(1)
University of Miami Sylvester Cancer Center, Miami, Florida, USA
As a dosimetrist, the basic skill is dose calculations. There are two essential categories for dose calculations: correction-based methods and model-based methods.
Correction-based methods use basic dose calculation methods based on PDD or TMR tables and then are corrected for other factors such as wedge, field size, distance, depth, inhomogeneity, etc. The main advantages for correction-based methods are that they are direct, fast, and easy to check and can be done manually. Most of the secondary MU calculation software, either commercial or customized, is correction based. However, correction-based methods can only calculate a single-point dose per calculation, which is inconvenient for volume dose calculations.
Model-based methods have become the standard dose calculation algorithm in modern treatment planning systems (TPS). Model-based methods are more accurate than correction-based methods since they consider more factors such as photon and electron scattering, secondary particle generation, and beam hardening, among others. A Monte Carlo simulation (MC) is considered as the most precise model-based method. Other well-known model-based methods include the pencil beam algorithm (PBA), the collapsed-cone convolution (CCC) by ADAC/Pinnacle, and the analytical anisotropic algorithm (AAA) by Eclipse/Varian. Model-based methods can be time consuming, but modern computing and variance reduction methods have reduced the calculation burden. The standard dose calculation algorithms in commercial TPSs calculate volume dose in which there are hundreds of points.
Most of the exam questions on dose calculations are for correction-based methods. It would be difficult to use a model-based algorithm to calculate dose on a written exam; therefore, CMD candidates only need to know the concepts and principles of model-based methods.
2.1 Dose Calculation (Questions)
Quiz 1 (Level 2)
1.
Basic dosimetry measurements are usually performed in a water phantom because:
A.
I, II, and III only
B.
I and III only
C.
II and IV only
D.
IV only
E.
All are correct
I.
Water approximates the radiation absorption and scattering properties of muscle and soft tissues.
II.
Water is universally available with reproducible radiation properties.
III.
Water is tissue equivalent.
IV.
Water has a high Z.
2.
Which of the following phantom materials is/are frequently used for radiation dosimetry?
A.
I, II, and III only
B.
I and III only
C.
II and IV only
D.
IV only
E.
All are correct
I.
Lucite
II.
Tungsten
III.
Polystyrene
IV.
Graphite
3.
A phantom with materials that simulate various body tissues like muscle, bone, lung, and air cavities is called:
A.
Homogeneous phantoms
B.
Anthropomorphic phantoms
C.
Solid water phantoms
D.
Wood phantoms
4.
The variation of absorbed dose in a patient or phantom depends on:
A.
I, II, and III only
B.
I and III only
C.
II and IV only
D.
IV only
E.
All are correct
I.
Beam energy
II.
Depth
III.
Field size and collimator
IV.
Distance from source
5.
The percentage of the absorbed dose at any depth d to the absorbed dose at a fixed reference depth d 0 along the central axis of the beam is the definition of:
A.
Mayneord F Factor
B.
Percentage depth dose
C.
Tissue-air ratio
D.
Tissue-phantom ratio
6.
Which of the following statements is/are true?
A.
I, II, and III only
B.
I and III only
C.
II and IV only
D.
IV only
E.
All are correct
I.
PDD beyond Dmax increases with beam energy.
II.
PDD decreases with depth beyond the Dmax.
III.
PDD increases with increasing field size.
IV.
PDD increases with increases SSD.
7.
The region between the surface and the point of maximum dose is called:
A.
Skin-sparing effect
B.
Dmax
C.
Dose buildup region
D.
Penumbra
8.
Match the following quality photons with its corresponding depth dose distribution.
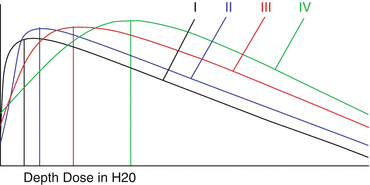
A.
25 MV (IV)
B.
4 MV (II)
C.
10 MV (III)
D.
60Co (I)
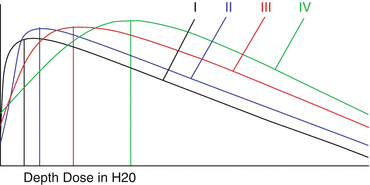
Fig. 2.1.1
9.
The projection, on a plane perpendicular to the beam axis, of the distal end of the collimator as seen from the front center of the source is the definition of:
A.
Source-to-surface distance
B.
Source-to-axis distance
C.
Dosimetric or physical field size
D.
Geometric field size
10.
Which of the following statements is/are true?
A.
I, II, and III only
B.
I and III only
C.
II and IV only
D.
IV only
E.
All are correct
I.
Rectangular field is equivalent to a square field if they have the same area/perimeter (A/P).
II.
Sterling developed the rule-of-thumb method for equating rectangular and square fields.
III.
Clarkson’s method includes irregular field dose calculations.
IV.
As the field size increases, PDD increases.
11.
Which of the following formulas is/are used to calculate equivalent square?
A.
I, II, and III only
B.
I and III only
C.
II and IV only
D.
IV only
E.
All are correct
I.
r = 4/√π × A/P
II.
ESF = 4 × A/P
III.
A/P = a/4 or A/P = a × b/2(a + b)
IV.
ESF = 2(L × W)/L + W
12.
The equivalent square formula can be used with:
A.
Circular-shaped fields
B.
Irregular-shaped fields
C.
Rectangular-shaped fields
D.
Triangular-shaped fields
13.
A PDD needs to be found for an equivalent square of a rectangular tangential breast field of 20 cm by 14 cm. Which of the following answers is the equivalent square of the above RT breast tangent field?
A.
23.33 × 23.33
B.
41.11 × 41.11
C.
16.47 × 16.47
D.
4.11 × 4.11
14.
What is the A/P of 10 × 25 cm field?
A.
35.7
B.
3.57
C.
125
D.
17.5
15.
Which of the following statements is/are true?
A.
I, II, and III only
B.
I and III only
C.
