Abstract
Objective
Ultrasound therapy effectively treats a joint range of motion limitation and pain originating from soft tissue in knee osteoarthritis (OA). Few interventional studies have focused on the infrapatellar fat pad (IFP), and the effects of high-intensity continuous ultrasound (HICUS) on IFP stiffness and gliding have not been investigated. Therefore, we aimed to determine the effects of HICUS on IFP stiffness and gliding.
Methods
This single-blind, randomized, controlled crossover study involved 24 healthy participants. The HICUS and placebo conditions were applied to the knee joint on 2 different days. HICUS was performed (1 MHz, 2.5 W/cm², duty cycle 100%, 5 min) using an ultrasound machine equipped with an applicator and adsorption-type fixed automatic rotation irradiation function. The main outcomes were IFP stiffness and gliding measured at 10 degrees and 120 degrees knee flexion. Gliding was measured as the difference between the patellar-tendon tibial angles at 10 degrees and 120 degrees knee flexion. Measurements were performed before (T1), immediately after (T2), and 15 min after (T3) treatment.
Results
Two-way repeated measures analysis of variance showed a significant interaction of IFP stiffness at 10 degrees knee flexion; post-test results showed that HICUS decreased stiffness at T2 and T3. There was no significant difference at 120 degrees. A significant interaction of gliding was observed under the HICUS condition. Post-tests showed that HICUS significantly improved gliding at T2 and T3.
Conclusion
HICUS is a simple, safe intervention for improving IFP stiffness and gliding in healthy participants, with sustained effects. Further studies are needed to evaluate its efficacy in patients.
Introduction
Osteoarthritis (OA) is a systemic progressive joint disease experienced by many adults worldwide [ ]. Pain and stiffness are the most common symptoms of OA [ ]. Pain is associated with decreased activities of daily living, quality of life, and survival [ , ]. The pathology and progression factors of OA have been studied; however, the factors involved in pain remain poorly understood [ ]. Although many conservative therapies have been tested and recommended [ ], they have not been highly satisfactory in the past [ ].
Recently, the involvement of the infrapatellar fat pad (IFP) in anterior knee OA pain has been reported [ , ]. The IFP is an adipose tissue located within the joint capsule outside the synovial membrane of the knee joint and plays a role in supplying nutrients to the joint and absorbing shock during loading [ , ]. However, the IFP is reported to be susceptible to pain [ ], and hypoxia-induced fibrosis is considered to cause intra-articular impingement pain [ ]. The IFP and synovium (SM) are contiguous in the subintimal layer of the SM and part of an anatomic functional unit (AFU) [ , , ]. In previous studies, the IFP of patients with knee OA who underwent total knee arthroplasty showed inflammatory infiltrates and fibrosis compared to that of those with nonknee OA, while lymphocytic infiltrates and SM hyperplasia were observed in the SM adjacent to the IFP compared to healthy individuals [ ]. The IFP of fibrotic knee OAs showed inefficient mechanical behavior compared to normal IFP without being affected by sex or body mass index [ , ]. IFP stiffness and gliding are factors used for IFP evaluation with ultrasonography [ ]. Ultrasonography is an easy-to-use, minimally invasive method in clinical practice. Kitano et al. [ ] and Kitagawa et al. [ ] conducted two randomized controlled trials of physical therapy for human IFP using ultrasonography. Kitano et al. [ ] evaluated the efficacy of a combined intervention of low-intensity pulsed ultrasound (LIPUS) and strengthening of quadriceps and hip abductor muscles in human IFP patients with medial knee joint pain. Kitagawa et al. [ ] also evaluated the effects of hot packs and manual therapy on IFP in healthy university students without knee pain or neurological or orthopedic history of IFP. Additionally, Kitagawa et al. [ ] examined the effects of LIPUS on IFP in a rat model of fibrosis and found that LIPUS suppressed hypoxia-inducible factor 1 alpha activation, a fibrosis transcription factor, and inhibited fibrosis-related factors, including connective tissue and vascular endothelial growth factors. However, LIPUS requires a long irradiation time because of its low power and pulsed waves. Additionally, although its effectiveness in preventing fibrosis has been recognized, its effect on tissues with reduced flexibility is unknown [ , ].
