Fig. 14.1
Corticospinal tract (CST) mapping with fMRI guidance. Seed ROIs in the cerebral peduncles showed multiple fiber tracts in addition to CST, left more than right. Hand (magenta) and face (orange) activation maps (a and b, enhanced T1 images) were used to select an inclusion ROI around sensorimotor cortex. The patient could not move their left hand and no hand activation was elicited, but face motor activation was identified in the right hemisphere (arrows). A generous ROI similar to that in (b) was chosen for both sides, showing left greater than right CST tracking (c, 3D cut plane view). Lower extremity function was still partially intact at this time, and the ROI included this cortical region as well (fMRI not shown)
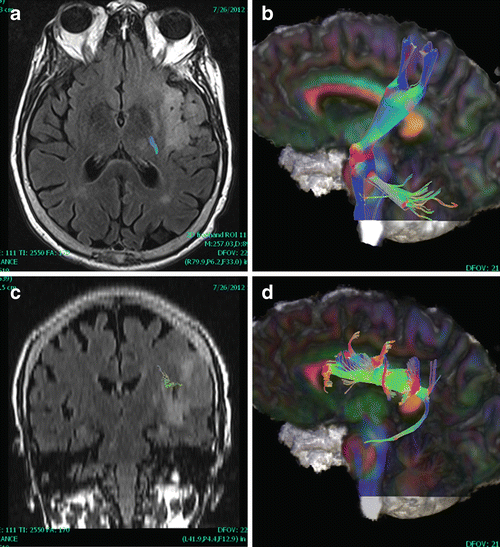
Fig. 14.2
Corticospinal tract (CST) and superior longitudinal fasciculus (SLF). For a patient with gliomatosis, fMRI failed because the tumor interfered with language such that the patient could not follow commands, even for motor tasks; however, fiber tracking was adequate. Left CST (a, b) and SLF (c, d) are shown on FLAIR (a, c) and 3D cut plane views (b, d)
Motor
Preservation of motor function is critical and mapping of the corticospinal (pyramidal) tract (CST) and motor cortex is most commonly requested. There are multiple reports of its utility in preoperative planning [13, 16–20]. If pathology has not sufficiently altered the tract, basic single tensor DTI FT for the CST in general works well for trunk and in some cases hand and lower extremity; however, it is less successful for cortical regions like the face because intervening tracts like the SLF interfere with continuous tracking in critical voxels due to crossing fibers in these locations. Some detail in somatotopic mapping is possible [6, 13]. Yamada et al. [21] used cortical ROIs for lower extremity, trunk, hand, face, and tongue and found robust tracking only for the trunk with a single tensor approach while a HARDI-based multitensor approach was more successful in tracking for other cortical regions including face and tongue. Techniques such as HARDI will be required if detailed somatotopic mapping is desired [3, 21].
Multiple studies have addressed validation of cortical and subcortical motor mapping with DTI FT [3, 15, 22–24]. Berman et al. [22] used seed points identified during intraoperative stimulation mapping (ISM) to retrospectively map ipsilateral CST in 11 glioma patients using the preoperative imaging data after the surgery was complete. Though not used for surgery, they showed appropriate tracking to the central cerebral peduncle based on the seed points verified intraoperatively; however, there were some failures in tracking depending on the extent of pathologic alterations in or near the CST. Kamada et al. reported studies confirming CST mapping with subcortical [23] and cortical mapping [15]. The latter included 30 patients with lesions in proximity to the motor system, with central sulcus and sensorimotor cortex identified using DTI FT and verified with intraoperative cortical somatosensory evoked potential (SEP) measurements. Functional MRI or MEG identification of primary motor cortex failed in nine patients while DTI FT was successful in all.
