Introduction
In this chapter, we review the concepts and principles necessary for understanding and interpreting color Doppler images and pulsed Doppler waveforms and discuss the key elements needed for the interpretation of Doppler spectral waveforms. This includes a study of normal waveform patterns and characteristic changes that occur with pathologic states. Important tips are given for recognizing Doppler spectral features that are essential for the diagnosis of arterial stenosis and occlusion. We discuss the principles of color and power Doppler, including the advantages and limitations of these imaging modes. Finally, we conclude with a brief overview of three-dimensional (3D) and B-mode imaging.
Blood Flow Patterns
In general, blood vessels change size and direction as they course through the body. Blood flow velocities also change depending on the size of the vessel lumen and the change in direction of flow. In addition, arterial blood flow is pulsatile and faster in the center of the vessel and slower at the periphery of the vessel lumen. Moreover, the vessel lumen may be distorted by atherosclerosis and other pathology. For these reasons, during Doppler evaluation, blood flow produces a mixture of Doppler frequency shifts that changes from moment to moment and from place to place within the vessel lumen. Spectrum analysis is needed to sort out the jumble of Doppler frequencies generated by blood flow and to provide quantitative information that is critical for diagnosis of vascular pathology.
The Doppler spectrum
The word spectrum , derived from Latin, means image . You may think of the Doppler spectrum as an image of the Doppler frequencies generated by moving blood. In fact, this image is a graph showing the mixture of Doppler frequencies present in a specified sample of a vessel over a short period of time. The key elements of the Doppler spectrum are time , frequency , velocity , and Doppler signal power; these elements appear on the images presented in Fig. 3.1 . Please refer to the images while reading about these important components of the color and spectral displays. We begin with Fig. 3.1A :
Color flow image: The vessel, the sample volume, and the Doppler angle are shown in the color flow image at the top half of the display screen.
Color flow information: The color bar to the left of the image shows the relationship between the direction of blood flow and the colors in the flow image. By convention, the upper half of the bar shows flow toward the transducer. This is logical because this part of the bar is nearest to the transducer in the image. The lower half represents flow away from the transducer. In this case, red/yellow colors correspond to flow toward the transducers, and blue/light blue colors indicate flow in the opposite direction. A shift in color from red to yellow or from blue to light blue represents increasing flow velocity.
Doppler angle: The Doppler angle for the spectral Doppler appears to the right of the color flow image, in this case 60 degrees (AC 60 = angle correction 60 degrees).
Time: The time is represented on the horizontal ( x ) axis of the Doppler spectrum at the base of the display. The lines represent divisions of a second, but a scale is not always provided.
Velocity: Blood flow velocity (cm/s) is shown on the vertical ( y ) axis of the spectrum. In this case, velocity is shown on the vertical axis as cm/s (centimeters/second).
The distribution of velocities within the sample volume is illustrated by the brightness of the spectral display (the z -axis). To better understand the z -axis concept, examine the magnified spectrum shown in (B) and imagine that the spectral display is made of tiny squares called pixels (picture elements). You cannot see the pixels in this image because they are purposely blurred to smooth the picture. The pixels are there, however, and each corresponds to a specific moment in time and a specific frequency shift or velocity. The brightness of a pixel (its z-axis) is proportionate to the number of blood cells causing that frequency shift at that specific point in time . In this example, the pixels at asterisk 1 are bright white, meaning that a large number of blood cells have the corresponding velocity (about 90 cm/s) at that moment in time. The pixels just below asterisk 2 are slightly darker, meaning that fewer blood cells have the corresponding velocity (about 20 cm/s) at that moment. The pixels below asterisk 3 are black, meaning that no (or very few) blood cells have the corresponding velocity at that moment. If this is not clear, read this again and remember that the brightness of each pixel is proportionate to the relative number of blood cells with a specific velocity at a specific moment in time. Because the brightness of the pixels also shows the distribution of flow energy, or power, at each moment in time, the spectrum display is also called a power spectrum.