II and IV only
D.
IV only
E.
All are correct
I.
PDD data for radiation therapy beams are usually tabulated for square fields.
II.
As the field size increase, the contribution of the scattered radiation to the absorbed dose increases.
III.
The increase in PDD caused by increase in field size depends on beam quality.
IV.
The field size dependence of PDD is less pronounced for the higher-energy than for the lower-energy beams.
16.
A rectangular field is equivalent to a square field if:
A.
Both have the same area/perimeter (A/P).
B.
Both have different area/perimeter (A/P).
C.
The rectangular is twice as bigger as the square.
D.
Both have the same isocenter.
17.
Match the following answers using a rectangular field of 25 × 5 cm.
A.
Area
B.
Perimeter
C.
Area/perimeter (A/P)
D.
Equivalent square
I.
60
II.
125
III.
8.33
IV.
2.083
18.
Match the following statements. There can be more than one answer for each letter:
A.
Energy increases.
B.
Depth increases.
C.
Field size increases.
D.
SSD increases.
I.
PDD increases.
II.
PDD decreases.
III.
PDD remains the same.
19.
The increase in PDD with an increase in SSD can be found by:
A.
Mayneord F factor formula
B.
Equivalent square formula
C.
Inverse square formula
D.
Tissue-air ratio formula
20.
Which of the following is the Mayneord F factor formula?
A.
F = [(SSD2 − d m)/(SSD1 − d m) × (SSD1 − d)/(SSD2 − d)]2
B.
F = [(SSD1 + d m)/(SSD1 + d m) × (SSD2 + d)/(SSD2 + d)]2
C.
F = [(d + d m)/(SSD1 + d m) × (d + d)/(SSD2 + d m)]2
D.
F = [(SSD2 + d m)/(SSD1 + d m) × (SSD1 + d)/(SSD2 + d)]2
21.
A CNS single spine field was calculated from C3 to S1 using 100 cm SSD at a depth of 5 cm, the PDD was determine to be 80 %, and the Dmax depth was 0.5 cm. After plan revision, the physician decided to include the entire sacrum. What is the new PDD if the field was changed from 100–120 SSD?
A.
1.0144
B.
81.2 %
C.
80.6 %
D.
75.5 %
22.
Which of the following field size has the highest PDD for the same beam energy at the same depth?
A.
I, II, and III only
B.
I and III only
C.
II and IV only
D.
IV only
E.
All are correct
I.
20 × 4
II.
12 × 6
III.
6.7 × 6.7
IV.
8 × 8
23.
At a lung-tissue interface, the dose is affected primary by:
A.
Attenuation of the primary beam
B.
Scatter
C.
Mass attenuation coefficient
D.
Heterogeneity correction
24.
One method for correcting tissue inhomogeneities and use to remove the SSD dependence is:
A.
SAR
B.
Clarkson’s method
C.
TAR
D.
TMR
I do not like this question. It is introducing two very different concepts of TAR, and the answer does not fully explain them. I would remove this question. The important point about the SSD independence of TAR is addressed in a few questions.
25.
The TAR is calculated by finding:
A.
The ratio of dose at depth in phantom to dose at a specified reference depth in phantom
B.
The ratio of dose at depth in phantom to dose in free space at the same point
C.
The ratio of dose at depth in phantom to dose at a depth of maximum dose in phantom
D.
The ratio of dose at maximum dose in phantom to dose in free space at the same point
26.
TAR depends on:
A.
I, II, and III only
B.
I and III only
C.
II and IV only
D.
IV only
E.
All are correct
I.
Field size
II.
Depth
III.
Energy
IV.
SSD
27.
The difference between TAR and BSF is:
A.
TAR is defined at Dmax only, and BSF is defined at any depth.
B.
TAR is defined at any depth, and BSF is defined at Dmax only.
C.
TAR and BSF are measured at a point in air.
D.
TAR and BSF are independent of distance.
28.
TAR increases as:
A.
I, II, and III only
B.
I and III only
C.
II and IV only
D.
IV only
E.
All are correct
I.
Energy increases.
II.
Depth decreases.
III.
Field size increases.
IV.
Field size decreases.
29.
Determine the treatment time to deliver 150 cGy (rad) at the center of rotation of an arc treatment where the dose rate in free space at SAD is 80.5 cGy/min and the average TAR is 0.550.
A.
3 min
B.
3.39 cGy/min
C.
3.39 min
D.
5 min
30.
Which of the following statements about scatter-air ratio (SAR) is/are true?
A.
I, II, and III only
B.
I and III only
C.
II and IV only
D.
IV only
E.
All are correct
I.
SAR is defined as the ratio of the scattered dose at a given point in the phantom to the dose in free space at the same point.
II.
SAR is used for the purpose of calculating scattered dose in the medium.
III.
SAR in the phantom is equal to the total dose minus the primary dose at that point.
IV.
SAR is the difference between the TAR for a given field and the TAR for the 0 × 0 field.
31.
SAR depends on:
A.
I, II, and III only
B.
I and III only
C.
II and IV only
D.
IV only
E.
All are correct
I.
Beam energy
II.
Depth
III.
Field size
IV.
SSD
32.
The SAR formula is:
A.
SAR = TAR(d, rd) + TAR(d, 0)
B.
SAR = TAR(d, rd) − TAR(d, 0)
C.
SAR = TAR(d, rd)/TAR(d, 0)
D.
SAR = TAR(d, rd) × TAR(d, 0)
33.
Match the correct name with the corresponding diagram.