Accuracy of CST localization with DTI FT compared with subcortical stimulation appears to be within 10 mm [3, 24, 25]. Berman et al. [3] reported a mean distance of 8.7 mm (±3.1 mm standard deviation) for 16 sites in 9 patients. Mikuni et al. [24] found consistent motor evoked potentials (MEP) at 7 mm or less and absent MEP over 13 mm when stimulating near the mapped CST fibers at the inferior aspect of the mass. Between 8 and 12 mm, stimulation near the mapped CST at the level of the corona radiata consistently elicited MEPs. Another study of 40 patients with masses within 2 cm of CST found MEPs elicited at less than 1 cm from mapped CST in 18 of 21, negative MEPs in 5 at stimulation points greater than 2 cm, and negative MEPs in 12 of 15 at 1–2 cm from mapped CST [26]. Motor function was ultimately preserved in all, improved in five cases with weakness prior to surgery, and two of the cases with negative MEP at 1–2 cm distance developed SMA syndrome , which subsequently resolved.
Other studies have also addressed impact on surgical plan and/or outcomes [16, 25, 27–31], in general with preserved or improved clinical function postoperatively. Bello et al. [27] reported high correlation of DTI FT for CST (and multiple language tracts) with intraoperative subcortical mapping (ISM). With low grade gliomas, fibers tracking through the neoplasm were often found. A combination of DTI FT and ISM decreased surgery time, patient fatigue and intraoperative seizure rate.
With increased extent of resection, postoperative deficits have been reported to increase transiently but with improvement over time [25, 32, 33]. The concept of a safety margin of about 5–10 mm has been introduced, but with careful intraoperative mapping this boundary may be minimized [32]. In a prospective randomized study by Wu et al. [30], 238 patients with suspected primary supratentorial glioma (214 ultimately proven gliomas) not previously treated and near the pyramidal tract with at least 1/5 motor strength were randomized to surgery with conventional neuronavigation versus neuronavigation with integrated DTI data. Only FA maps were used for intraoperative mapping, although in the later stages of the study tractography was added for preoperative planning and postoperative assessment (23 of the cases). Those in the neuronavigation plus DTI group were more likely to have complete resection of high grade tumors (74.4 % vs. 33.3 %), although low grade gliomas had similar extent of resection. Decline in motor function postoperatively was less likely overall (15.3 % versus 32.8 %) and 6 month Karnofsky scores were better (86 ± 20 vs. 74 ± 28). They also reported improved survival for high grade gliomas (21.2 months vs. 14.0 months).
Vision and Language
The optic radiations (OR) and in particular Meyer’s loop pose difficulties for DTI FT. Problems include not only the anatomy with tight turns, but location along medial temporal lobe where susceptibility-related artifacts can affect image quality with DTI sequences using EPI [34, 35]. Crossing fibers can create problems here as elsewhere. There are early reports using DTI FT to map visual pathways with integrity of OR on DTI FT linked to deficits pre- and/or postoperatively [29, 35–38], and intraoperative correlation also described in at least one case [36]; however, results remain preliminary and further validation is required and caution warranted in this setting [35].
Validation of language tractography is also ongoing and though reports are less extensive than for CST mapping, experience is growing. Co-localization with intraoperative mapping seems to be similar to CST; that is, distance between DTI FT prediction and subcortical mapping is in the range of 5–10 mm. For example, Kamada et al. [39] studied 22 lesions near the superior longitudinal/arcuate fasciculus (SLF/AF) in the dominant hemisphere, using fMRI or MEG to choose seed and target ROIs. For two of these cases, functional mapping was integrated into neuronavigation and validated by cortical and subcortical mapping during awake surgery with stimulation near AF leading to paranomia without speech arrest at a distance of 6 mm. Leclercq et al. [40] studied multiple language tracts in ten patients with low grade gliomas or dysplasia, reporting positive subcortical stimulation responses 6 mm or less from DTI FT prediction in 81 %, but they also reported four false negative results (positive stimulations away from any mapped fiber tract). Of course, understanding of language functional cortical regions and connections remains incomplete, and important fiber tracts not anticipated and thus not mapped are considerations as well [41].
False positives for DTI FT based on subcortical mapping have also been described [27], with DTI FT mapping of SLF larger than identified on subcortical mapping in some cases such that ISM did not evoke a response in some tracked fibers, and there were also false negatives in this study which could be addressed in part by adjusting ROI placement. For low grade gliomas, tracts could be identified within the neoplasm and in some cases ISM evoked a response within the mass even when tracts appeared interrupted. IFOF and UF were also evaluated in this study. The majority of patients where motor or language tracts were identified with ISM had decline immediately after surgery, in general improving after 1 week (about 90 % normal at 1 month).