Flow direction : The direction of flow is shown in relation to the spectrum baseline. In this case, flow toward the transducer is shown above the baseline, and flow away from the transducer is shown below the baseline. Note that the number 50 in the lower right corner is preceded by a minus sign. Because the area below the baseline corresponds to flow away from the transducer, a negative Doppler shift is generated. The relationship between the flow direction and the Doppler baseline may be reversed by the operator, but flow toward the transducer will always be represented by positive velocity or frequency values.
Pulse repetition frequency : Please note the pulse repetition frequency (PRF) on the display on Fig. 3.1A . The PRF for the color flow image is shown on the right of the image under CF (color flow) and is 3.9 kHz. The PRF for the spectral Doppler is located underneath, under PW (pulsed wave) and is also 3.9 kHz. A higher PRF may be needed for the spectral Doppler to ensure that systolic velocities are shown accurately, without aliasing.
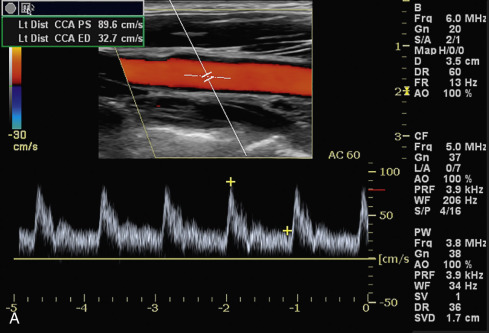
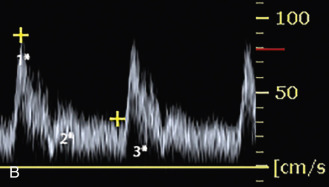
What is a power spectrum?
The Doppler frequency spectrum reviewed in Fig. 3.1 is sometimes called a power spectrum because the power, or strength, of each frequency is shown by the brightness of the pixels. The power of a given frequency shift, in turn, is proportionate to the number of red blood cells producing that frequency shift. If a large number of blood cells are moving at the same velocity, the corresponding Doppler frequency shift is powerful, and the pixels assigned to that frequency shift (velocity) are bright. Conversely, if the same number of red blood cells are moving at slightly different velocities then the pixels assigned to the corresponding frequency shifts are dimmer. The power spectrum concept is important for understanding power Doppler flow imaging, which is discussed later in this chapter. The concept of the power Doppler spectrum is nicely illustrated in Fig. 2.35 .
Frequency shift conversion to velocity information
The echoes that are reflected back to the transducer from moving cells in a sampled blood vessel contain only Doppler frequency shift information; however, the Doppler spectrum usually displays velocity (cm/s or m/s) information. How does the instrument convert the Doppler frequency shift to velocity? This conversion occurs when the sonographer “informs” the duplex instrument of the Doppler angle, which is shown in Fig. 3.2 . If the instrument “knows” the Doppler angle, it can then compute the blood flow velocity via the Doppler formula (see Chapter 2 ).
fD=fR−fo=2foVcosθc
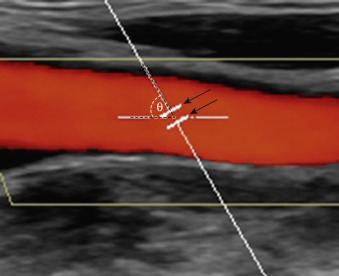
It is desirable to operate the duplex instrument in the velocity mode rather than the frequency mode for several reasons. First, velocity measurements compensate for variations in vessel alignment relative to the skin surface. For instance, the Doppler frequency shift observed in a tortuous internal carotid artery might be radically different from one point to another, but angle-corrected velocity measurements will be similar throughout the vessel, in spite of dramatic changes in vessel orientation relative to the skin. Second, the Doppler frequency shift is inherently linked to the output frequency of the transducer, but velocity measurement is independent of the transducer frequency. For instance, if the output frequency goes from 5 to 10 MHz, the frequency shift is doubled. This could lead to misinterpretation of the findings. If transducers with different frequencies were used to determine stenosis severity, different diagnostic parameters would be needed for each ultrasound transducer (e.g., 3.5, 5, 7.5 MHz). This problem is eliminated when the instrument converts the raw frequency information to velocity data. Third, current diagnostic criteria are presented as velocity parameters. Frequency information is no longer used to determine severity of disease.