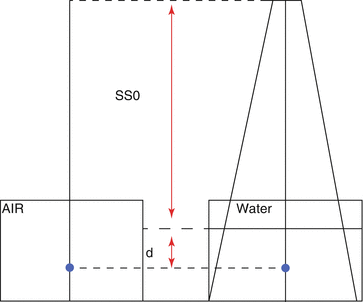
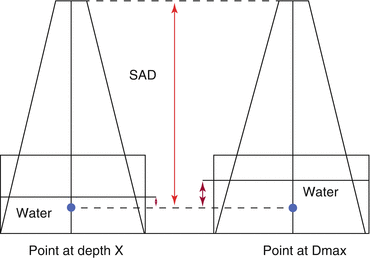
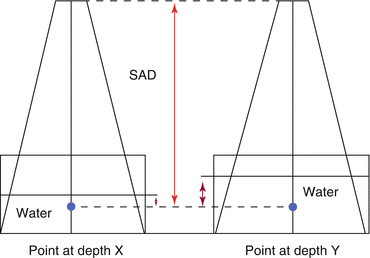
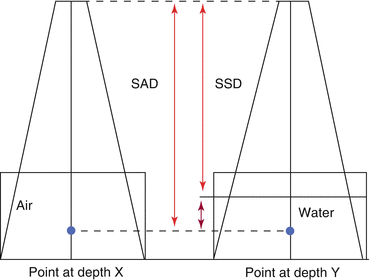
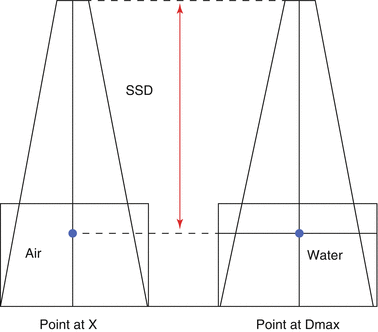
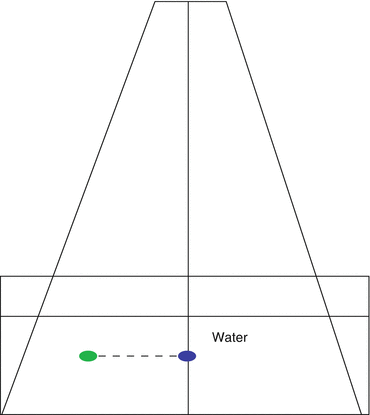
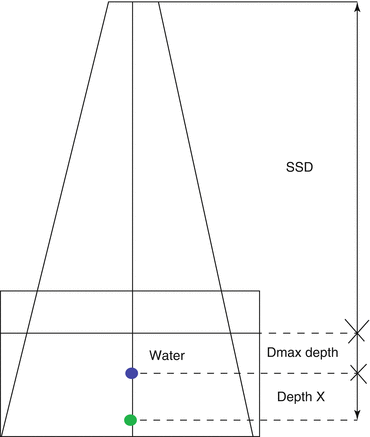
A.
TMR
B.
PDD
C.
SAR
D.
TAR
E.
TPR
F.
Off-axis ratio
G.
BSF
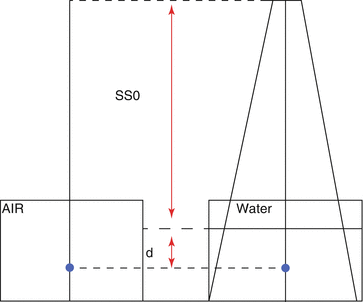
Fig. 2.1.2
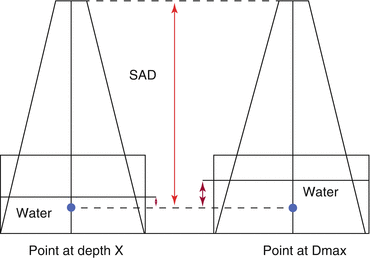
Fig. 2.1.3
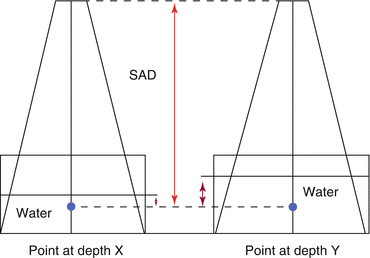
Fig. 2.1.4
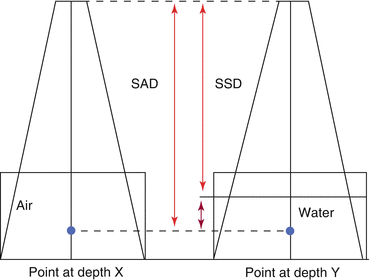
Fig. 2.1.5
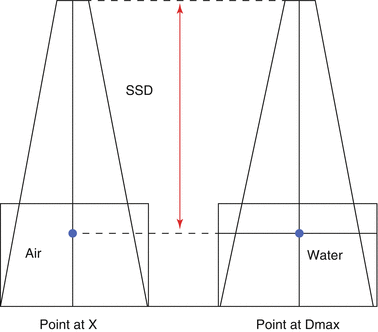
Fig. 2.1.6
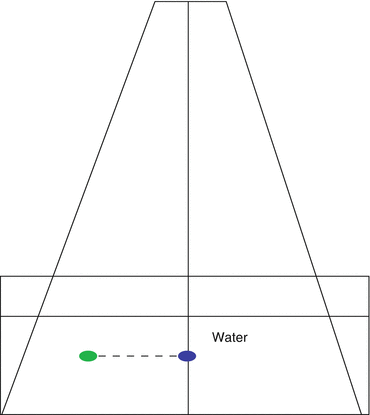
Fig. 2.1.7
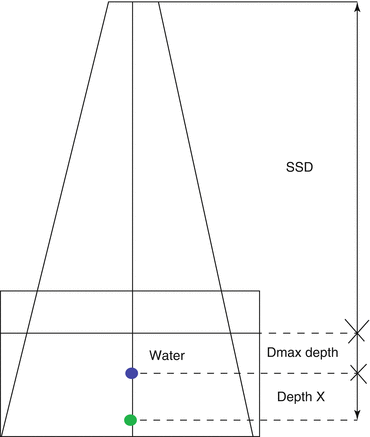
Fig. 2.1.8
34.
An irregular field is:
A.
I, II, and III only
B.
I and III only
C.
II and IV only
D.
IV only
E.
All are correct
I.
Any rectangular or circular field
II.