Zhao et al. [42] used preoperative as well as intraoperative DTI FT for the SLF/AF in 20 patients, 9 of which had no preoperative deficit and 11 with variable conduction aphasia. They monitored intraoperative shifts of the tract from −5 to 2 mm. After surgery, follow up within 1 month revealed one previously normal patient with new conduction aphasia while those with language deficit had improved. Many of the studies discussed here have integrated DTI FT data with neuronavigation systems , but not all scenarios necessarily require it. Review of preoperative data may be sufficient for confirming or altering an operative approach. Powell et al. [43] used a probabilistic fiber tracking method for hemisphere dominance prior to anterior temporal lobe resection, finding a significant correlation between frontotemporal tract lateralization to dominant hemisphere resections and postoperative naming deficits. Romano et al. [29] performed tractography for CST, OR, and AF in 25 patients, and for 35 of 75 tracts analyzed which were in proximity to a given neoplasm reported a change in surgical plan in 16 % and a change in extent of resection in 68 %.
Reduction in Radiation Damage to Eloquent WM Pathways
The concepts for sparing eloquent structures in surgery extend also to radiosurgery and to an extent to other therapeutic radiation strategies. Koga et al. [44] for example integrated tractography for CST, OR, and AF with navigation for AVM Gamma Knife radiosurgery in 71 of 155 treatments for 144 patients, optimizing dose such that the maximum dose to CST, OR, and AF was 20 Gy, 8 Gy, and 8 Gy respectively. Of the 71/155 treatments planned with this approach, 60 % had less than 5 mm separation from these WM tracts. Maruyama [45] integrated tractography for OR with navigation in ten patients receiving Gamma Knife near OR, reporting 8 Gy or more single dose to be associated with neurological changes such as visual field deficit and new (or improved) migraine symptoms. Another study included 24 patients (9 retrospective and 15 prospective) [46], reporting maximum dose to CST of 23 Gy with 5 % complication risk and 20 Gy and 25 Gy volumes for 5 % complication risk were 58 mm3 and 21 mm3, respectively.
There is also an interest in using DTI metrics for microstructural assessment not only to estimate extent of infiltration [47–50] but also to improve accuracy in diagnosis, assess grade or prognosis, evaluate treatment response, and potentially predict recurrence patterns. Jena et al. [48] described the use of a DTI-based “high risk volume” or IHVDTI which allowed a 35 % reduction in planning target volume and a dose escalation such that complications did not exceed conventional plan.
Targeted DBS Electrode Placement
Conventional imaging is often inadequate for visualization of important functional anatomy; for example, the subthalamic nucleus (STN) is difficult to demonstrate with high image contrast and targeting is not trivial [51]. DTI data can be used to improve localization of functional anatomy relevant to deep brain stimulation (DBS) placement. Simple integration of color FA maps has been utilized to augment identification of STN and globus pallidus interna (GPi) targets [52].
Other studies have shown proof of concept for use of DTI FT in this setting. Pouratian et al. [53] retrospectively studied cases where DBS had been placed using traditional methods for tremor control. The procedure was efficacious in 11/12 electrodes placed (six patients), and postoperative CT or MR fused with preoperative probabilistic tractography for thalamic voxel connectivity to primary, premotor, and prefrontal cortex showed thalamic sites with connectivity to premotor and supplemental motor regions were most efficacious in controlling tremor. A postoperative study of white matter tracts in proximity to leads placed in GPi, STN, and ventral intermediate nucleus (VIM) was useful in understanding side effects related to stimulator setting [54]. Tracts of interest in proximity to these targets included motor, premotor, supplemental motor, and frontal eye fields. Coenen et al. [55] also used a retrospective approach for DTI FT, showing unilateral refractory tremor control in a patient with Parkinson disease with DBS placement involving the dentate-rubral-thalamic tract (DRT) , subsequently prospectively targeting the DRT for DBS implantation bilaterally with excellent control of head tremor in a patient with myoclonus dystonia [56]. Other fields of interest where tractography may be potentially useful in this setting include neuropsychiatric problems [57, 58] including OCD and depression [59, 60], headache and chronic pain [61, 62].
