Fig. 17.1
Three common variations of sporadic Creutzfeldt–Jakob disease presentation on MRI. (a) Neocortical (solid arrow), limbic (dashed arrow), and subcortical gray matter hyperintensities (dotted arrow) on diffusion weighted imaging (DWI) and fluid attenuated inversion recovery (FLAIR) scans. (b) Neocortical and limbic cortex involvement. (c) Limbic and subcortical involvement. Note that the DWI shows the hyperintensities much more than the corresponding FLAIR sequences, and that DWI hyperintensities often have corresponding apparent diffusion coefficient (ADC) hypointensity. ADC abnormalities are most easily identified in the basal ganglia. [Reprinted from Vitali, P., E. Maccagnano, et al. (2011). “Diffusion-weighted MRI hyperintensity patterns differentiate CJD from other rapid dementias.” Neurology 76(20): 1711–9. With permission from Wolters Kluwer Health.]
To our knowledge, only one study evaluated the relationship between DWI alterations and the severity of the clinical course in nine patients with sCJD [21], demonstrating that patients harboring hyperintensities in both the cortex and basal ganglia experience a significantly shorter interval from disease onset to akinetic mutism than those with only cortical ribbon hyperintensity.
Cortical and basal ganglia degeneration is an important feature of CJD pathology. On the contrary, only a very few studies investigated white matter (WM) involvement using DT MRI in these patients [22, 23]. One study, which assessed patients with the E200K familial variant of CJD [22], found a significant decrease of fractional anisotropy (FA) of the corticospinal tract, internal capsule, external capsule, fornix, and posterior thalamic radiation, which was correlated with disease duration. FA alterations of fCJD were mainly due to an increase of radial diffusivity [22], suggesting axonal damage presumably secondary to PrPSc propagation along WM pathways. A second DTI study in sCJD patients did not find WM abnormalities in the corpus callosum and posterior limb of the internal capsule, but showed a significant mean diffusivity (MD) decrease in the caudate and pulvinar regions relative to healthy controls [23].
Alzheimer’s Disease and Mild Cognitive Impairment
The earliest pathologic brain abnormalities associated with AD develop years, if not decades, before the onset of the first memory symptoms [24, 25]. Such alterations include misfolded proteins aggregating into extracellular amyloid β plaques and intracellular neurofibrillary tangles, followed by inflammatory damage, oxidation, excitotoxicity, and cell death in the central nervous system . The prospect of experimental treatment with the potential to slow or prevent AD progression has prompted an increased interest in the identification of individuals with AD early in the course of the disease, even at the mild cognitive impairment (MCI) stage [6].
DTI in AD and MCI Patients
The loss of cortical neurons in AD is invariably accompanied by axonal degeneration along WM pathways . DTI studies of AD have found consistently increased MD and decreased FA compared with controls in several brain regions (Fig. 17.2), most notably in temporal and frontal lobes, posterior cingulum, corpus callosum, superior longitudinal fasciculus (SLF), and uncinate fasciculus [26, 27]. A posterior-to-anterior gradient in the severity of WM abnormalities has been observed, with posterior regions being affected more severely [26]. WM changes in AD generally follow the anatomical pattern of cortical atrophy [26, 28], supporting the theory that Wallerian degeneration may account for WM involvement in this disease.
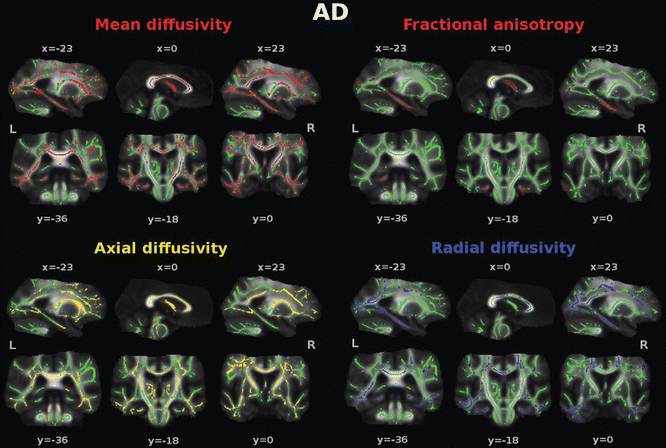
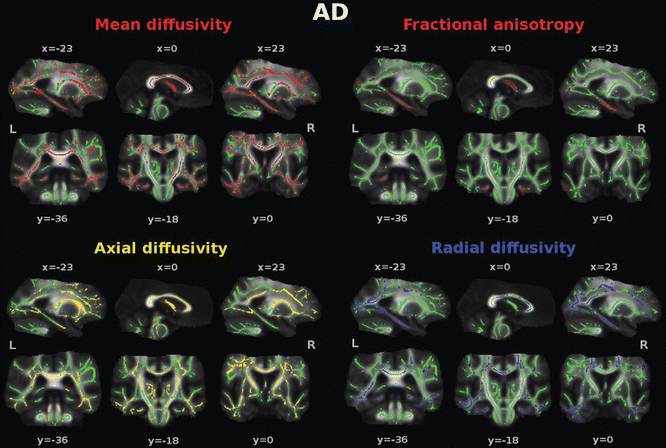
Fig. 17.2
Diffusion tensor imaging (DTI) abnormalities in patients with Alzheimer’s disease (AD) compared with healthy controls. Tract-based spatial statistics color maps showing voxel-wise differences between patients and healthy controls are overlaid on a mean fractional anisotropy (FA) skeleton. Voxels of increased mean diffusivity and decreased FA are shown in red; voxels of increased axial and radial diffusivity are shown in yellow and blue, respectively. The results for mean, axial, and radial diffusivity are shown at p < 0.05 corrected for multiple comparisons (family-wise error). Results from FA are shown at an uncorrected statistical threshold (p < 0.05) [Reprinted from Agosta F, Pievani M, et al. “White matter damage in Alzheimer disease and its relationship to gray matter atrophy.” Radiology 2011;258(3): 853–63. With permission from The Radiological Society of North America.]