Any blocked or rectangular field
III.
Any square and rectangular field
IV.
Any field other than the rectangular, square, or circular field
35.
Which of the following data is/are used in the calculation of Clarkson’s method?
A.
I, II, and III only
B.
I and III only
C.
II and IV only
D.
IV only
E.
All are correct
I.
SAR
II.
PDD
III.
TAR (0)
IV.
TMR
36.
TAR at Dmax is equal to:
A.
PDD
B.
BSF
C.
TPR
D.
SAR
37.
The interrelationship between TAR and PDD is:
A.
TAR = BSF × PDD/inverse square
B.
TAR = PDD × BSF × inverse square
C.
PDD = TAR × inverse square
D.
TAR = TMR × BSF/inverse square
38.
Limitations of PDD and TAR include:
A.
I, II, and III only
B.
I and III only
C.
II and IV only
D.
IV only
E.
All are correct
I.
PDD depends on SSD only.
II.
PDD depends on SAD only.
III.
TAR cannot be used on higher-energy photons.
IV.
TAR cannot be used on lower energies such as 60Co.
39.
The dose to a point in the medium may be calculated using:
A.
Primary and scattered radiation
B.
Compton and pair production
C.
Scatter and neutron radiation
D.
Photoelectric and coherent scatter
40.
Effective primary dose is:
A.
Dose due to the primary photons only
B.
Dose scatters produced by the patient
C.
Dose scatters produced by the collimating system
D.
Dose due to primary photons as well as those scattered from the collimating system
41.
The most commonly used beam-modifying device is:
A.
Flattening filter
B.
The anode
C.
Bending magnet
D.
A wedge
42.
A wedge angle refers to:
A.
The angle between the central axes of the two beams
B.
The angle of the isodose tilt at Dmax
C.
The angle through which an isodose curve is tilted at the central axis of the beam at a specified depth
D.
The physical degree angle of the wedge
43.
Which of the following wedge types should be avoided in the medial tangent of a breast treatment?
A.
I, II, and III
B.
I and III only
C.
II and IV only
D.
IV only
E.
All are correct
I.
Dynamic wedge
II.
Asymmetric wedge
III.
Universal wedge
IV.
Physical wedge
44.
Physical wedge should be avoided, if possible, in the medial field of a tangent breast treatment:
A.
Because physical wedge can fall and harm the patient
B.
Because physical wedge produces scatter which affect the contralateral breast
C.
Because the physical wedge is too close to the patient
D.
Because wedge factor is difficult to calculate on the medial tangent
45.
What happens to the wedge angle or tilt of the isodose curve as depth is increased?
A.
Increases
B.
Decreases
C.
Stays the same
D.
Depth has no effect on the isodose curve
46.
The wedge transmission factor expresses:
A.
The ratio of the dose rate at Dmax with and without the wedge
B.
The ratio of the dose rate on the central axis at specified depth with and without the wedge
C.
The dose rate on the central axis with the wedge
D.
The dose rate at Dmax with the wedge on in-out position
47.
The thicker end of a wedge is called:
A.
The toe
B.
The heel
C.
The compensator
D.
The grow
48.
Wedges are typically used:
A.
I, II, and III
B.
I and III only
C.
II and IV only
D.
IV only
E.
All are correct
I.
On sloped surfaces such as AP chest fields
II.
On tangential breast treatments
III.
On lateral larynx treatments
IV.
On a PA lumbar spine
49.
Which of the following wedges is designed so that the central axis thickness remains constant and the center of the wedge is fixed in the center of the beam for all field sizes?
A.
Dynamic wedge
B.
Universal wedge
C.
Asymmetric wedge
D.
Individualized wedge
50.
Which of the following definitions are true?
A.
I, II, and III only
B.
I and III only
C.
II and IV only
D.
IV only
E.
All are correct
I.
Output of a machine is defined as the dose delivered at a specified point in the beam, at a specified distance from the target, and in a specified medium.
II.
Absorbed dose in the irradiated medium is the energy absorbed per unit mass.
III.
Maximum dose or Dmax is obtained at the depth where electron equilibrium is reached.
IV.
The buildup region is the region between the surface and Dmax.
51.
Which of the following is/are true about half-value layer (HVL)?
A.
I, II, and III only
B.
I and III only
C.
II and IV only
D.
IV only
E.
All are correct
I.
The HVL is the thickness of the material that reduces the intensity of the beam to half (50 %) its original value.
II.
The HVL expresses the penetration, quality, or hardness of a beam.
III.
If the HVL of a given beam is 5 mm Cu, inserting 5 mm Cu in the beam path will reduce its intensity to half of its original value.
IV.
The HVL is related to the linear attenuation coefficient (μ) by the equation (HVL = 0.693/μ).
52.
Which of the following is/are true about dose rate?
A.
I, II, and III only
B.
I and III only
C.
II and IV only
D.
IV only
E.
All are correct
A.
Dose rate increases with increasing field size.
B.
Dose rate decreases with increasing field size.
C.
Dose rate varies inversely proportional with the square of the distance from the source.
D.
Dose rate increases as distance from the source increase.
53.
Which of the following materials is used for the measurement of HVL?
A.
Aluminum (Al), Cerrobend, or tin (Sn)
B.
Aluminum (Al), copper (Cu), or tungsten
C.
Lipowitz, copper (Cu), or tin (Sn)
D.
Aluminum (Al), copper (Cu), or tin (Sn)
54.
For a monoenergetic beam:
A.
HVL1 > HVL2
B.
HVL1 < HVL2
C.
HVL1 = HVL2
D.
HVL1 ≤ HVL2
55.
For a polyenergetic beam:
A.
HVL1 > HVL2
B.
HVL1 < HVL2
C.
HVL1 = HVL2
D.
HVL1 ≤ HVL2
56.
For monoenergetic photon beams, the linear attenuation coefficiency formula is:
A.
HVL × 0.693
B.
0.693/HVL
C.
λ = −0.693/T½
D.