Practical Considerations in Neurosurgery
Clinical Implementation
Image Acquisition and Postprocessing
There are multiple sources of potential image degradation related to patient and technique. Bulk and physiologic motion including cardiac and respiratory can degrade image quality. Additionally, the imaging sequence typically used (single shot spin echo EPI) has limitations which include eddy current distortion, mechanical vibrations related to large amplitude and rapidly switching gradients, Nyquist ghosting, chemical shift, magnetic field inhomogeneity, local susceptibility effects near bone and paranasal sinus interfaces , and limitations in spatial resolution and SNR [9]. Pathologic and postsurgical changes can also be problematic (e.g., hemorrhage and surgical hardware). There are strategies for solving or at least minimizing these problems including fat saturation techniques , cardiac gating, parallel imaging, and phased array coils. Improvements in image quality need to be balanced with the need for efficient implementation in this clinical setting such that data are adequate for presurgical planning but acquisition or processing times do not exceed a patient’s ability to cooperate or a clinician’s ability to obtain processed data integrated with other imaging data for surgical planning and intraoperative navigation.
An example of a standard acquisition at 3 T is provided in Table 14.1. While at least six diffusion encoding directions plus one baseline (b = 0 s/mm2 or low b-value) are needed to generate the diffusion tensor, a minimum of 30 directions are recommended for good quality DTI in this setting with at least 3–6 low-b acquisitions if possible [9, 63]. Acquisition time under 10 min is a reasonable target for integration into a full planning protocol and for patient compliance. Cardiac gating is not routinely used at our institution due to increased scan time, but this can improve image quality [64]. Small isotropic voxels with no gaps between imaging sections, on the order of 2 mm at 3 T, helps minimize partial volume effects and thus extent of crossing fibers in a given voxel [9].
Table 14.1
Sample DTI parameters at 3 T
Resolution (mm) | 1.72 × 1.72 × 3.0a |
Matrix | 128 |
FOV (cm) | 22 cm |
Encoding directions | 30 (plus baseline) |
NEX | 2–3 |
b-value (s/mm2) | 1000 |
TR/TE | 5000/86 ms |
Acquisition time | 8 min |
Parallel imaging acceleration | 2 |
Post-processing may include motion correction and eddy current distortion as well as registration with structural imaging data. Post-processed DTI data include scalar and directional data which can be used for fiber tracking, usually with a fiber assignment by continuous tracking (FACT) approach (most of the vendor supplied algorithms currently in use are based on this strategy). At least one “seed” ROI must be chosen, but typically at least one seed and target (inclusion) ROI are most commonly used (see below). Additional inclusion or exclusion ROIs are useful to minimize stray tracked fibers. Typical starting FA thresholds might be greater than 0.1–0.15 and turning angle less than 40–70°, and these can be adjusted for optimal display of desired tracts.
Preparation of multiple fiber tracts can be time-consuming and requires an understanding of neuroanatomy . A knowledgeable support staff is needed. Judicious selection of fiber tracts chosen and displayed for intraoperative navigation is important; for example, some navigation software can display only binary imported tract maps and though independent colors can be assigned, the amount of information can become confusing and counterproductive. Another approach is to generate all tracts based on a chosen threshold FA and curvature around the anatomic region of interest with subsequent selection of specific tracts of interest using ROIs [9]. Automated or semiautomated fiber tracking strategies are not routinely used for preoperative planning, but a technique allowing atlas-based segmentation would be of obvious utility for preoperative or radiation planning [65].
Both color FA maps and tractography should be used in interpretation [66, 67]. FA maps can be more reliable in proximity to a mass distorting local anatomy, while tractography is helpful for visualizing major tracts travelling in proximity to each other though less successful and potentially misleading when anatomy is substantially distorted and/or when anisotropy is altered such that fibers can no longer be tracked [68] (Fig. 14.3). Some institutions currently integrate only the color FA map for intraoperative navigation .