The clinical relevance of DTI alterations in AD patients is reflected by the association between decreased global cognition, as measured using the Mini-Mental State Examination (MMSE) , and reduced FA [29–41] or increased MD [30, 32, 33] values in at least one brain region. Significant correlations between altered DTI metrics of temporo-parietal WM structures and neuropsychological measures of memory performance have also been reported by several studies [42–46]. In addition, a number of studies also showed that decreased FA in the frontal lobe correlates with poor performance at neuropsychological assessment of executive functions of AD patients [35, 47–49], as well as with the severity of neuropsychiatric symptoms [50, 51].
DTI studies have attempted to elucidate the earliest point at which diffusivity abnormalities can be detected by focusing on patients with amnestic MCI [28, 33, 35, 43, 46, 52–63]. Two recent meta-analyses showed that differences between amnestic MCI and controls parallel those between AD and controls, but fewer regions reached statistical significance [26, 27], possibly because MCI consists of an heterogeneous group of individuals and there are no universally recognized criteria to define this condition. Corpus callosum, cingulum, parahippocampal and frontal tracts were found to be affected in the prodromal stages of AD [28, 43, 54, 55, 59, 60, 63]. Interestingly, in patients with amnestic MCI, damage to the majority of WM tracts was not correlated with cortical atrophy [28], thus suggesting that, in the early phases of the disease, WM abnormalities may reflect a primary WM tract damage over and above cortical pathology . Similar findings have been reported in cognitively normal individuals who were later diagnosed with amnestic MCI at 2-year follow-up [64]. WM alterations at baseline in these subjects were predictive of subsequent cognitive decline, in the absence of significant correlation with cortical atrophy [64]. In addition, a number of studies [65–72] showed a similar pattern of WM damage in cognitively normal individuals with high risk for the development of AD (i.e., Apolipoprotein E4 genotype and/or positive family history). Preclinical subjects with familial autosomal dominantly inherited AD also showed decreased WM integrity of the fornix and orbitofrontal lobe [73]. Taking together, these studies suggest that microstructural WM changes may serve as a potential imaging marker of early AD-related brain damage.
DTI tractography can improve the sensitivity and specificity of diffusion measurements by localizing WM changes in specific neuronal pathways of MCI/AD patients . Diffusivity abnormalities have been shown in the splenium of the corpus callosum, posterior cingulum and uncinate fasciculi [74–78] of AD patients. Only a few tractography studies have been carried out in amnestic MCI patients [79–82], and diffusion abnormalities of the posterior cingulum were found in these patients relatively to controls, but not to early AD patients [79]. In amnestic MCI, WM damage was found not only in the limbic pathways (i.e., fornix [82] and cingulum [81]), but also in the major cortico-cortical WM tracts subserving association cortices (i.e., the uncinate fasciculus, the inferior fronto-occipital fasciculus, the inferior longitudinal fasciculus, the superior longitudinal fasciculus and the corpus callosum) [80]. Recently, a novel method to analyze DTI data, called anatomical connectivity mapping (ACM) , has been proposed to assess abnormalities of structural brain connectivity. ACM can be easily obtained by initiating diffusion tractography streamlines from all parenchymal voxels, and then counting the number of streamlines passing through each voxel of the brain. An exploratory study employing such an approach in patients with AD and amnestic MCI revealed that ACM provides information that is complementary to that offered by FA with increased sensitivity [83]. Additionally, an unexpected increase of ACM has been found in the putamen of AD (the only group under treatment with cholinesterase inhibitors) but not of MCI patients [83]. An intriguing explanation is that such an increase of ACM in AD patients might reflect brain plasticity driven by cholinesterase inhibitors .
