Fig. 9.1
Classification of strut apposition by OCT. (a) Embedded struts (arrows), (b) protruding-covered struts (arrows), (c) protruding-uncovered (arrows) and malapposed struts (arrow heads)
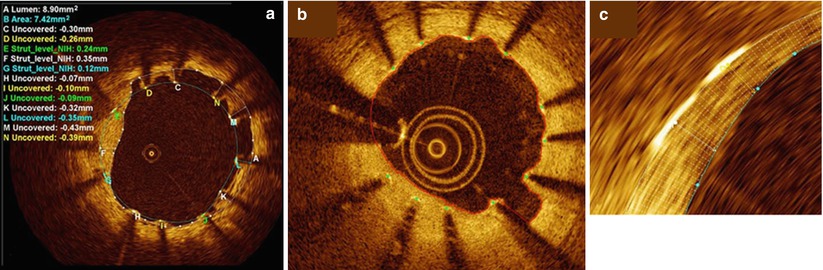
Fig. 9.2
Strut level analysis including qualitative assessment for strut coverage and quantitative measurement. (a) Heterogeneity of strut coverage (C–N refer to labeled struts): covered struts (E–G), uncovered apposed struts (H–J), and malapposed struts (K–N, C, D). (b) Automatic lumen and stent detection: stent struts (green dots) and lumen contour (red line). (c)Magnification of the automatic 360° chord system, applied between the stent and lumen contours (From Bezerra et al. [12])
9.3 OCT Findings During PCI
With parallels to IVUS predictors of stent failure, OCT is useful for precisely assessing stent expansion and edge problems. In addition, OCT is more beneficial for detecting even minor degrees of strut malapposition, tissue prolapse, thrombus, and edge dissections as compared to intravascular ultrasound (IVUS) [13]. Therefore, OCT helps us understand the underlying pathophysiology of coronary artery disease and to make better clinical decisions.
9.3.1 Thrombus
Thrombi in target lesions can protrude between stent struts. As previously described in native coronary lesions, thrombi can be classified as red, white and mixed forms by using OCT.
9.3.2 Tissue Protrusion
Plaque or thrombus protrusion is defined as the projection of tissue into the lumen between stent struts. On OCT, plaque protrusion is characterized by a smooth surface without signal attenuation, whereas thrombus protrusion appears as an irregular surface associated with significant signal attenuation [14]. Tissue protrusion is more frequently observed in the culprit lesion of acute coronary syndrome, because the stent is likely placed over the unstable lesion containing thrombi, lipid-rich tissue or necrotic core. Sometimes, it may be difficult to differentiate tissue prolapsed from other pathologic conditions such as thrombus, neointima, intimal or intra-stent dissection. A significant relationship between OCT-detected tissue protrusion and stent failure has not been demonstrated [15].
9.3.3 Dissection
Dissections are frequently seen especially at the edge of a stent. Compared to IVUS, OCT more sensitively detects even small dissections [14]. Gonzalo et al. evaluated OCT-defined plaque types at stent edges that were related to edge dissection. Edge dissection was more common at distal than at proximal portions. Distal edge dissection was more frequently occurred when the plaque type at the edge was fibrocalcific (43.8 %) or lipid-rich (37.5 %) than when the plaque was fibrous (10 %) (P = 0.009), [15]. Kume et al. evaluated the natural history of stent edge dissection detectable only on OCT (not on IVUS) [16]. The lesions with OCT-dissection, detected only by OCT, showed relatively short maximal length of dissection and were mostly healed without restenosis or thrombus formation at follow-up (median 188 days). When there is no narrowing of the true lumen, an additional procedure may not be recommended for the treatment of dissection. Moreover, acute stent complications such as tissue protrusion, and incomplete stent apposition that were detectable only by OCT, tended to be smaller than those seen on both OCT and IVUS. The majority of OCT-detected minor complications healed or were resolved at follow-up.
9.4 OCT Versus Intravascular Ultrasound for Stent Assessment
Even though IVUS has been the standard imaging tool for the assessment of coronary stents, its poor resolution has limited its evaluation of the morphology of stented lesions. Conversely, OCT has a resolution of 10–20 μm which is much better than IVUS.