T a = 1.44 × T 1/2
57.
If the linear attenuation coefficient for a 23 MV photon beam is 0.046 mm−1 lead, what is the HVL thickness?
A.
0.03 mm lead
B.
15 mm lead
C.
0.066 mm lead
D.
10 mm lead
58.
Match the following definitions.
A.
Tissue maximum ratio (TMR)
B.
Tissue-phantom ratio (TPR)
C.
Backscatter factor (BSF)
D.
Scatter-air ratio (SAR)
I.
The ratio of dose at a specified point in tissue or in a phantom to the dose at the same distance in the beam at a reference depth
II.
The ratio of the dose at a specified point in tissue or in a phantom to the dose at the same point when it is at the depth of maximum dose
III.
Scattered dose at a given point in a medium to the dose in air at the same point
IV.
The ratio of dose on the central axis at Dmax to the dose at the same point in air or free space
59.
TMR is a special case of:
A.
TPR
B.
SAR
C.
SMR
D.
Backscatter factor
60.
Which of the following statements is/are true?
A.
I, II, and III only
B.
I and III only
C.
II and IV only
D.
IV only
E.
All are correct
I.
The difference between SAR and SMR is that SMR reference point is at Dmax only versus SAR reference point is at any given point.
II.
The difference between TAR and TMR is that TMR reference point is at Dmax only versus TAR reference point is at any given point.
III.
SAR and TAR are independent of distance.
IV.
SAR and SMR are mainly used in calculation of scattered dose in a phantom or tissue.
61.
Methods of inhomogeneity corrections for photon beams are:
A.
I, II, and III only
B.
I and III only
C.
II and IV only
D.
IV only
E.
All are correct
I.
TAR
II.
Effective SSD method
III.
Isodose shift method
IV.
Coefficient of equivalent thickness
62.
How many HVLs are required to reduce a photon beam of 320 mR/h to 5 mR/h?
A.
5 HVLs
B.
7 HVLs
C.
6 HVLs
D.
12 HVLs
63.
How many TVLs are required to reduce a photon beam of 4,000 mR/min to 4 mR/min?
A.
3 HVLs
B.
5 HVLs
C.
7 HVLs
D.
12 HVLs
64.
Match the beams with its corresponding characteristics (monoenergetic or polyenergetic beam).
A.
Photon beam from a diagnostic or therapeutic device
B.
Electron beam
C.
Gamma rays
D.
Characteristic x-rays
I.
Monoenergetic beam
II.
Polyenergetic beam
65.
Match the following reduction and/or increase of dose from each photon beam energy due to the presence of bone or healthy lung in the irradiated area.
A.
3 mm Cu
B.
60Co
C.
4 MV
D.
10 MV
E.
20 MV
I.
2 %/cm increase in dose beyond healthy lung
II.
−3 % reduction of dose beyond bone or 4 %/cm increase in dose beyond healthy lung
III.
−3.5 % reduction of dose beyond bone or 3.5–5/cm % increase in dose beyond healthy lung
IV.
−2 % reduction of dose beyond bone
V.
−7 % reduction of dose beyond bone or 10 %/cm increase in dose beyond healthy lung
66.
A test has been conducted with a material having a HVL of 3.5 cm. What will be the transmission of the beam through the same material with a thickness of 10.5 cm?
A.
0.0007
B.
0.125
C.
0.088
D.
0.795
67.
After 10 HVLs, the intensity of the beam is reduced by:
A.
I = I 0(0.1)10
B.
I = I 0(0.5)10
C.
I 10 = I 0(0.5)
D.
I = I 0(10)10
68.
The collimator scatter factor (Sc):
A.
I, II, and III only
B.
I and III only
C.
II and IV only
D.
IV only
E.
All are correct
I.
Is commonly called the output factor.
II.
Is the ratio of the output in air for a given field to that for a reference field.
III.
As the field size increased, Sc or output factor increases.
IV.
Is the ratio of the output in the tissue for a given field to that for a reference field.
69.
The phantom scatter factor (Sp):
A.
I, II, and III only
B.
I and III only
C.
II and IV only
D.
IV only
E.
All are correct
I.
Takes into account the change in scatter radiation originating in the phantom at a reference depth as the field size is changed.
II.
Is the ratio of the dose rate for a given field at a reference depth to the dose rate at the same depth for the reference field size, with the same collimator opening.
III.
Is related to changes in the volume of the phantom irradiated for a fixed collimator opening.
IV.
If backscatter factors can be measured, Sp at Dmax may be defined as the ratio of BSF for the given field to that for the reference field.
70.
Clarkson’s method is used to calculate:
A.
Circular fields
B.
Irregular fields
C.
Rectangular fields
D.
Square fields
71.
The relationship between TMR and TAR is:
A.
TMR(d, rd) = BSF(rd)/TAR(d, rd)
B.
TMR(d, rd) = BSF(rd) × TAR(d, rd)
C.
TMR(d, rd) = TAR(d, rd)/BSF(rd)
D.
TMR(d, rd) = SAR(rd)/TAR(d, rd)
72.
Scatter-maximum ratio (SMR):
A.
I, II, and III only
B.
I and III only
C.
II and IV only
D.
IV only
E.
All are correct
I.
Is the quantity designated specifically for the calculation of scattered dose in a medium
II.
Is the ratio of the scattered dose at a given point in the phantom to the effective primary dose at the same point at Dmax
III.
Is related to SAR
IV.
Is the ratio of the scattered dose at a given point in the phantom to the effective primary dose at any point
73.
Which of the following statements is/are true about the backscatter factor?
A.
I, II, and III only
B.
I and III only
C.
II and IV only
D.
IV only
E.
All are correct
I.
BSF is independent of SSD.
II.
BSF depends on energy and the field size.
III.
BSF and TAR at Dmax are the same.
IV.
BSF is much lower for megavoltage beams than lower-energy beams.
74.
Match the formulas with their corresponding definitions.
A.
PDD
B.
TMR
C.
TAR
D.
TPR
E.