Diagnostic Accuracy of DTI in AD and MCI Patients
For DTI to be useful in the clinical setting, one must be able to make inferences at the level of the individual rather than the group. Adding DTI measurements of the posterior cingulum to hippocampal volume significantly improves the accuracy in separating AD and amnestic MCI subjects from healthy controls (from 63 to 74 % in amnestic MCI, and from 78 to 91 % in AD) [55]. Diffusivity abnormalities of the left posterior cingulum were able to distinguish subjects with amnestic MCI from those with non-amnestic MCI with an accuracy of 85 % [56]. In addition, hippocampal diffusivity measurements were found to be more sensitive than hippocampal volume in predicting conversion to AD in patients with amnestic MCI [33, 52, 53]. The severity of microstructural damage beyond the medial temporal lobe was also associated with an increased short-term risk to develop AD in amnestic MCI patients [58]. A reduction of FA of the fornix has been recently proposed as an imaging sign that may be helpful in order to differentiate AD patients from normal controls with an accuracy of 75 %, as well as to predict conversion from normal cognitive status to amnestic MCI and from amnestic MCI to AD (with an accuracy of 96 % and 92 %, respectively) [41]. An individual classification of MCI cases using support vector machine analysis of DTI data allowed for an individual classification with an accuracy up to 91 % (healthy controls vs. MCI) and 98 % (stable vs. progressive MCI at 1 year) [57]. Furthermore, such an approach resulted in a highly accurate individual classification of stable vs. progressive MCI at 1 year, regardless of the MCI subtype, indicating that it might become a tool for early detection of MCI subjects evolving to overt dementia [57].
Longitudinal DTI Studies
Longitudinal studies of fiber tract injury in neurodegenerative diseases are still scanty. Amnestic MCI subjects experience a significant fractional anisotropy (FA) decline predominantly in the anterior corpus callosum after 13–16 months from a baseline scan [84]. More recently, longitudinal changes of WM microstructural alterations in AD patients with and without treatment with galantamine, a cholinesterase inhibitor, were evaluated over a 12-month follow up [85]. Galantamine slowed the FA decrease of the posterior body of the corpus callosum over a 6-month period compared to placebo, but this effect was not seen anymore after a 6-month open-label treatment of all AD patients [85]. A large-scale application of DTI in multicentre studies may help in the identification of markers that are likely to provide sensitive outcome measures in clinical trials of patients with AD and MCI.
Novel Strategies of Analysis
A correlation between the site of amyloid-β deposition in AD patients and the location of major brain hubs as defined by graph theoretical analysis of functional connectivity in healthy adults has been demonstrated [86]. These regions include the posterior cingulate cortex/precuneus, the inferior parietal lobule, and the medial frontal cortex, implying that the hubs are preferentially affected in the progression of AD. Using structural and DTI, abnormal topological properties were described also in the structural brain networks of patients with AD. In a study that used between-subject covariation in regional measures of cortical thickness to infer anatomical networks from a large structural MRI data set, global clustering and path length were increased in patients with AD relative to controls [87]. This study also found a decreased centrality of the classical hubs, such as the temporal and parietal heteromodal cortices, and an increased centrality of unimodal association cortex, such as the lingual gyrus and lateral occipitotemporal gyrus, as well as paralimbic regions [87]. The global clustering coefficient and path length of MCI structural networks were found to be intermediate between the AD group and normal elderly people [88]. In addition, compared with controls, AD and MCI patients retained their hub regions in the frontal lobe but showed a loss of such regions in the temporal lobe [88]. Similar to functional MRI data, this study also revealed increased short-range interregional correlations and disrupted long distance interregional correlations in MCI and AD [88]. An abnormal topological organization of large-scale WM networks was found in AD patients using DTI, with increased path length and decreased global efficiency compared with controls [89]. More importantly, WM connectivity patterns were associated with cognitive deficits [89], implying that a disturbed communication between different brain regions is likely to be important in the cognitive decline typical of this condition.