When a small degree of in-stent neointima was histologically defined as occupying <30 % of the stent area, diagnostic accuracy of OCT for detecting the small degree of neointima (area under the receiver operating characteristic curve = 0.967, 95 % confidence intervals = 0.914–1.019) was higher than that of IVUS (area under curve = 0.781, 95 % confidence intervals = 0.621–0.838) [17]. The data suggested that OCT is more accurate for identifying neointimal coverage of stent struts. As determined by OCT, the optimal cutoff value of the percent of neointimal hyperplasia to be detected by IVUS was >14.7 %, (sensitivity = 0.887, specificity = 0.790) [18]. For the assessments of neointimal morphology, OCT and IVUS showed a moderate correlation, while approximately 30 % of the lesions showed a discrepancy.
As compared to time-domain OCT, frequency-domain OCT system has higher frame rates that allow faster pullback speeds. As a result, frequency-domain OCT showed a shorter procedural time (3.2 ± 0.8 min vs. 11.2 ± 2.5 min, p < 0.01) and a higher acquisition rate of clear images (99.4 % vs. 80.8 %, p < 0.01) than those of time-domain OCT [19]. Frequency-domain (FD) OCT also showed better detection of incomplete stent apposition (malapposition) or uncovered strut compared with IVUS [20, 21]. Immediately post-PCI, in-stent minimal lumen areas (MLAs) were similar between FD-OCT and IVUS. However, both FD-OCT and time-domain-OCT detected smaller MLAs at follow-up than did IVUS, probably due to the better detection of neointimal hyperplasia by OCT [20]. Post-PCI malapposition and tissue prolapse were more frequently identified by OCT which generates similar reference lumen dimensions but higher degrees of disease severity and intimal hyperplasia as well as better detection of malapposition and tissue prolapse compared with those of IVUS [9]. When compared with histology, the correlation of minimum neointimal thickness was inferior in IVUS (R2 = 0.39) than that in OCT (R2 = 0.67) [21]. Similarly, IVUS showed a weak correlation of the ratio of uncovered to total stent struts per section (R2 = 0.24), whereas OCT was superior (R2 = 0.66). In a more detailed analysis, OCT identification of individual uncovered struts demonstrated a sensitivity of 77.9 % and a specificity of 96.4 % [20].
9.5 Strut Coverage
After stent implantation, intimal hyperplasia is considered a wound healing process following vascular injury [22]. Anti-proliferative drugs inhibit the migration and proliferation of smooth-muscle cells that result in delayed arterial healing and uncovered struts of drug-eluting stents [23, 24].
The low axial resolution of IVUS (100–150 μm) and frequent artifacts around stent struts, preclude IVUS as the proper modality for a precise assessment of vascular microstructures, such as thin layers of neointima over the struts. Conversely, the high-resolution properties of OCT can detect histologically defined minor structures and quantification of thin neointima overlying struts. Strut coverage thickness can be measured as the distance between the luminal surface of the covering neointima and the luminal surface of the strut.
Strut-level analysis with an accurate assessment and quantification of neointimal coverage over stents has been adopted as a surrogate end point in numerous clinical trials of DES and as an important parameter for the safety profile of drug-eluting stents and the approval of new drug-eluting stent technologies.
OCT has been validated as a reliable and reproducible method for assessing neointimal formation and strut coverage. Prati et al. reported on the capacity of OCT for assessing arterial healing after stenting [25]. The histological and OCT measurements of mean neointimal thickness were similar and closely related (r = 0.85, p < 0.001) to an excellent intra- and interobserver reproducibility of OCT measurements (r2 = 0.90 and 0.88, respectively). Murata et al. showed that there was a high correlation between OCT and histology in the evaluation of neointimal area (r2 = 0.804), luminal area (r2 = 0.825) and neointimal thickness (r2 = 0.789) [26]. These results were independent of the stent type. In the ODESSA trial [27], OCT was more sensitive to a small amount of neointimal hyperplasia compared to IVUS. However, OCT has not yet visualized the endothelium.