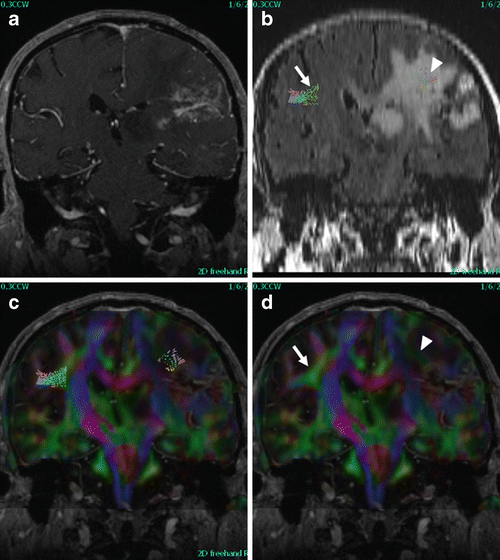
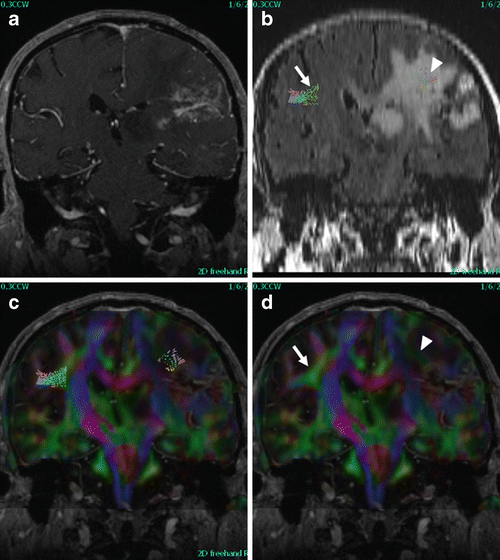
Fig. 14.3
Fiber tracking and color fractional anisotropy (FA) maps. Coronal enhanced T1 (a), reformatted FLAIR (b), and color FA maps with and without superior longitudinal fasciculus (SLF) tracts displayed (c, d). Fiber tracking on left was incomplete and did not extend anterior to enhancing mass, color FA maps show decreased intensity and loss of directional information (d, arrowhead) compared to normal hemisphere (d, arrow)
Incorporation into Navigation Software for Multimodal Data
Functional MRI , MEG and DTI with or without tractography are complementary techniques for assessing feasibility and extent of resection, predicting risk, and planning operative approach and they are also helpful intraoperatively for shortening cortical and subcortical intraoperative mapping times [68–70]. Many of the studies using DTI and/or tractography in preoperative planning discussed so far have also integrated results into neuronavigation systems prospectively, and while not yet universal across institutions this is becoming more widespread as validation improves. Tractography can show variable results with different software packages and with differences in user selection of tracking ROIs [66, 71], and of course pathology in and around a brain neoplasm can also lead to suboptimal or failed fiber tracking .
DTI and fMRI data can be integrated into commonly used navigation packages, in some instances the DTI/tractography software is integrated with the navigation software. In our current implementation, fMRI and DTI/tractography data are processed separately and functional activation maps and selected fiber tracts are uploaded to the navigation computer in DICOM format pre-registered with structural images, but there are many possible solutions which can be customized for each institution’s needs (Fig. 14.4).