Frontotemporal Lobar Degeneration
Frontotemporal lobar degeneration (FTLD) represents the second most common early-onset neurodegenerative dementia after AD. FTLD is a clinically and pathologically heterogeneous spectrum of disorders, which encompasses distinct clinical syndromes: the behavioral variant of frontotemporal dementia (bvFTD) [10], and the language variant [9]. BvFTD presents with marked changes in personality and behavior [10], and, pathologically, is associated with all the three major FTLD pathologies, characterized by abnormal cellular inclusions containing either tau, TAR DNA-binding protein 43 (TDP-43), or fused-in-sarcoma protein [90]. In the language variant, known as primary progressive aphasia (PPA) , a prominent, isolated language deficit is the dominant feature during the initial phase of the disease [91]. Distinct profiles of language impairment define the three clinical phenotypes of PPA [9]: the nonfluent/agrammatic (for convenience hereafter called nonfluent), characterized by agrammatism in language production and effortful speech with motor speech deficits; the semantic, characterized by progressive loss of knowledge about words and objects in the context of relatively preserved fluency of speech; and the logopenic, characterized by impaired naming and repetition in the context of spared syntactic and motor speech abilities. Clinicopathological series suggest that the majority of nonfluent patients exhibit FTLD-tau or, less frequently, FTLD-TDP pathology, while most semantic cases have a TDP-43 proteinopathy [90]. AD is the most likely underlying pathology of the logopenic variant [92].
DTI vs. Pathology and Genetic Background in FTLD
In a single, pathology-proven bvFTD case, DTI detected decreased FA values in WM frontal regions, where histopathology revealed a typical frontal lobe degeneration of non-AD type [93]. Neuropathological [94, 95] and structural MRI [96] observations suggested that FTLD-tau may have a more severe involvement of WM than FTLD-TDP cases. In view of this, DTI metrics were recently evaluated in autopsy- or genetic-proven FTLD patients and showed a 96 % sensitivity and 100 % specificity in distinguishing between the two pathological variants [97]. This could prove to be clinically relevant, as potential disease-modifying treatments emerge that target tau or TDP-43.
Microtubule-associated protein tau (MAPT), progranulin (GRN), and C9orf72 mutations are the major genetic causes of autosomal dominant FTLD [98, 99]. Presymptomatic individuals with these mutations represent the ideal population to assess the initial alterations of FTLD. A DTI study performed in a small sample of subjects with GRN mutation revealed decreased FA of the left uncinate and left inferior occipitofrontal fasciculi, with respect to non-carrier controls [100]. More recently, a larger sample of MAPT or GRN mutation carriers was found to have distributed pattern of reduced FA in frontotemporal WM tracts, in comparison with non-carriers [101].
DTI in bvFTD Patients
Using a regions of interest (ROI)-based approach, a study of bvFTD patients found DTI abnormalities of WM tracts passing through the frontal—anterior cingulum, genu of the corpus callosum, anterior SLF—and temporal lobes—uncinate fasciculus and inferior longitudinal fasciculus (ILF) [102]. Voxel-based DTI studies in bvFTD patients found FA reduction in frontoparietal regions, which are likely to correspond to the SLF [103, 104], and in frontal and temporal WM regions, including the anterior corpus callosum, anterior cingulum, and uncinate, bilaterally (Fig. 17.3) [75, 104]. Such findings were confirmed by a DTI tractography study, which disclosed a significant FA decrease in all major association WM tracts (ILF, uncinate, and SLF) and genu of the corpus callosum, as well as a sparing of corticospinal tracts and splenium of the corpus callosum [105]. The majority of studies also detected diffusivity abnormalities in posterior WM regions, such as the posterior SLF and the posterior cingulum [75, 102, 104]. Although bvFTD is typically associated with frontal and anterior temporal atrophy [106], patterns of atrophy are known to be heterogeneous in this condition [107]. In addition, even in the classic frontotemporal cases, the lateral and medial parietal lobes usually become affected later in the disease course [108]. It is therefore tempting to speculate that DTI metrics may be viewed as early markers of WM injury in bvFTD patients, which may result at a later stage in detectable volumetric abnormalities.