BSF
F.
Output
G.
SAR
H.
Absorbed dose
I.
Collimator scatter factor (Sc)
J.
Phantom scatter factor (Sp)
K.
SMR
I.
Ratio of dose at depth in a phantom to dose at a depth of Dmax in a phantom. TAR(d, rd)/BSF(rd). Dose in tissue/dose in phantom at Dmax
II.
Percentage of the absorbed dose at any depth d to the absorbed dose at a fixed reference depth d 0, along the central axis of the beam
III.
Ratio of dose at depth in phantom to dose in free space at the same point. BSF × PDD/inverse square. Dose in tissue/dose in air
IV.
Ratio of dose at maximum dose in phantom to dose in free space at the same point or tissue-air ratio at the depth of maximum dose on central axis of the beam. Dose at Dmax/dose in free space
V.
Ratio of dose at depth in phantom to dose at a specified reference depth in phantom. Dose in tissue/dose in phantom at reference depth
VI.
Dose delivered at a specified point in the beam, at a specified distance from the target, and in a specified medium
VII.
Energy absorbed in a material per unit mass
VIII.
Ratio of scattered dose at a given point in the phantom to the dose in free space at the same point. TAR(d, rd)−TAR(d, 0)
IX.
Ratio of the output in air for a given field to that for a reference field. Also called the output factor. D f s (r)/D f s (ref)
X.
Ratio of the scattered dose at a given point in the phantom to the effective primary dose at the same point at Dmax
XI.
Ratio of the dose rate for a given field at a reference depth to the dose rate at the same depth for the reference field size, with the same collimator opening
75.
The dose on a fixed SAD technique is usually normalized at:
A.
The surface of the patient
B.
The isocenter
C.
At Dmax
D.
The tissue surrounding the target
76.
Which of the following statements is/are true?
A.
I, II, and III only
B.
I and III only
C.
II and IV only
D.
IV only
E.
All are correct
I.
The SAD technique is also known as an isocentric technique.
II.
The axis of rotation of the machine is the isocenter.
III.
Small errors in SSD are balanced out using an opposing field.
IV.
SAD technique is inferior to an SSD technique.
77.
Which of the following methods are used in calculating the dose in an SAD technique?
A.
I, II, and III only
B.
I and III only
C.
II and IV only
D.
IV only
E.
All are correct
I.
TAR and dose rate in air
II.
PDD
III.
TPR and TMR
IV.
Clarkson’s method
78.
Which of the following is/are correct about the isocenter?
A.
I, II, and III only
B.
I and III only
C.
II and IV only
D.
IV only
E.
All are correct
I.
Refers to a point in space that is the same distance from the source for all gantry angles.
II.
Is the intersection of the collimator axis and the axis of rotation.
III.
Linacs have the isocenter typically at 80 or 100 cm.
IV.
The isocenter is fixed and cannot be changed.
79.
The dose on a fixed SSD technique is usually normalized at:
A.
The surface of the patient
B.
The isocenter
C.
At Dmax
D.
The tissue surrounding the target
80.
Which of the following statements is/are true about SSD?
A.
I, II, and III only
B.
I and III only
C.
II and IV only
D.
IV only
E.
All are correct
I.
SSD is patient specific.
II.
Field size is defined on the patient’s skin on an SSD setup.
III.
SSD is a non-isocentric technique.
IV.
SSD calculation uses the PDD method.
81.
If an SAD technique is used for treatment, the SAD is equal to:
A.
SSD – depth
B.
SSD + depth
C.
SSD/depth
D.
SSD × depth
82.
Machines are usually calibrated:
A.
To deliver 1 cGy per monitor unit (MU) at 100 cm SSD, the reference depth, for a maximum open field 40 × 40 cm
B.
To deliver 1 cGy per monitor unit (MU) at 100 cm SSD, the reference depth, for a reference field size 10 × 10 cm
C.
To deliver 1 cGy per monitor unit (MU) at 100 cm SSD, the reference depth, for a reference field size, usually 10 × 10 cm
D.
To deliver 100 cGy per monitor unit (MU) at 100 cm SAD, for a reference field size, usually 10 × 10 cm
83.
An isodose line is defined as:
A.
A line passing through points of equal dose representing a percentage of dose at a reference point
B.
A region near the edge of the field margin where the dose falls rapidly
C.
A tilting of the isodose lines from their standard position
D.
A line passing through points of different dose and representing percentage of dose at a reference point
84.
A number of isodose curves depicted in 10 % increments is a function of:
A.
Isodose line
B.
Isodose chart
C.
DVH
D.
BEV
85.
The light source, which coincides with the beam, is aligned to match:
A.
100 % isodose line
B.
75 % isodose line
C.
50 % isodose line
D.
25 % isodose line
86.
Geometric field size is defined as:
A.
The intersection of the 50 % isodose line and the surface
B.
The intersection of the collimator axis and the axis of rotation
C.
The region between the surface and maximum dose or Dmax
D.
Region near the edge of the field margin, where the dose falls rapidly
87.
The size of the penumbra depends on:
A.
I, II, and III only
B.
I and III only
C.
II and IV only
D.
IV only
E.
All are correct
I.
Size of the radiation source
II.
Source-to-collimator distance (SCD)
III.
Source-to-skin distance (SSD)
IV.
Depth of penumbra calculation
88.
Which of the following statements is/are true?
A.
I, II, and III only
B.
I and III only
C.
II and IV only
D.
IV only
E.
All are correct
I.
Isodose chart for a Co60 machine dose decreases away from the central axis near the surface.
II.
Isodose chart for a linac machine dose increases away from the central axis near the surface.
III.
Increased dose away from the central axis near the surface is due to overflattening of the beam at shallow depths.
IV.
The flattening filter reduces the dose along the central axis and produces a flat beam at a specified depth, usually 10 cm.
89.
Which of the following statements is/are true?
A.
I, II, and III only
B.
I and III only
C.
II and IV only
D.
IV only
E.
All are correct
I.