9.6 In Vivo OCT Data for Stent Coverage
Strut coverage patterns and the degrees of intimal hyperplasia have varied amongst different types of drug-eluting stents. For the first generation drug-eluting stents, a previous study reported that only 16 % of sirolimus-eluting stents showed complete coverage at 6 months post-intervention [24]. Kim et al. demonstrated that the incidence of completely covered stents at 9 months follow-up was 29.6 % for paclitaxel-eluting stents and 9.1 % for sirolimus-eluting stents [28]. Uncovered struts were frequently observed in sirolimus-eluting stents, whereas the pattern of neointima was more heterogeneous in paclitaxel-eluting stents.
Newer generation drug-eluting stents have been developed using a more advanced strut platform, polymer, and new anti-proliferative agents. The frequency of uncovered struts as well as malapposed struts was significantly lower in zotarolimus-eluting stents than in sirolimus-eluting stents (0.3 % vs 12.3 %, p < 0.001 and 0.1 % vs. 2.6 %, p < 0.001) [29]. Resolute zotarolimus-eluting stents also showed higher rates of strut coverage than sirolimus-eluting stents at 9 months follow-up [30]. The percentage of uncovered struts and malapposed struts at 9 months after resolute zotarolimus-eluting stent implantation was only 4.4 ± 4.8 % and 0.1 ± 0.4 %, respectively, which was significantly lower than that seen in sirolimus-eluting stents. Intracoronary thrombus was less frequently detected in lesions treated with resolute zotarolimus-eluting stents than in sirolimus-eluting stents at the 9-month follow-up. Everolimus-eluting stents also showed better vascular responses compared to sirolimus-eluting stents [31]. The percentage of uncovered struts was significantly smaller in the lesions with everolimus-eluting stents than that in lesions with sirolimus-eluting stents (4.4 ± 4.7 % vs. 10.5 ± 12.7 %, P = 0.016, respectively). There was no significant difference in the percentage of malapposed struts between the two groups (0.4 ± 0.8 % in everolimus-eluting stents vs. 1.7 ± 4.5 % in sirolimus-eluting stents, P = 0.344). Biolimus-eluting stents with the unique property of a biodegradable polymer and abluminal coating showed more frequent OCT-defined strut coverage compared to sirolimus-eluting stents at 9 months [32].
With regard to clinical outcomes, delayed neointimal healing of implanted stents was associated with the occurrence of late stent thrombosis. The ratio of uncovered to total stent struts per section >30 % was related to stent thrombosis (odds ratio 9.0, 95 % confidence intervals 3.5–22) [25]. Moreover, the length of an uncovered stent strut segment was significantly associated with stent thrombosis [33]. In a recent OCT study, independent predictors for major adverse cardiac events were post-intervention minimal lumen diameter (odds ratio 0.019, 95 % CI = 0.001–0.513, p = 0.018) and the percentage of uncovered struts ≥5.9 % (odds ratio 19.781, 95 % CI = 2.071–188.968, p = 0.010) [34]. A greater percentage of uncovered struts ≥5.9 % as the cut-off value was significantly associated with a high rate of major adverse cardiac events after DES implantation. More favorable biological properties and vascular responses may contribute to greater clinical safety, which possibly explains the less frequent stent thrombosis after newer generation drug-eluting stent implantation as well as after biolimus-eluting stents, compared to the older generation drug-eluting stents [35, 36].