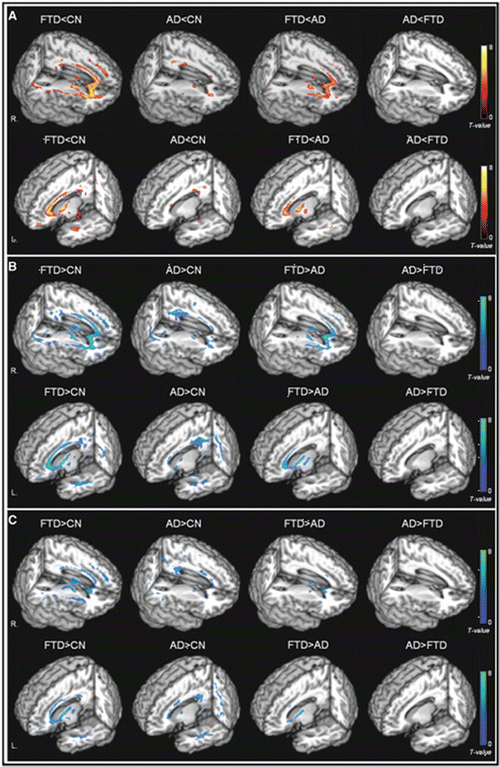
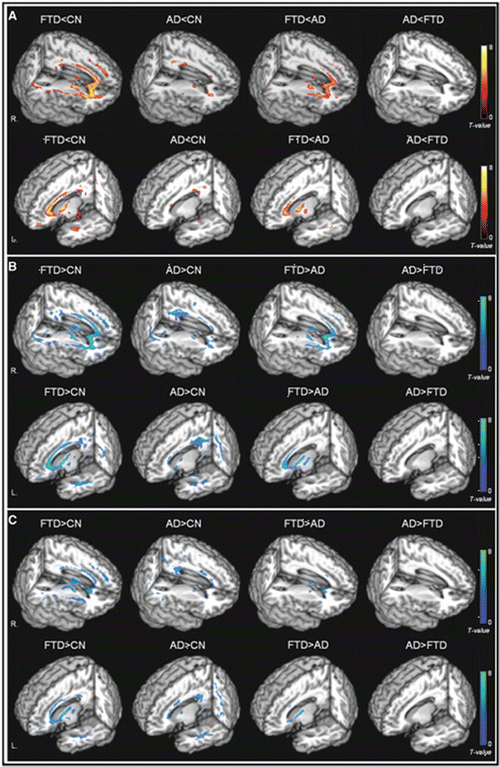
Fig. 17.3
Rendered displays of diffusion tensor imaging (DTI) abnormalities in patients with the behavioral variant of frontotemporal dementia (bvFTD) or Alzheimer’s disease (AD) compared with cognitively normal subjects, as well as direct comparisons between bvFTD and AD: (a) reduced fractional anisotropy (warm colors); (b) increased radial diffusivity (cool colors); (c) increased axial diffusivity (cool colors) [Reprinted from Zhang, Y., N. Schuff, et al. “White matter damage in frontotemporal dementia and Alzheimer’s disease measured by diffusion MRI.” Brain 2009;132(Pt 9): 2579–92. With permission from Oxford University Press.]
DTI in PPA Patients
To date, only a few studies to date have investigated the patterns of WM abnormalities in small samples of PPA patients using DTI. Most of these studies were performed in patients with the two major variants (i.e., nonfluent and semantic) .
Using ROI-based or tractography DTI to investigate the WM language tracts of these patients, it has been showed that the nonfluent variant is characterized by an involvement of all the left SLF components [102, 109–111] (Fig. 17.4). In contrast, the ventral tracts connecting the temporal lobe with the occipital and the orbitofrontal cortices (i.e., the ILF and uncinate fasciculi) were relatively spared [102, 109, 111]. Studies using a voxel-wise approach showed that nonfluent patients have not only a damage to the fronto-parieto-temporal connections but also an involvement of the corpus callosum, cingulum bundle, external capsule, several regions of the prefrontal and orbitofrontal and parietotemporal WM, mainly in the left hemisphere [104, 112, 113]. A recent tract-based spatial statistics (TBSS) study found a damage to portions of the ILF in nonfluent patients [112].
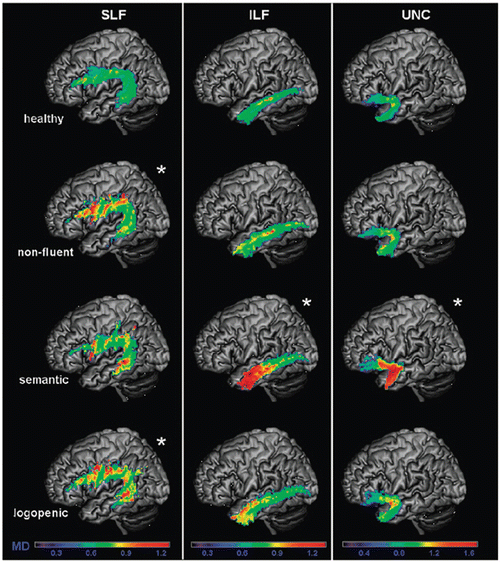
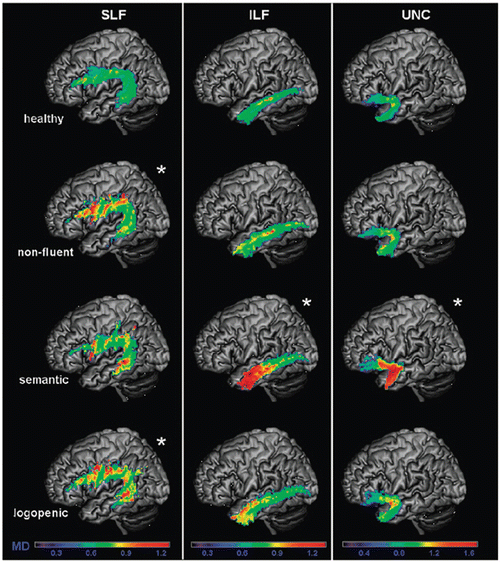
Fig. 17.4
Mean diffusivity (MD) values of healthy controls, nonfluent, semantic, and logopenic variants of primary progressive aphasia are shown on the probability maps for left superior longitudinal fasciculus (SLF), inferior longitudinal fasciculus (ILF), uncinate fasciculus (UNC), overlaid on a standard Montreal Neurological Institute brain. Only voxels that are in common in at least 20 % of the subjects in each group were included in the probability maps. Asterisks denote significant difference relative to normal controls at p < 0.05. The chromatic scale represents average MD values ranging from lower (violet-blue) to higher values (yellow–red). MD is measured in mm2/s × 10−3 [Reprinted from Galantucci, S., M. C. Tartaglia, et al. White matter damage in primary progressive aphasias: a diffusion tensor tractography study. Brain 2011;134(Pt 10): 3011–29. With permission from Oxford University Press.]