- •
The key elements of the Doppler spectrum are time , frequency , velocity , and Doppler signal power .
- •
By convention, the upper half of the color bar and the upper part of the Doppler spectral display show blood flow directed toward the transducer.
- •
A Doppler angle of 60 degrees or less is required to derive accurate frequency and velocity measurements. The Doppler angle should always be checked when using velocity information.
Listening to the Auditory Frequency Spectrum
The human ear was the instrument used initially for Doppler blood flow studies. The ear is a highly capable spectrum analysis instrument, which is evident in its ability to distinguish one person’s voice from another. Even though duplex ultrasound instruments are equipped with electronic spectrum analysis devices, an audible Doppler output is provided as well, to take advantage of the human ear’s capabilities. Certain features of the Doppler flow signal can be appreciated aurally that are difficult or impossible to display electronically, and as a result, the audible Doppler signal remains important in ultrasound vascular diagnosis. For instance, in very high-grade carotid stenoses, a distinctive high-pitched whining or whistling sound is heard. In spite of its abilities, however, the human ear has three major drawbacks. First, the ear is a purely qualitative device; second, it is not equipped with a hard copy output for permanent storage; and third, some ears work better than others—some cannot hear very high frequencies. Electronic spectrum analysis overcomes these obstacles.
The sample volume
The frequency spectrum displays blood flow information from a specific location called the Doppler sample volume , illustrated in Fig. 3.2 . You should be familiar with the following three characteristics of the Doppler sample volume: First, it is, in fact, a volume (three dimensions), even though only two of its dimensions are shown on the duplex image. The “thickness” of the sample volume cannot be shown on the two-dimensional spectrum display, and this can sometimes lead to errors of localization. Doppler signals may be obtained from vessels located near the area of interest and marginally within the sample volume but are not shown on the two-dimensional display. For example, the ultrasound image may show the internal carotid artery, but you may actually be receiving flow signals from an adjacent external carotid branch. Second, the actual shape and size of the sample volume may be somewhat different from the linear representation shown on the duplex image. Third, and most important, the Doppler spectrum displays blood flow information obtained within the sample volume and does not provide information about blood flow in other portions of the vessel visible on the ultrasound image. Therefore if the sample volume is positioned incorrectly, key diagnostic information may be overlooked.
How to determine the direction of flow
The frequency spectrum shows blood flow relative to the transducer . Flow in one direction, toward the transducer, is displayed above the spectrum baseline, and flow in the opposite direction is shown below the baseline. One must always remember that the flow direction is relative to the transducer and is not absolute. The apparent direction of blood flow can be reversed by turning the transducer around or by pressing a button on the instrument that inverts the spectrum! The arbitrary nature of this arrangement can lead to significant diagnostic error. How often is reversal of flow in the vertebral artery missed? Clues to the correct direction of flow can be found by comparing the color (e.g., red or blue) in the vessel with the color bar or color velocity scale and by checking whether the velocity information on the spectrum is positive (toward the transducer) or negative (away from the transducer). Another method to check direction of flow is comparison with a reference vessel in which the flow direction is known (e.g., when working in the abdomen, the aorta is a handy reference vessel).
What You Need to Know About Waveform Analysis
Pulsatility
In arteries, each cycle of cardiac activity produces a distinct wave on the Doppler frequency spectrum that begins with systole and terminates at the end of diastole. The term waveform refers to the shape of each of these waves, and this shape, in turn, defines a very important flow property called pulsatility . In general terms, Doppler waveforms have low, moderate, or high pulsatility features, as illustrated in Fig. 3.3 . Please review the three types of pulsatility on this figure before proceeding to the following discussion.
