caused by ischemia. It was hypothesized that the increase in radial diffusivity in TBI patients was related to myelin damage and edema. The moderate decrease in axial diffusivity suggests axonal damage due to direct impact at trauma or ischemic changes due to secondary mechanism caused by intracranial lesions . However, relating changes in the axial and radial diffusivities to specific microstructural features is fraught with challenges, particularly in the context of tissue injury. As a consequence, these hypotheses for the observed DTI changes remain tentative.
Kasahara et al. [26] performed DTI in healthy controls and patients with mild TBI and DAI. Decreased axonal and radial diffusivity was found in the DAI patients. In mild TBI patients, normal radial diffusivity and increased axial diffusivity values were observed, indicating possible axonal abnormality.
DTI and Prognosis in TBI
DTI may also be used as a prognostic predictor in traumatic brain injury.
A DTI voxel-based analysis by Perlbarg et al. [27] investigating the prediction of 1-year outcome in severe TBI showed no significant ADC differences between the favorable and unfavorable outcome groups. However, significant changes were observed in the FA values between these two groups, with a decrease in the FA value in the unfavorable outcome group.
Tollard and colleagues [28] performed a study with DTI and magnetic resonance spectroscopy (MRS) for outcome prediction in severe traumatic brain injury. Forty-three patients with severe TBI and 15 control subjects underwent MRI with DTI and MRS sequences. The imaging was performed in the subacute phase after trauma (24 ± 11 days after injury). DTI was performed using symmetrical regions of interest (ROI) analysis in the left and right mesencephalon, temporal and occipital white matter, anterior and posterior centrum semiovale, and anterior and posterior part of the pons.
These regions were predetermined regardless of the presence of morphologic lesions.
The results revealed significantly lower FA values in patients with unfavorable outcome (i.e., Glasgow Outcome Scale, GOS 1–3). Lower FA values were also seen in the temporal white matter and the centrum semiovale for patients with favorable outcome (i.e., GOS 4–5) compared to the control group. The authors suggest this may be because these lesions have no effect on the recovery of consciousness.
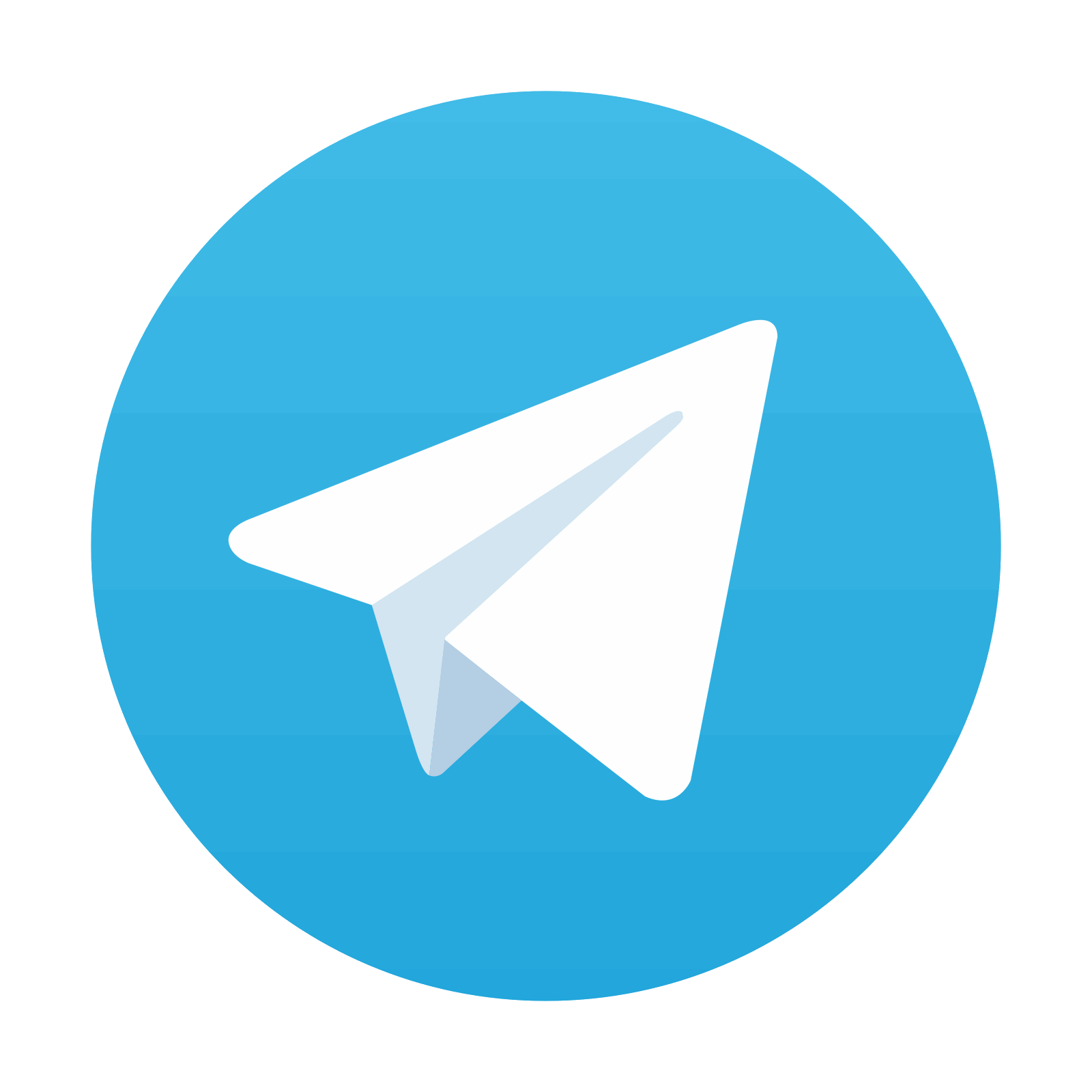
Stay updated, free articles. Join our Telegram channel

Full access? Get Clinical Tree
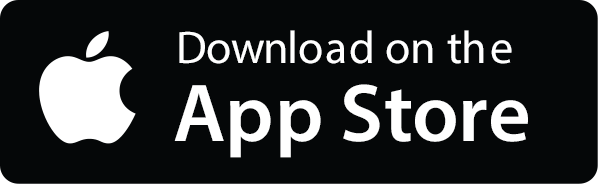
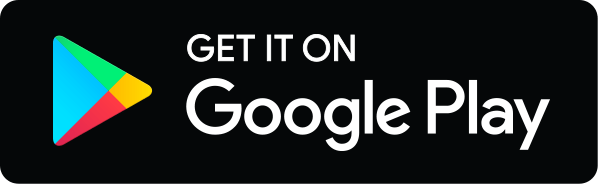