Fibrosis is a condition in which chronic hypoxia causes connective tissue overgrowth and strong collagen fiber cross-linking [ ]. Ultrasound therapy (UST), which has thermal effects, is a type of physical therapy intervention used to improve tissue flexibility [ ]. The advantages of UST are that the frequency can be adjusted to improve collagen fiber flexibility and blood circulation [ ] and to reach deep tissues that cannot be reached by manual therapy or hot packs [ , ]. However, when performing thermal UST, it is necessary to use a moving method to avoid tissue damage caused by hot spots or standing waves. Therefore, it is relatively difficult to appropriately combine manual therapy and exercise therapy with joint movement because the physical therapist must perform applicator manipulation. To address these problems, an ultrasound unit equipped with an adsorption-type fixed applicator and automatic rotation irradiation function was developed. This device is characterized by its autorotation function, which irradiates multiple applicators in turn at a set cycle, thus suppressing standing waves, even though the applicators are fixed. Therefore, applicator manipulation by a physical therapist is no longer necessary, and appropriate manipulation of the affected limb can be performed in combination with the intervention. In previous reports [ ], the UST irradiation area was limited to twice the effective radiating area (ERA), but this therapy device can switch output applicators in 0.1-s increments; consequently, treatment effects can be expected even when irradiating a wider area by using up to five applicators (approximately 5x ERA). Although the effectiveness of conventional UST in improving the joint range of motion and pain originating from soft tissues in knee OA has been reported [ , ], the effect of high-intensity continuous ultrasound (HICUS) administered using an applicator with an adsorption-type fixed autorotation irradiation function on the IFP is unknown.
Recently, ultrasound shear-wave elastography (SWE) was used to evaluate tissue stiffness [ ]. In SWE, a region of interest (ROI) is aligned with a B-mode image, and the shear wave modulus of that region is measured to evaluate the stiffness of a muscle, tendon, ligament, joint capsule, or other tissues [ ]; only Satake et al. [ ] and Katayama et al. [ ] have reported on using this method for evaluating IFP stiffness.
Furthermore, the patellar-tendon tibial angle (PTTA) measured using an ultrasound imaging device is used to evaluate IFP gliding [ ]. The PTTA refers to the angle between the patellar ligament and the anterior margin of the proximal tibial tubercle in B-mode images. The difference in the PTTA measured at different knee joint angles is used to evaluate gliding.
However, the effects of HICUS on IFP stiffness and gliding have not been previously elucidated.
In this study, we aimed to administer HICUS to a relatively wide area of the IFP (frontal, medial, and lateral) and evaluate its effects on IFP stiffness and gliding in healthy human participants. We hypothesized that HICUS would decrease IFP stiffness and improve IFP gliding during joint movement.
Materials and methods
Ethics
This study was approved by the Ethics Review Board for Research Involving Human Subjects of Josai International University (approval number: 14G230050). Per the Helsinki Declaration, oral and written information regarding the purpose, content, benefits, and risks of the study was provided to all participants before the study began, and they provided consent to participate in the study by signing a consent form. The methodological design of this study was based on the 2010 CONSORT statement extension to crossover trials.
Participants
The participants were 24 healthy male adults [mean (standard deviation): age, 20.2 (1.4) y; height, 168.6 (6.2) cm; weight, 64.4 (10.1) kg; body mass index, 22.6 (2.8) kg/m 2 ]. The exclusion criteria were as follows: participants with a history of neurological or knee joint disease, those who could not provide consent to study participation, and those who were deemed unsuitable for study participation by the investigators for specific reasons.
The sample size was calculated using G*power 3.1.9.7 (Heinrich Heine University, Dusseldorf, Germany). The sample size required for a two-way analysis of variance (ANOVA) with repeated measures (effect size = 0.25, α error = 0.05, power = 0.8) was calculated to be 24 participants. None of the participants were excluded because of missing data.