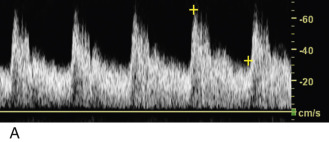
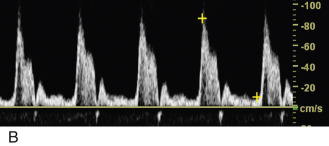
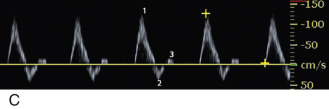
Low-pulsatility Doppler waveforms have broad systolic peaks and forward flow throughout diastole (see Fig. 3.3A ). The carotid, vertebral, renal, and celiac arteries all have low-pulsatility waveforms in normal individuals because these vessels feed circulatory systems with low resistance to flow (low peripheral resistance). Low-pulsatility waveforms are also monophasic , meaning that flow is always forward, and the entire waveform is either above or below the Doppler spectrum baseline (depending on the orientation of the ultrasound transducer). Low-pulsatility waveforms do not demonstrate reversal of flow.
Moderate-pulsatility Doppler waveforms have an appearance somewhere between the low- and high-resistance patterns (see Fig. 3.3B ). With moderate flow resistance, the systolic peak is tall and sharp, but forward flow is present throughout diastole (sometimes interrupted by early-diastolic flow reversal). Examples of moderate pulsatility are found in the waveforms obtained from the external carotid artery and the superior mesenteric artery (during fasting).
High-pulsatility Doppler waveforms have tall, narrow, sharp systolic peaks and reversed or absent diastolic flow. The classic example of high pulsatility is the triphasic flow pattern seen in an extremity artery of a resting individual (see Fig. 3.3C ). A sharp systolic peak (first phase) is followed by brief flow reversal (second phase) and then by brief forward flow (third phase). High-pulsatility waveforms are a feature of circulatory systems with high resistance to blood flow (high peripheral resistance).
Pulsatility and flow resistance may be gauged qualitatively, either by visual inspection of the Doppler spectrum waveforms or by listening to the auditory output of a Doppler instrument. Qualitative assessment of pulsatility is often sufficient for clinical vascular diagnosis, but in some situations (e.g., assessment of renal transplant dysfunction), quantitative assessment is desirable. A variety of measurements have been developed for this purpose, but the most popular are the pulsatility index (of Gosling), the resistivity index (of Pourcelot), and the systolic/diastolic ratio, all of which are illustrated in Fig. 3.4 .
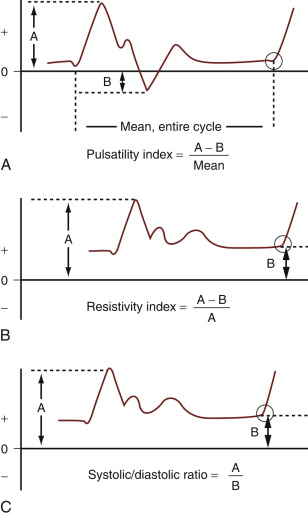
Normal values for pulsatility measurements vary from one location in the body to another. Furthermore, both physiology and pathology may alter arterial pulsatility. For example, the normal high-pulsatility pattern seen in extremity arteries during rest converts to a low-resistance, monophasic pattern after vigorous exercise (because the capillary beds open and flow resistance decreases). Although this monophasic pattern is normal after exercise, it is distinctly abnormal in a resting patient and, in that circumstance, indicates arterial insufficiency resulting from obstruction of more proximal arteries. The point to be made here is that proper interpretation of pulsatility requires knowledge of the normal waveform characteristics of a given vessel and the physiologic status of the circulation at the time of examination. The status of cardiac function is also important; slowed ventricular emptying, valvular reflux, valvular stenosis, and other factors may significantly affect arterial pulsatility and waveform shape.