For an SSD technique, dose at Dmax is usually normalized to 100 %.
II.
For an SAD technique, dose at isocenter is usually normalized at 100 %.
III.
Dose profile displays relative doses across a field or across a treatment plan consisting of multiple beams.
IV.
A beam’s eye view shows the beam in a plane perpendicular to the central axis.
90.
Match the following wedge types with its corresponding characteristic.
A.
Dynamic wedge
B.
Universal wedge
C.
Asymmetric wedge
D.
Individualized wedge
I.
Wedge of a given angle that is fixed in the beam and serves all beam widths up to a designated limit.
II.
Multiple wedges are required for each isodose tilt and are typically used in Co-60 beams. This wedge is mounted so that the thin edge of the wedge coincides with the edge of the light field.
III.
Wedge effect can be produced by driving one of the secondary collimator jaws across the field.
IV.
A wedge is mounted such that one can use an asymmetric jaw to block half the treatment field and utilize the wedging effect for the other open half.
91.
The angle of the sloping isodose curve on a dynamic wedge is determined by:
A.
The speed with which the gantry moves
B.
The dose rate
C.
The speed with which the collimator jaw moves
D.
The amount of monitor units delivered
92.
What is the distance in cm that a block or wedge should be placed away from the patient skin?
A.
At least 10 cm away from the skin.
B.
It could be placed as close as 1 cm away from the skin.
C.
The distance is not important.
D.
At least 15 cm away from the skin.
93.
A wedge is usually constructed of:
A.
I, II, and III
B.
I and III only
C.
II and IV only
D.
IV only
E.
All are correct
I.
Lead
II.
Steel
III.
Copper
IV.
Tungsten
94.
Which of the following statements is/are true about a dynamic wedge?
A.
I, II, and III
B.
I and III only
C.
II and IV only
D.
IV only
E.
All are correct
I.
Can be produced by driving one of the collimator jaws across the field
II.
Can generate different wedge angles
III.
Has different transmission factors as a function of field size
IV.
Has a sharper penumbra
95.
Which of the following techniques can be used to create the junction between the cranial field and the upper spine field for craniospinal irradiation?
A.
I, II, and III
B.
I and III only
C.
II and IV only
D.
IV only
E.
All are correct
I.
Using a gap on the skin
II.
Using independent jaws or half-beam block
III.
Using collimator and couch angles
IV.
Positioning the patient in a Trendelenburg or decubitus position
96.
The advantage of using independent table and collimator angles to match the craniospinal fields is:
A.
I, II, and III
B.
I and III only
C.
II and IV only
D.
IV only
E.
All are correct
I.
Field matching is achieved with no overlap between the cranial and spine fields at any depth.
II.
Patient’s comfort and reproducibility are better.
III.
The independent jaw can be conveniently used to move the craniospinal junction line caudally to smooth out the junction dose distribution.
IV.
The independent jaw can be conveniently used to move the craniospinal junction line cephalic to smooth out the junction dose distribution.
97.
Which of the following is/are true about field matching?
A.
I, II, and III
B.
I and III only
C.
II and IV only
D.
IV only
E.
All are correct
I.
The site of the field matching should not contain tumor or a critically sensitive organ.
II.
For deep-seated tumors, the fields may be separated on the skin surface.
III.
The line of field matching must be de drawn at each treatment session on the basis of the first treated fraction.
IV.
The field-matching technique must be verified by actual isodose distributions before it is adopted for general clinical use.
98.
What will be the outcome of the HVL if a machine is assigned a HVL of 3 mm Cu, but the engineer accidentally inserts a 3.5 mm Cu HVL filter?
A.
HVL will decrease.
B.
HVL will increase.
C.
HVL will remain unchanged.
D.
HVL will increase at extended SSDs only.
99.
The International Commission on Radiological Units and Measurements (ICRU) recommends that dose delivered to a tumor to be:
A.
Within 5.0 % of the prescribed dose
B.
Within 8.0 % of the prescribed dose
C.
Within 10.0 % of the prescribed dose
D.
Within 15.0 % of the prescribed dose
2.2 Applied Mathematics (Questions)
Quiz 1 (Level 1)
1.
Match the following problems with its corresponding answer.
A. | 155 × 103 | I. | 3 |
B. | 1.5 × 1210 × 2.5 × 107 | II. | 8,000 |
C. | 203 | III. | 4.517 |
D. | √25 | IV. | 4 |
E. | 3√27 | V. | 759,375,000 |
F. | √16 | VI. | 5 |
2.
Match the following metric system with its corresponding equivalent.
A. | 1012 | I. | 0.1 |
B. | 103 | II. | 1000 |
C. | 10−9 | III. | 0.000 000 001 |
D. | 101 | IV. | 1 000 000 000 000 |
E. | 10−6 | V. | 0.000 001 |
3.
Match the following metric system with its corresponding equivalent.
A. | 10−6 | Pico |
B. | 10−2 | Centi |
C. | 10−12 | Mega |
D. | 106 | Micro |
E. | 109 | Giga |
4.
Match the following metric system with its corresponding equivalent.
A. | 1015 | One thousandth |
B. | 102 | One tenth |
C. | 10−1 | One quadrillionth |
D. | 10−3 | One hundred |
E. | 1012 | One trillion |
5.
Match the following problems with its corresponding hot or cold doses.
A. | Rx = 30 Gy: 15 % of 30 Gy | I. | 0.40 |
B. | Rx = 40 Gy: 95 % of 40 Gy | II. | 32.4 Gy |
C. | Rx = 46 Gy: 90 % of 36 Gy | III. | 15 |
D. | Rx = 30 Gy: 10 % hot spot | IV. | 0.05 |
E. | Rx = 30 Gy: 10 % reduction from 30 Gy | V. | 33 Gy |
F. | Rx = 60 Gy: What % of 60 Gy is 9 Gy? | VI. | 38 Gy |
G. | Decimal equivalent of 40 % | VII. | 0.50 |
H. | Decimal equivalent of 50 % | VIII. | 27 Gy |
I. | Decimal equivalent of 5 % | IX. | 4.5 Gy |
6.