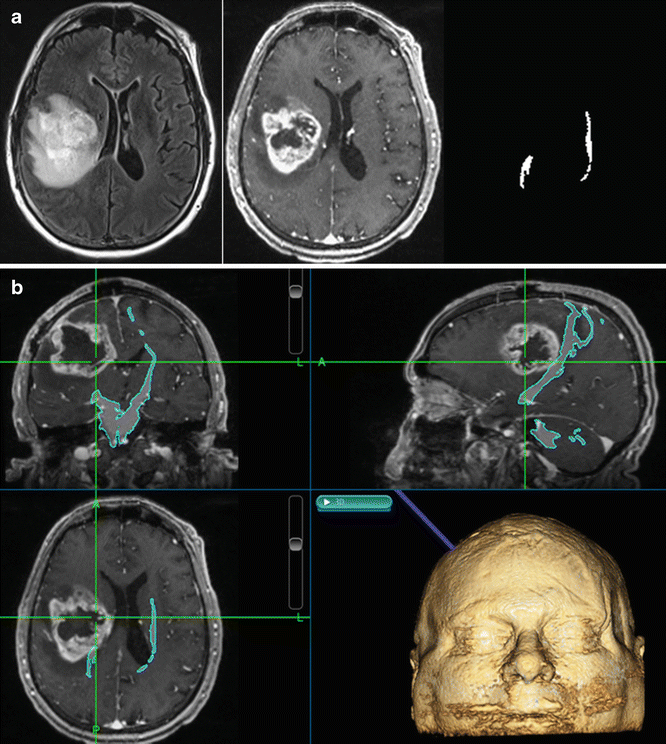
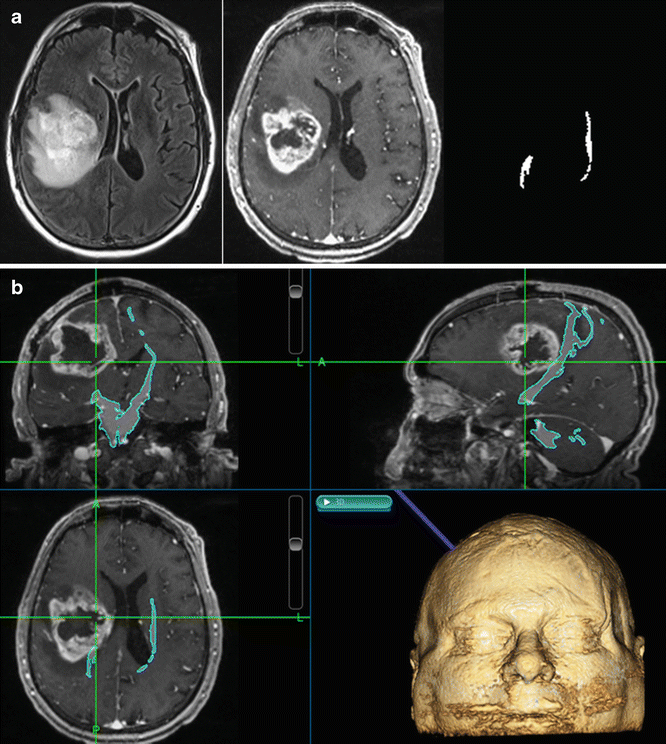
Fig. 14.4
Incorporation of fiber tracking into navigation software. Registered FLAIR, 3D T1 post-contrast and fiber bilateral corticospinal tract masks (a) were uploaded to the intraoperative navigation computer (b). Fiber tracts were less selective in this case to show cut-off anteriorly near the enhancing mass, a glioblastoma. The patient had facial paralysis on the left, but upper and lower extremity motor function was intact (although there was left upper extremity numbness)
Clinical Examples
Atlases of white matter tracts including proposed ROIs for tract generation are available [72–75]. These only provide guidelines since factors such as location and size of mass, degree of infiltration or destruction of fibers, presence of blood products or surgical hardware causing susceptibility, motion, or other factors affecting image quality may necessitate one or more approaches for a given patient. Alternatively, tractography may have to be abandoned if inadequate or potentially misleading.
Corticospinal (Pyramidal) Tract
A simple approach is to choose a seed ROI in the cerebral peduncle and display all tracts passing through this region. Other fiber tracts will be incorporated including thalamocortical fibers and other tracts in proximity in the peduncle, so increased specificity can be accomplished by using inclusion ROIs in the posterior limb of the internal capsule and/or subcortical ROIs in proximity to the precentral gyrus based on standard neuroanatomic landmarks. Alternatively, one may choose seed ROIs adjacent to the precentral gyrus and use inclusion ROIs corresponding to the posterior limb of the internal capsule (PLIC) and/or cerebral peduncle. Unwanted fibers can be further eliminated by choosing inclusion or exclusion ROIs above and/or below the tentorium.
Mapping of the CST is most frequently requested since preservation of motor function is paramount. Depending on extent of fiber disruption and where it occurs, motor impairment can be devastating and also more enduring than at the cortical level. The basic strategies outlined here are not sufficient for mapping the more lateral primary motor cortex for the face and sometimes for the hand and foot, but is usually robust for fiber tracking corresponding to the locus for trunk. Better tracking can ultimately be obtained with more complex techniques with longer acquisitions such as HARDI . The primary goal is to preserve contralateral motor function and so the focus here was on the CST, but of course other important tracts pass through the internal capsule in the anterior limb, the genu as well as in the PLIC (e.g., thalamocortical sensory fibers intermingle and are tracked with motor fibers). Other deficits may also occur with injuries to PLIC near the CST, including sensory deficits and ataxia [72] (Fig. 14.5).