9.7 Malapposition
In IVUS studies, incomplete stent apposition is defined as a separation of at least one stent strut from the intimal surface of the arterial wall that is not overlapping a side branch, and has evidence of blood flow behind the strut [37]. Apposition of the stent struts to the vessel wall can be seen using OCT by way of its high resolution and capability to identify the tissues surrounding the struts. Malapposed stent struts seen on OCT are defined as the distance between the strut marker and the lumen contour greater than the strut thickness plus the polymer (if present) and the axial resolution of OCT [10]. Thus, the definitions of malapposed struts differ depending on the type of coronary stent and in turn, the thickness of struts and the applied polymer. For the various types of drug-eluting stents, malapposed stent struts have been defined as the struts with detachment from the vessel wall that is: ≥160 μm for sirolimus-eluting stents (Cypher Select™, Cordis, Miami Lake, FL, USA), ≥130 μm for paclitaxel-eluting stents (TaxusLiberte™, Boston Scientific, Natick, MA, USA), ≥110 μm for zotarolimus-eluting stents (Endeavor™ and Resolute™, Medtronic, Santa Rosa, CA, USA), ≥100 μm for everolimus-eluting stents (Xience™, Abbott Vascular, Santa Clara, CA, USA), and ≥130 μm for biolimus-eluting stents (Nobori™, Terumo Corporation, Tokyo, Japan or BioMatrix™, Biosensors Interventional, Singapore) [30, 31, 38]. Apposition can be described on a per-strut, per–cross-sectional area and on a per-stent basis.
With the better resolution, OCT provides a more accurate visualization of stent struts with a small malapposition which is detected by OCT but not by IVUS. As neointimal tissue proliferates over time, the majority of malapposed struts usually disappears or decreases on the follow-up OCT, especially when the malapposition was small [38–40]. The percent of malapposed struts significantly decreased from 12.2 ± 11.0 % post-stent to 1.0 ± 2.2 % at the 6-month follow-up (P < 0.001). In those lesions with minimal malapposition, there was a significant decrease in the mean extra-stent malapposition area from 0.35 ± 0.16 mm2 post-stent to 0.04 ± 0.11 mm2 at follow-up (P < 0.001) [38].
On the other hand, there are persistent malappositions and newly developed late stent malappositions detected at follow-up. A recent study showed that 11 % of the struts with acute malapposition remained malapposed at 8-month follow-up [40]. The mean distance between the strut and the vessel wall of persistent malapposed struts on post-stenting OCT images was significantly longer than that of resolved malapposed struts (342 ± 99 μm vs. 210 ± 49 μm; p < 0.01). Based on receiver-operating characteristic curve analysis, the distance between strut and the vessel wall ≤260 μm seen on post-stenting OCT images was the best cut-off value for predicting resolved malapposed struts (sensitivity: 89.3 %, specificity: 83.7 %, area under the curve = 0.884). Coverage of the incomplete stent apposition and nonapposed side-branch struts is delayed with respect to well-apposed struts in drug-eluting stents, as assessed by OCT at the 9–13 months follow-up after drug-eluting stent implantation [41]. Kim et al. reported that the lesions with the median value of the percentage of malapposed struts ≥1.3 % showed a significantly larger percentage of uncovered struts compared to those with malapposed struts <1.3 % or without malapposition [42]. There was a significant correlation between malapposed and uncovered struts in lesions with malapposed struts ≥1.3 % (r = 0.393, p = 0.016), while no relationship was found in the lesions with minimally malapposed struts <1.3 % (r = −0.007, p = 0.965). A recent OCT analysis showed that the percentage of uncovered struts at 6 months varied significantly with post-stent strut apposition (i.e. 0 % [0, 11.4 %] in embedded, 16.3 % [8.1, 31.3 %] in apposed, and 26.8 % [0, 56.3 %] in malapposed, p < 0.001) [43]. The data suggested that OCT-guided optimization of stent strut apposition enhances the strut coverage seen at follow-up.