Acceleration
Acceleration is another important flow feature evident in Doppler spectral waveforms. In most normal situations, red cells in an artery accelerate very rapidly during systole, and the peak velocity is reached within a few hundredths of a second after ventricular contraction begins. Rapid acceleration produces an almost vertical deflection of the Doppler waveform at the start of systole ( Fig. 3.5A ). The systolic upstroke is nearly at a 90-degree angle to the baseline. This is described as a rapid systolic upstroke. If, however, severe arterial obstruction is present proximal (upstream) to the point of Doppler examination, systolic flow acceleration may be slowed substantially, as shown in Fig. 3.5B and C . There is a significant delay to peak systole compared with the normal waveform. Quantitative measurement of acceleration is achieved by measuring the acceleration time and the acceleration rate (index), as illustrated in Fig. 3.6 . These measurements are used, for example, in evaluating renal artery stenosis.
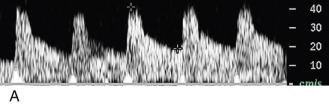
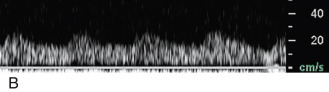
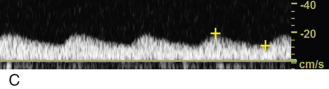
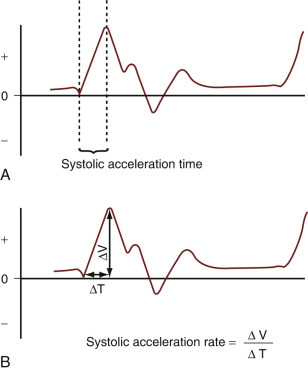
Vessel identity
As you may have already surmised, vessels can be characterized by their waveform pulsatility features. For example, Doppler waveforms readily differentiate between lower extremity arteries, which are distinctly pulsatile, and lower extremity veins, which have gently undulant flow features. In addition, each vascular system has a characteristic Doppler signature. Doppler waveforms are particularly helpful in distinguishing the internal and external carotid arteries, which have low and moderate pulsatility, respectively. Pulsatility is also of value within the liver for differentiating among the portal veins, hepatic veins, and hepatic arteries, as discussed in Chapter 27 .
Laminar and disturbed flow
Blood generally flows through arteries in an orderly way, with blood in the center of the vessel moving faster than the blood at the periphery. This flow pattern is described as laminar because the movement of blood is in parallel lines. a
a The term plug flow is actually more precise for this spectral pattern, as discussed in Chapter 1 , but the term laminar is used throughout this text, in keeping with common convention.
When flow is laminar, the majority of blood cells at a given point are moving at a uniform speed, and the Doppler spectrum shows a thin line that outlines a clear space called the spectral window ( Fig. 3.7 ). The area under the window is clear and black, indicating that all the red blood cells captured within the sample volume are moving at the same speed at any given point in time.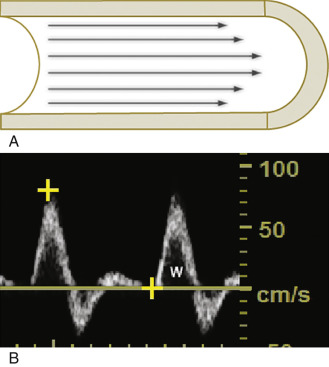
In disturbed flow , the movement of blood cells is less uniform and orderly than in laminar flow. Disturbed flow is manifested as spectral broadening or filling in of the spectral window. The degree of spectral broadening (or fill-in of the spectral window) is proportionate to the severity of the flow disturbance, as illustrated in Fig. 3.8 . Although disturbed blood flow often indicates vascular disease, it must be recognized that flow disturbances also occur in normal vessels. Kinks, curves, and arterial branching may produce flow disturbances, as illustrated quite vividly in the carotid bulb, where a prominent area of reversed flow is a normal occurrence ( Fig. 3.9 ). In addition, spectral broadening may be created in normal arteries through the use of a large sample volume that encompasses both the slow-flow area near the vessel wall and more rapid flow at the vessel center. In such cases the Doppler spectrum appears broadened because both the high-velocity flow at the vessel center and the slow flow at the periphery of the vessel are captured by the wide sample volume and then displayed.