In the semantic variant, abnormalities of WM tract diffusivity were identified in the major inferior and superior temporal connections of the left hemisphere, thus mirroring the severe atrophy affecting the same regions: the ILF, inferior-fronto-occipital fasciculus, and the uncinate fasciculus within the ventral stream, and the arcuate and the temporo-parietal component of the SLF within the dorsal stream [102, 104, 109, 112, 114, 115] (Fig. 17.4). Although tractography studies have suggested that the fronto-parietal connections are relatively spared in the semantic variant [109, 114], a finding which couples with the absence of syntactic deficits in these patients, a few TBSS reports have shown an involvement of the left prefrontal and parieto-frontal WM contributing to the SLF and corona radiata [104, 112].
DTI measures of the anterior corpus callosum and left SLF differentiated bvFTD from nonfluent cases, while the best predictors of semantic PPA compared with both bvFTD and nonfluent cases were diffusivity abnormalities of the left uncinate and inferior longitudinal fasciculus [104].
Two studies so far applied DTI to patients with the logopenic variant of PPA [104, 109], showing the most consistent abnormalities in the left SLF temporoparietal component; abnormalities were also detected in the left arcuate fasciculus, other components of the left SLF and right temporoparietal SLF (Fig. 17.4). These findings suggest that the logopenic variant is associated with damage to the WM tracts connecting regions important for sentence repetition and phonological short-term memory [116].
Differential Diagnosis: FTLD vs. AD
DTI is increasingly being used to improve the diagnostic differentiation between FTLD and AD. When compared with AD, bvFTD is associated with greater reductions of FA in frontal regions (Fig. 17.3) [75], which suggests that WM injury is more prominent in bvFTD than in AD. One recent study combined cortical thickness measurement and DTI to compare FTLD (both bvFTD and PPA) and AD patients, with autopsy- or cerebrospinal fluid-confirmed disease [117]. The direct comparison of the two groups showed a significantly greater atrophy in inferior frontal, medial frontal and prefrontal cortical regions, and a reduced FA of the genu of the corpus callosum, left inferior fronto-occipital fasciculus, cingulum, uncinate and bilateral corona radiata in FTLD cases relative to AD [117]. Conversely, AD patients showed no areas of significant reduction in cortical thickness or WM integrity relative to FTLD patients [117]. Another recent study compared DT MRI alterations and patterns of cortical atrophy between FTLD and AD patients, identifying a significant area of reduced FA in the anterior corpus callosum of FTLD patients, as well as a significantly more severe atrophy of the precuneus and posterior cingulate cortex in AD patients [118]. A combination of WM and cortical data provided a highly accurate classification of these two conditions, with 87 % sensitivity and 83 % specificity [118].
Dementia with Lewy Bodies
Dementia with Lewy bodies (DLB) is the second most common form of neurodegenerative dementia in elderly subjects [8]. Memory impairment is generally less severe in patients with DLB than in those with AD, whereas deficits on tests of attention, executive function, and visuospatial ability can be prominent. In pathological studies, a distributed pattern of Lewy bodies has been observed in the neocortex, limbic structures, subcortical nuclei and brainstem of these patients [119, 120].
DTI in DLB Patients
Only a few studies investigated diffusivity alterations in DLB patients [76, 121–127]. The comparison between DLB patients and controls using DTI resulted in controversial findings, ranging from isolated damage to the ILF [123, 124], uncinate fasciculus [126] or parietal lobe [122] to a distributed pattern of FA reduction [121, 125]. The most consistent finding among studies was a decreased FA in the ILF [121, 123, 124], as well as reduced FA or increased MD in tracts of the parieto-occipital lobes [121, 122, 124, 125, 127], particularly the posterior cingulum and precuneus (Fig. 17.5a). Damage to these regions, which are important for visual information processing, may reflect the prevalent impairment of visuospatial abilities, as well as the presence of visual hallucinations in these patients. One study [124] reported significantly higher diffusivity of the ILF from patients with DLB experiencing visual hallucinations, in comparison with DLB patients who did not.