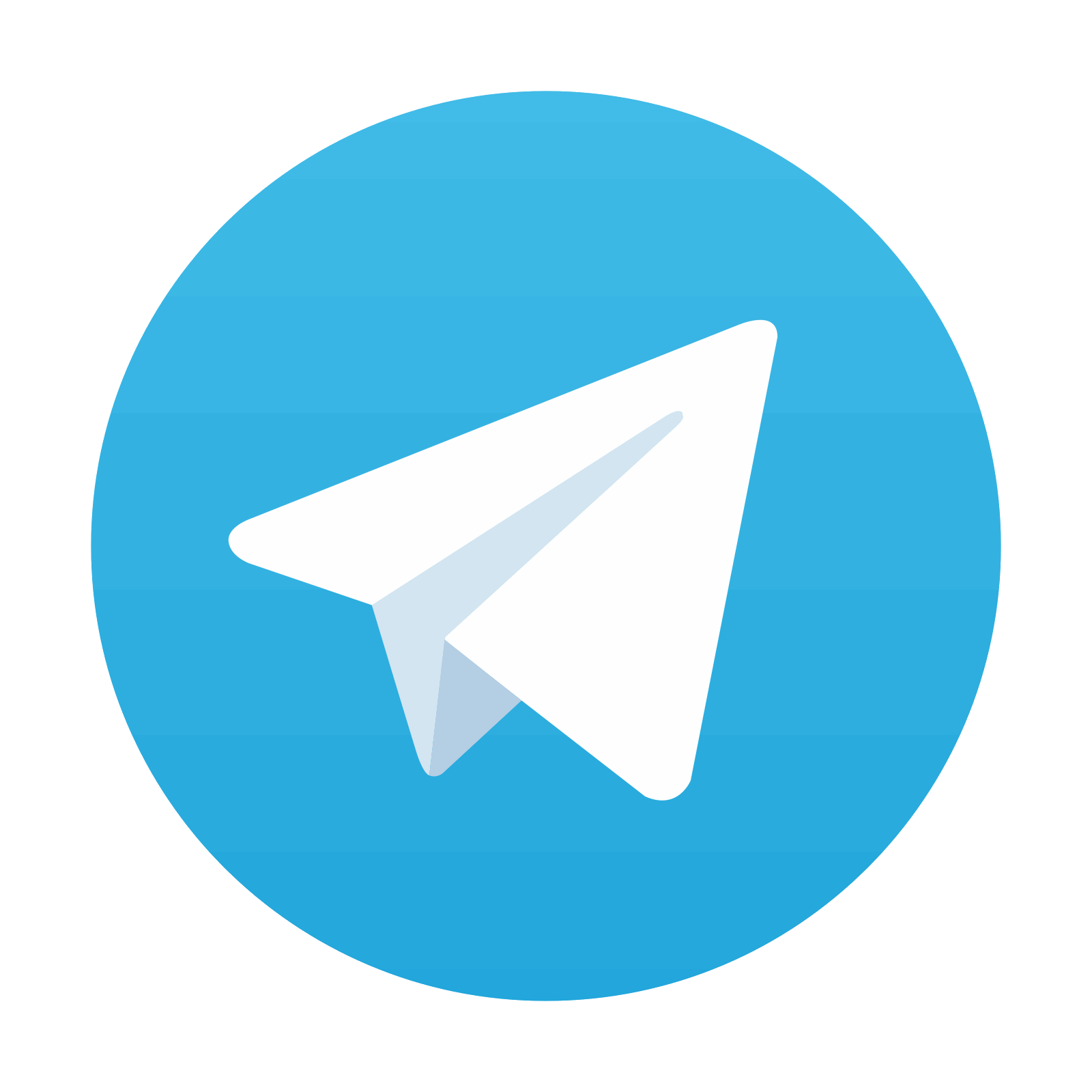
Stay updated, free articles. Join our Telegram channel

Full access? Get Clinical Tree
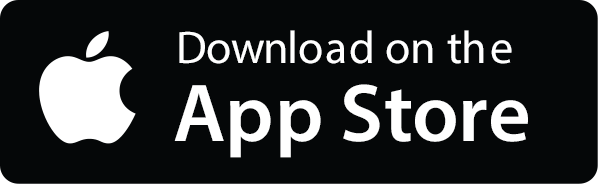
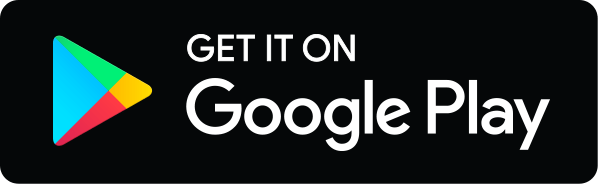