An ENT plan was given to the dosimetrist. The Rx is 60 Gy, and the dose goal states that 95 % of the PTV should receive 100 % of the Rx dose and no more than 115 % of the Rx dose is accepted. Also, 107 % of the Rx dose should cover less than 10 % of the volume, and 110 % of the Rx dose should cover less than 5 % of the volume. Which of the following answer is correct?
A.
V95 % = 60 Gy; max dose = 69 Gy; V10 % =42 Gy; V5 % = 6.6 Gy
B.
V95 % = 60 Gy; max dose = 96 Gy; V10 % = 66 Gy; V5 % = 64.2 Gy
C.
V95 % = 66 Gy; max dose = 96 Gy; V10 % =64.2 Gy; V5 % = 66 Gy
D.
V95 % = 60 Gy; max dose = 69 Gy; V10 % = 64.2 Gy; V5 % =66 Gy
7.
Match the following with its corresponding answer.
A. | sin 20° | I. | 84.3 |
B. | cos 30° | II. | 0.34 |
C. | tan 50° | III. | 0.40 |
D. | sin−1 0.10 | IV. | 36 |
E. | cos−1 0.5 | V. | 60 |
F. | tan−110° | VI. | 0.87 |
G. | sin 25° + cos 20° | VII. | 5.7 |
H. | tan−1 0.15 + cos−1 0.10 | VIII. | 1.19 |
I. | sin−10.8 – tan−10.3 | IX. | 92.8 |
8.
Match the following with its corresponding answer.
A. | e3 | I. | 0.00001 |
B. | Ln(30) | II. | 20 |
C. | Log(150) | III. | 2.18 |
D. | 1017 | IV. | 3.40 |
E. | 10−5 | V. | 10,000,000 |
9.
Convert the following.
A. | 100 Gy to cGy | I. | 1.2 |
B. | 10 cGy to Gy | II. | 3,000 |
C. | 120 rad to Gy | III. | 10,000 |
D. | 30 Gy to rad | IV. | 0.1 |
10.
Convert the following meter, cm, mm, inches, feet, °F, and °C and match to the corresponding number.
A. | 10 m to cm | I. | 30 |
B. | 550 cm to meters | II. | 0.3937 |
C. | 120 cm to mm | III. | 8 |
D. | 300 mm to cm | IV. | 24.8 |
E. | 15 in. to cm | V. | 38.1 |
F. | 10 mm to inches | VI. | 240 |
G. | 20 ft to inches | VII. | 1,000 |
H. | 46.5 °F to °C | VIII. | 1,200 |
I. | −4 °C to °F | IX. | 5.5 |
11.
A PA field is clinically set up at 70 SSD using 20 × 25 cm on the skin, but the whole treatment area is not included, and the SSD needs to be extended to 95 cm. What is the new field size on the skin at 95 cm SSD?
A.
34 × 27 cm
B.
27 × 34 cm
C.
332.5 × 266 cm
D.
45 × 50 cm
12.
A femur is set up AP/PA to cover a prosthesis that lies 10 cm from the anterior surface and 5 cm from the posterior surface. Assume that this means the separation is 15 cm and the treatment is 100 cm SAD with the isocenter placed on the prosthesis. Both AP/PA fields are 30 × 7 cm. What is the field size on the anterior and posterior skin?
A.
AP = 28.5 × 6.65 cm; PA = 27 × 6.3 cm
B.
AP = 6.65 × 6.3 cm; PA = 28.5 × 2.7 cm
C.
AP = 27 × 6.3 cm; PA = 28.5 × 6.65 cm
D.
AP = 10 × 15.3 cm; PA = 25 × 7 cm
13.
A tumor 10 cm deep needs to be treated using 100 cm SSD, 25 × 20 cm field size on the tumor. If a 1 cm bolus is placed on the skin what will be the new field size on the tumor?
A.
25 × 20 cm
B.
20.45 × 16.36 cm
C.
22.5 × 18 cm
D.
30.5 × 24.4 cm
14.
Calculate the SSD required if a TBI patient needs to be set up using at least 1 cm flash on a patient who is 5 ft and 7 in. tall by 18 in. wide, but the maximum field size at machine isocenter (100 cm) is 40 × 11.5 cm.
A.
170.5 cm SSD
B.
174 cm SSD
C.
432.5 cm SSD
D.
400 cm SSD
15.
A treatment setup is done at 100 SSD using a 110 mm × 150 mm on the skin, what is the field size 25 in. below the surface where the tumor lies?
A.
125 mm × 187.5 mm
B.
179.3 mm × 245.25 mm
C.
179.3 cm × 245.25 cm
D.
125 cm × 187.5 cm
16.
Calculate the magnification factor to construct a block if the field size at 100 cm SAD is 13 × 15 cm and the image projection on the film is 15.6 × 18 cm.
A.
0.833
B.
28.6
C.
2.6
D.
1.2
17.
An HDR cylinder measuring 12 × 2 cm is placed in the patient’s vagina at a depth of 5 cm. The patient is set up at 80 cm SSD, and the film is placed at 130 cm from the source. Calculate the size of the cylinder image on the film along with the magnification factor.
A.
18.4 cm × 3.05 cm image size and 1.5 magnification
B.
18.4 cm × 3.05 cm image size and 0.65 magnification
C.
19.5 cm × 3.25 cm image size and 1.5 magnification
D.
920 cm × 5,525 cm image size and 1.5 magnification
18.
Calculate the size of the field on a film if the field size on the skin measures 20 × 15 cm and the magnification factor is 1.45.
A.
21.7 × 29 cm
B.
29 × 21.75 cm
C.
21.45 × 16.45 cm
D.
13.8 × 10.34 cm
19.
Get Clinical Tree app for offline access
Which of the following statements is/are correct about patient anatomical orientation using Cartesian coordinates in radiation therapy?