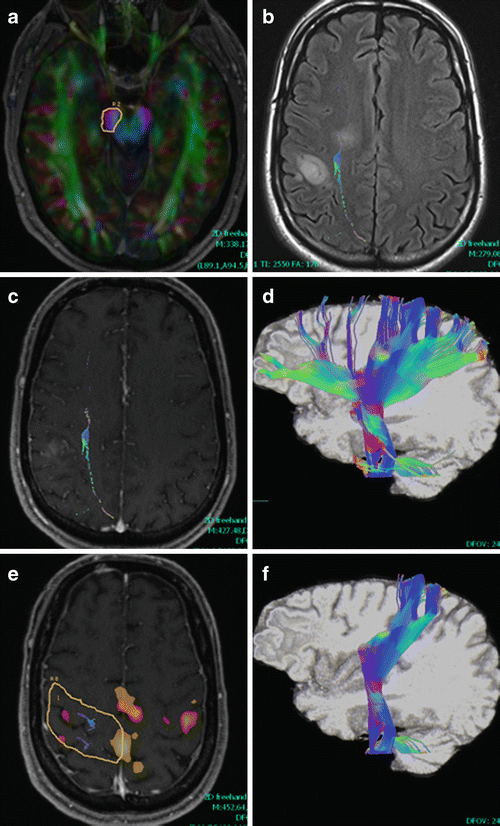
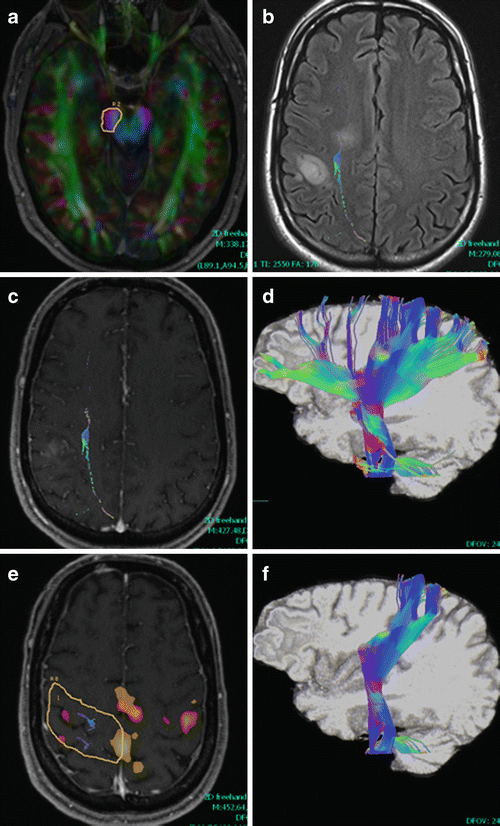
Fig. 14.5
Corticospinal tract (CST). A seed ROI was chosen to include the cerebral peduncle (a), leading to multiple fiber tracts in addition to CST displayed (b, c FLAIR and T1 post-contrast and d, 3D cut plane view). Fibers pass between nonenhancing (anteromedial) and enhancing (posterolateral) parts of a multicentric glioblastoma (b, c). An inclusion ROI chosen based on fMRI (e) around precentral and postcentral gyri was used in this case, resulting in (f). For the fMRI in (e), the red/yellow color scale corresponds to hand motor activation (weaker in right precentral gyrus) and orange/white corresponds to left foot tapping. Both tasks show supplemental motor area as well
Superior Longitudinal/Arcuate Fasciculus
Though not strictly accurate, SLF and AF are commonly used to represent the same fiber tract(s) linking frontal, parietal and temporal perisylvian cortex, and for the purposes of this chapter they are used interchangeably. Typically one triangular ROI places on coronal views is sufficient (Fig. 14.6), though specificity and image quality can be improved by adding a target or inclusion ROI. Disruption of this tract in the dominant hemisphere may lead to not only conduction aphasia but also other deficits including ideational apraxia [72]. In the nondominant hemisphere, injury to the SLF may result in contralateral neglect.