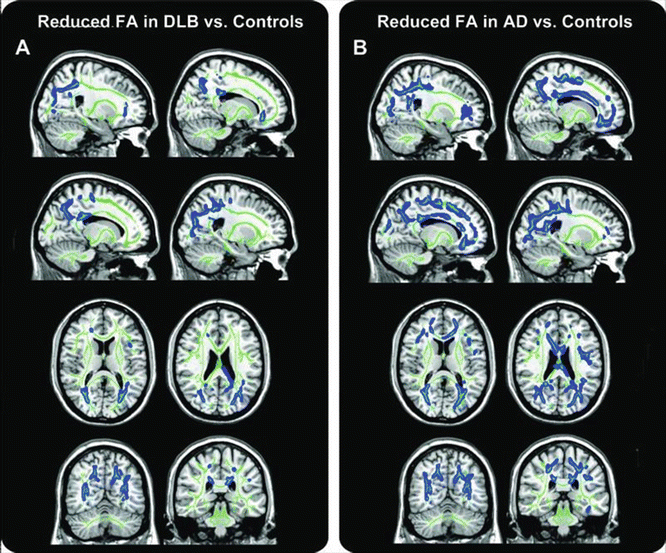
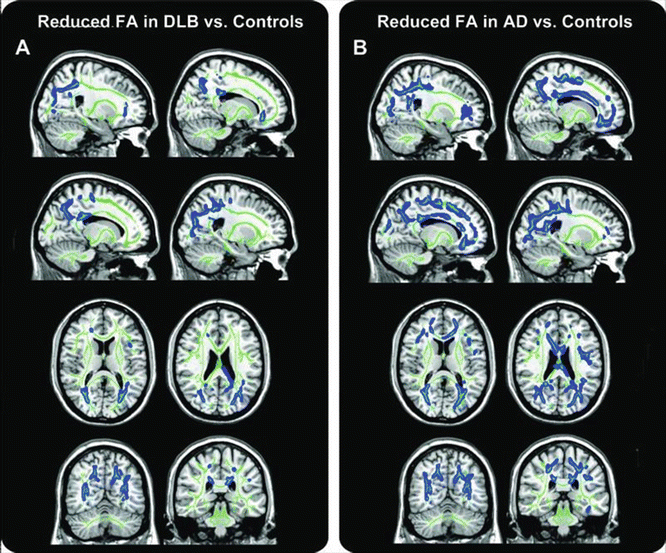
Fig. 17.5
Fractional anisotropy (FA) reduction in patients with dementia with Lewy bodies (DLB) and Alzheimer’s disease (AD). Tract-based spatial statistics color maps showing voxel-wise differences in FA (blue) between these groups are overlaid on a mean FA skeleton (green). (a) DLB vs. controls: abnormalities were identified mainly in the parieto-occipital areas (precuneus and cingulate gyrus). Reduced FA in the temporal lobes was detected in a region of the posterior thalamic radiation that included the optic radiation (p < 0.05, corrected for multiple comparisons). (b) AD vs. controls: the pattern of abnormalities was more distributed than in DLB and included clusters in the temporal, parieto-occipital, and frontal lobes (p < 0.05, corrected) [Reprinted from Watson, R., A. M. Blamire, et al. “Characterizing dementia with Lewy bodies by means of diffusion tensor imaging.” Neurology 2012;79(9): 906–14. With permission from Wolters Kluwer Health.]
Differential Diagnosis: DLB vs. AD
Diffusivity abnormalities in the cortex of patients with AD are more distributed and severe than in those with DLB [122, 124]. Patients with AD experience an increased diffusivity and a reduced cortical volume in the medial temporal lobe, posterior cingulate cortex, precuneus, and temporoparietal association cortex compared to both healthy controls and patients with DLB [124]. The addition of diffusivity values of the hippocampus and parahippocampal gyrus to those of cortical volumes improves further the ability to distinguish AD patients from those with DLB [124].
A reduction of FA in the uncinate fasciculus has been shown both in AD and DLB patients [76, 126], with additional more posterior damage in patients with DLB [76]. A recent study [127] showed an involvement of the parieto-occipital and temporal WM tracts both in DLB and AD, but a much greater ratio of posterior-to-anterior suprathreshold voxels was found in DLB—6.5—when compared to AD—1.1 (Fig. 17.5) [127]. Similarly, the few other studies investigating WM damage in DLB showed a more posterior pattern of abnormalities in comparison to AD [122, 123], with a relative sparing of the structures of the temporal and frontal lobes , reflecting the structural preservation of these areas in DLB [128].