Experimental design
This was a randomized, single-blind, placebo-controlled crossover study. The intervention conditions included the HICUS and placebo conditions (quasi-experimental), which were administered in a randomized order. The RANDOM.ORG web application was used to randomize the intervention order, and the washout period was at least 24 h.
Interventions
An ultrasound unit (USTRON DS-602; Techno Link. Co., Ltd., Niigata, Japan) was used to administer HICUS. The ERA of the applicator was 2.0 cm², and the beam nonuniformity ratio was 1.8. The HICUS conditions were as follows: frequency, 1 MHz; spatial average time-averaged intensity, 2.5 W/cm² (100% duty cycle); and HICUS irradiation time, 5 min. Three multipoint applicators were placed horizontally on the patellar ligament and on the medial and lateral sides of this ligament, and a band on top of these applicators was used to fix them in place; subsequently, they were irradiated automatically ( Fig. 1 ). This treatment device is equipped with an autorotation function that irradiates multiple applicators in turn at a set cycle, preventing standing waves and enabling safe treatment, even with the fixation method. In the placebo condition, the intensity was 0 W/cm² (no ultrasound irradiation); the other procedures were the same as those in the HICUS condition. The HICUS and placebo interventions were administered using sufficient ultrasound gel (LOGIQLEAN, GE Healthcare Co., Ltd, Tokyo, Japan).
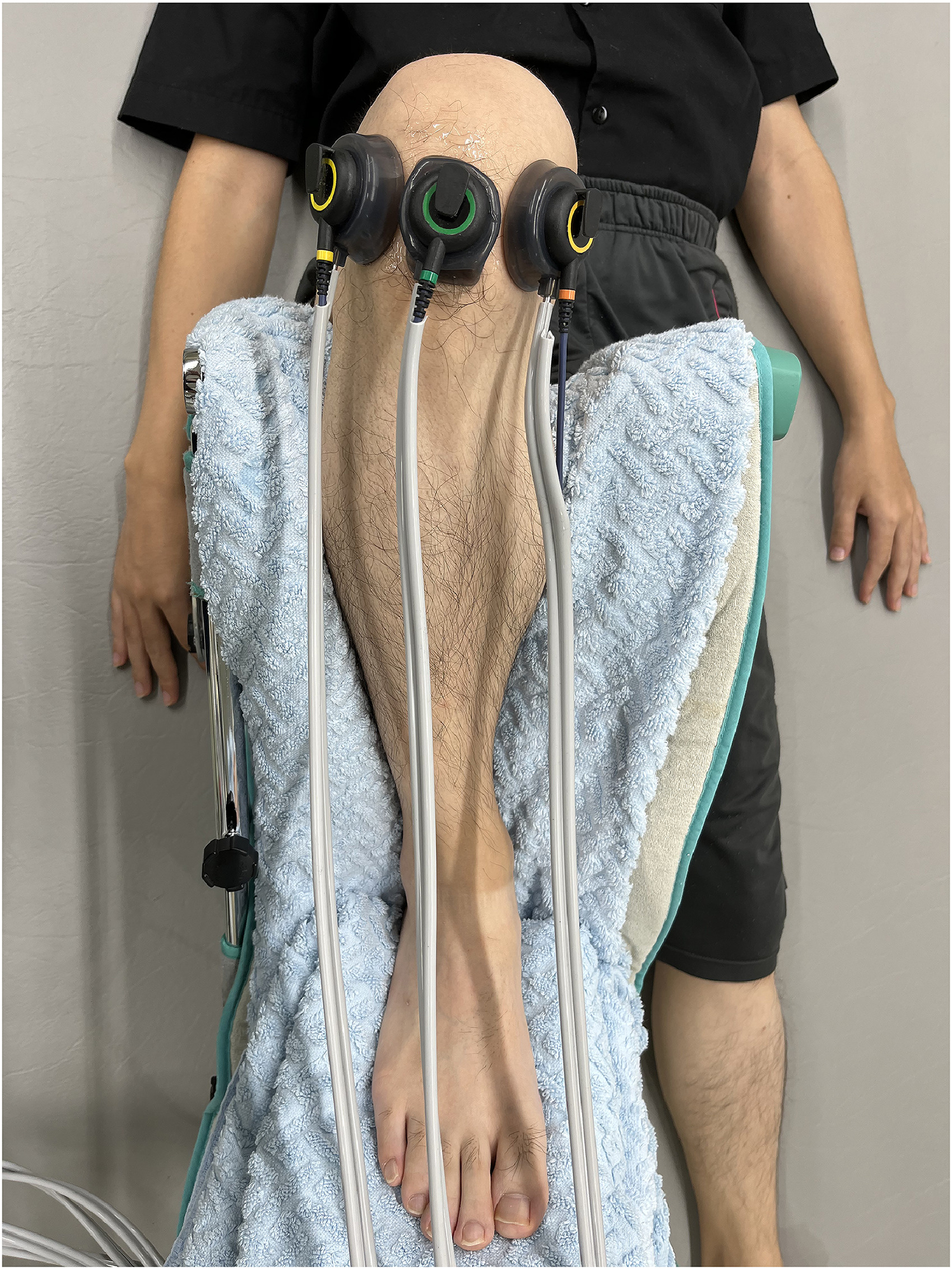
Experimental procedures
The measurement environment was kept as constant as possible [mean (standard deviation): room temperature, 24.1 (1.4)°C; humidity, 55.0 (10.4) %RH]. A 10-min acclimation period was allowed for the participant to acclimate to the measurement room environment before starting the measurement. The measurement protocol consisted of 10 min of acclimation, 5 min of intervention, and 15 min of postintervention rest, resulting in a total duration of 30 min. Measurements were taken at three time points: T1 (before intervention), T2 (immediately after intervention), and T3 (15 min after intervention).
Outcome measures
IFP stiffness measurement