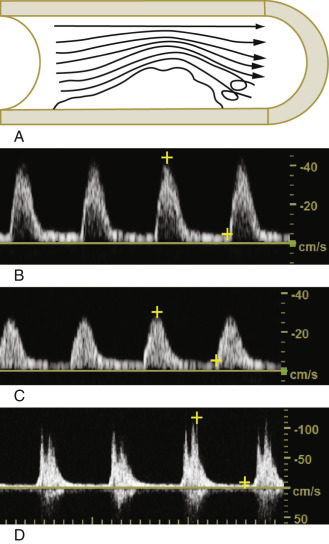
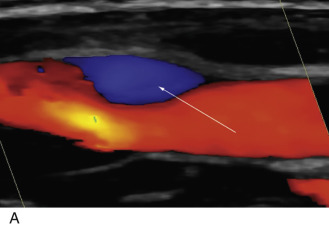
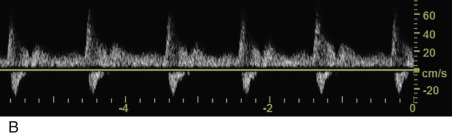
Volume flow
Modern duplex instruments are capable of measuring the volume of blood flowing through a vessel (volume flow). This is done by measuring the average flow velocity across the entire lumen (slow peripheral flow and high central flow) through several cardiac cycles while simultaneously measuring the vessel diameter, which is converted mathematically into cross-sectional area. Knowing the average velocity and the vessel area, it is easy for the Doppler instrument to estimate the blood flow (in mL/min), and this is done automatically by the ultrasound instrument. Although the ability to calculate volume flow has been available on duplex instruments for more than 20 years, issues of reproducibility have prevented volume flow measurements from being used routinely in a clinical setting. a
a References .
Volume flow measurements are currently used in evaluation of dialysis fistulae and grafts.- •
When performing pulsed Doppler sampling of a vessel, remember the three characteristics of the sample volume:
- •
It is a volume with three dimensions.
- •
The size and shape of the sample volume may be different from what is displayed on the duplex image.
- •
Doppler spectral information is obtained from the sample volume alone and not from other visible segments of the blood vessels.
- •
- •
Color flow direction is relative to the transducer and is not absolute. The apparent direction of flow can be reversed by turning the transducer around or by pressing a button on the instrument that inverts the spectrum.
- •
Proper interpretation of pulsatility requires knowledge of the normal waveform characteristics of a given vessel and the physiologic status of the circulation at the time of examination. Remember, each vascular system has a characteristic Doppler signature.
- •
In most normal situations, flow velocity in an artery accelerates very rapidly in systole and produces an almost vertical deflection of the Doppler waveform at the start of systole. If, however, severe arterial obstruction is present proximal to the point of Doppler examination, systolic flow acceleration may be slowed substantially.