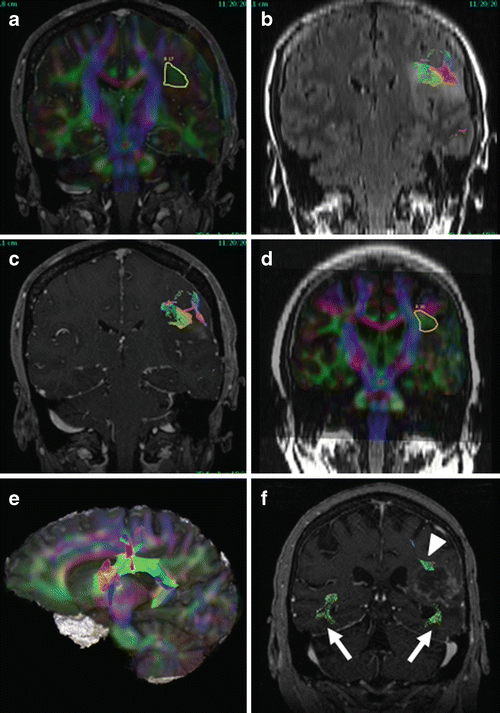
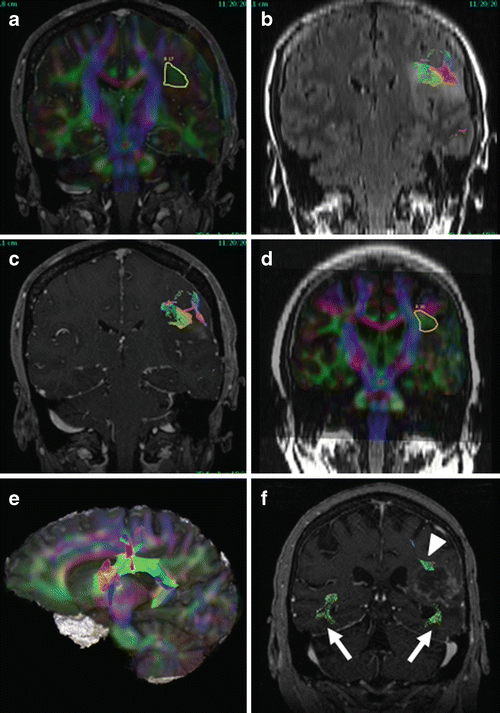
Fig. 14.6
Superior longitudinal fasciculus (SLF). Two cases are shown, one patient with anaplastic astrocytoma (AA, a-c) and the other patient with breast cancer metastasis after whole brain radiation and subsequent stereotactic radiosurgery now with primarily treatment related necrosis (TRN, d-f). Seed ROIs are shown for the left SLF in each case (a, d). For the patient with AA (a-c), decreased FA compared to contralateral side is noted (a), likely reflecting infiltration of SLF fibers by the neoplasm. Fiber tracking was suboptimal anterior and posterior to the mass. For the patient with TRN (d-f), color FA in SLF is more symmetric and tracking was more complete (e, 3D cut plane view), but fibers of inferior longitudinal fasciculus (ILF) and inferior frontal occipital fasciculus (IFOF) were asymmetric (f, arrows). Both SLF and ILF/IFOF tracked in proximity to edge of enhancing mass (arrowhead and arrow, respectively)
Inferior Longitudinal Fasciculus, Inferior Frontal Occipital Fasciculus
These can be seeded together from an ROI in the occipital region when tracts are intact, with inclusion ROIs in the mid temporal lobe and anterior frontal lobe for ILF and IFOF , respectively (Fig. 14.7). If this fails (e.g., extensive occipital lobe pathology), one could use seed ROIs in the mid temporal lobe and anterior frontal lobe instead, tracking posteriorly and using appropriate inclusion ROIs. Injury to the ILF may result in visual agnosia, alexia, and deficits in naming and visual memory. Examples of deficits related to the IFOF include semantic paraphasias and problems with visuospatial processing and visual recognition [72] (Figs. 14.7, 14.8, and 14.9).