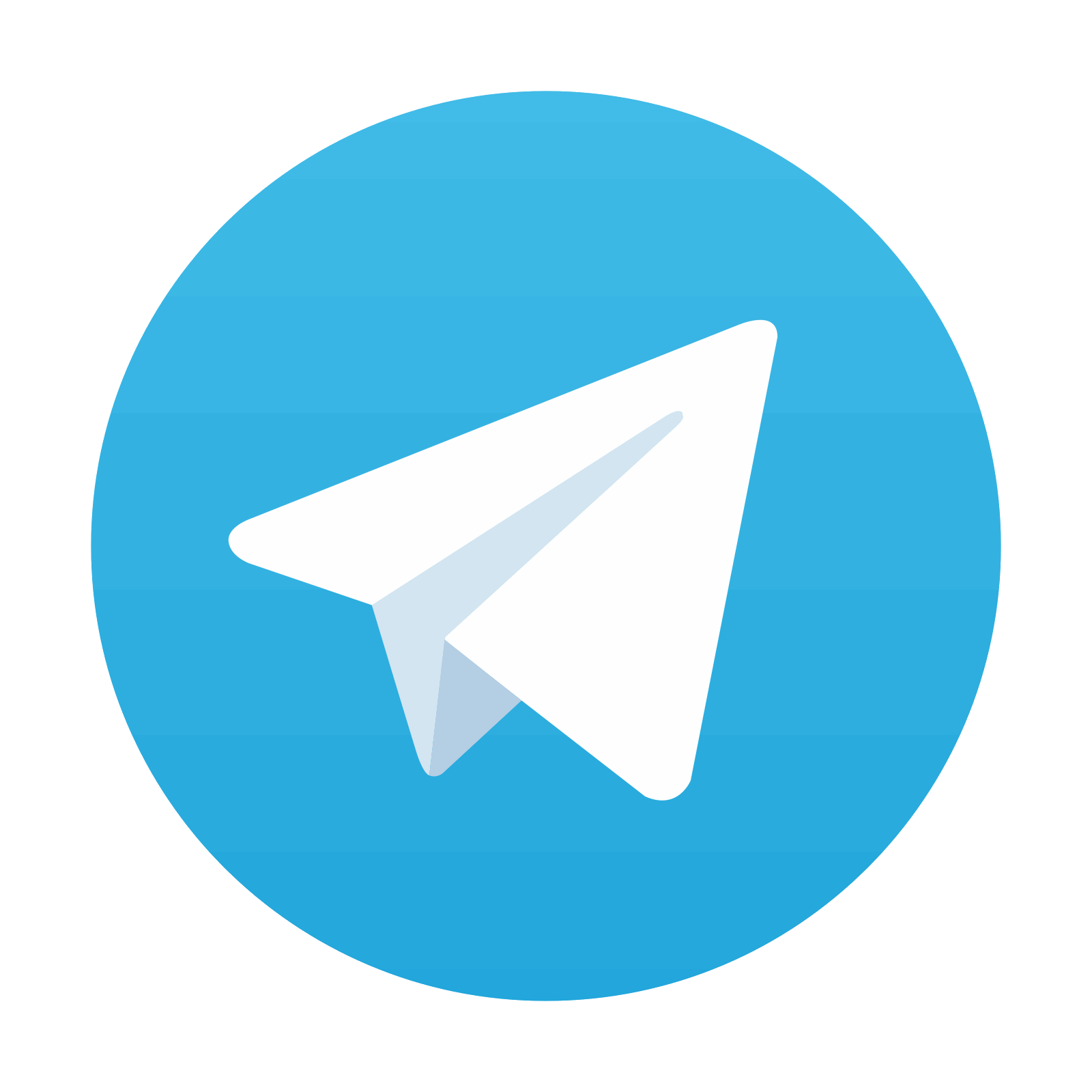
Stay updated, free articles. Join our Telegram channel

Full access? Get Clinical Tree
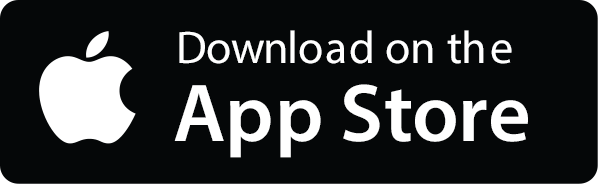
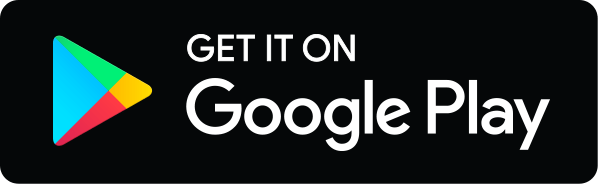