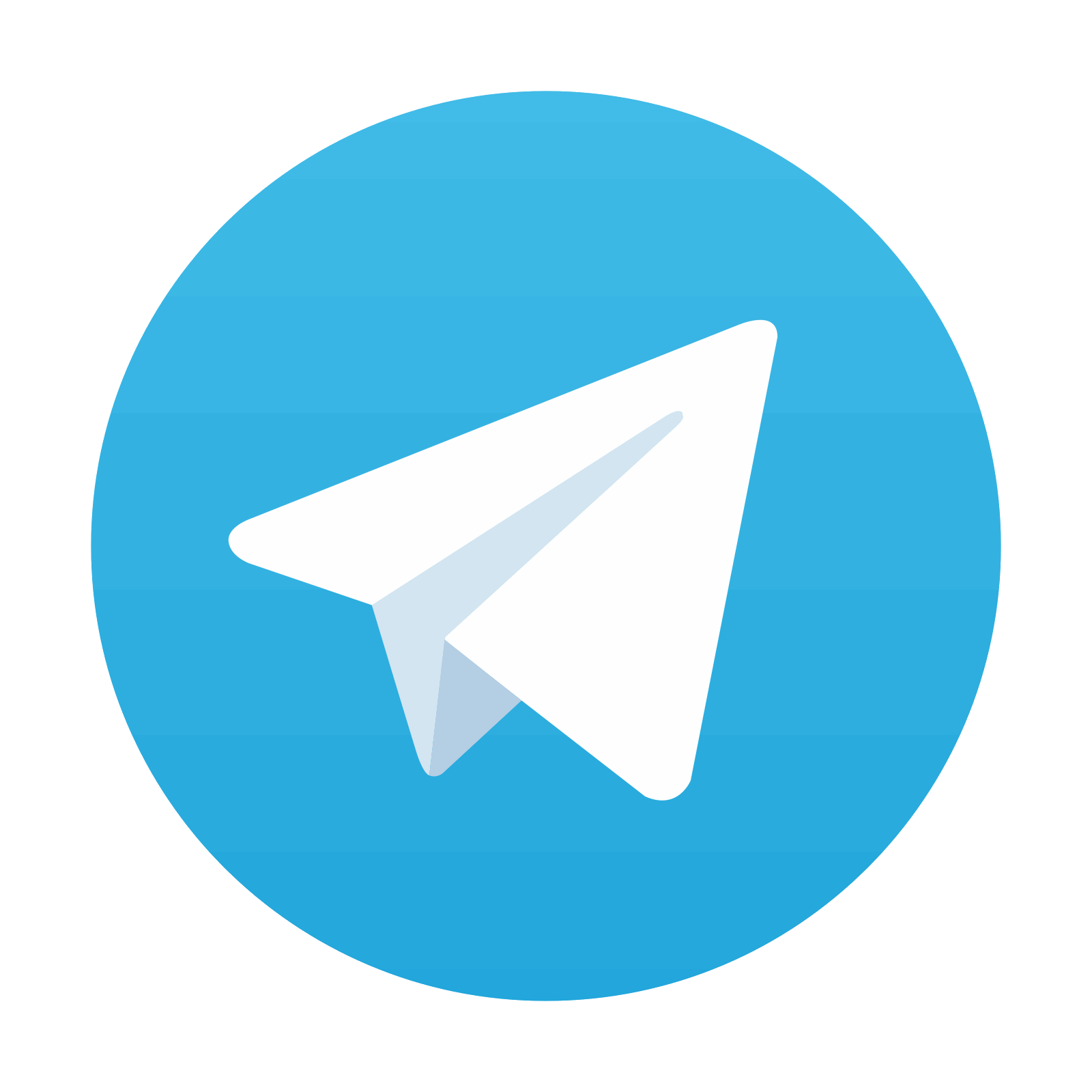
Stay updated, free articles. Join our Telegram channel

Full access? Get Clinical Tree
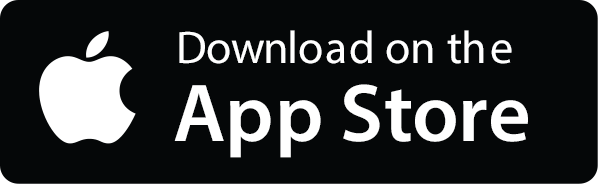
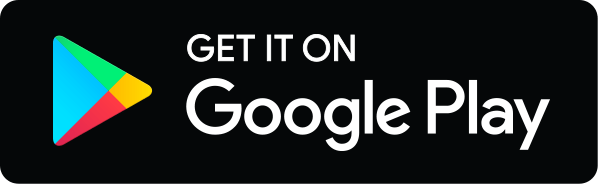