IFP stiffness was measured using the SWE function of a diagnostic ultrasound system (SUPERSONIC™ MACH 30, Supersonic imagine, Aix-en-Provence, France). An L18-5 MHz linear probe (frequency range: 5–18 Hz, MSK, and C-Knee mode) was used for the measurements.
To minimize the effect of compression on IFP stiffness, imaging was performed using minimal compression force to prevent the skin from being distorted in the ultrasound image; uniform imaging settings were used with a B-mode gain of 60 and a depth of 4.0 cm. The measurement positions were 10 degrees and 120 degrees of knee flexion in the supine position. In each measurement position, the knee joint was immobilized by using a continuous passive knee motion device (CPM-SP100; SAKAI Medical Co., Ltd., Tokyo, Japan) to eliminate the influence of muscle contraction.
IFP stiffness was measured by aligning the ultrasound probe with the long axis of the patellar ligament, which extends from the patellar apex to the rough tibial surface. The ROI was set to the maximum possible extent, which included the shallow and deep IFP layers. For the analysis, the analysis radius (circle) was set to cover the maximum possible area of the IFP, which did not include the noisy area or patellar tendon. Measurements were performed twice, and the average value was used as the representative value. Ultrasound imaging was performed by a physical therapist with 21 y of experience in using ultrasound imaging equipment, and the analysis was performed by a different examiner.
IFP gliding measurement
IFP gliding was measured as described in previous studies [ ]. The B-mode diagnostic ultrasound imaging system (SUPERSONIC™ MACH 30, Supersonic imagine, Aix-en-Provence, France) was used to visualize the distal end of the patellar ligament, anterior surface of the proximal tibial epiphysis, and IFP. The PTTA (the angle between the patellar ligament and the anterior surface of the proximal tibial epiphysis) was calculated from the obtained B-mode images using image analysis software (ImageJ, National Institutes of Health, Bethesda, Maryland, USA). The difference between the PTTA at 10 degrees and 120 degrees knee flexion was then used as an index of the gliding ability ( Fig. 2 ). All measurements were performed twice, and the average value was used as the representative value.
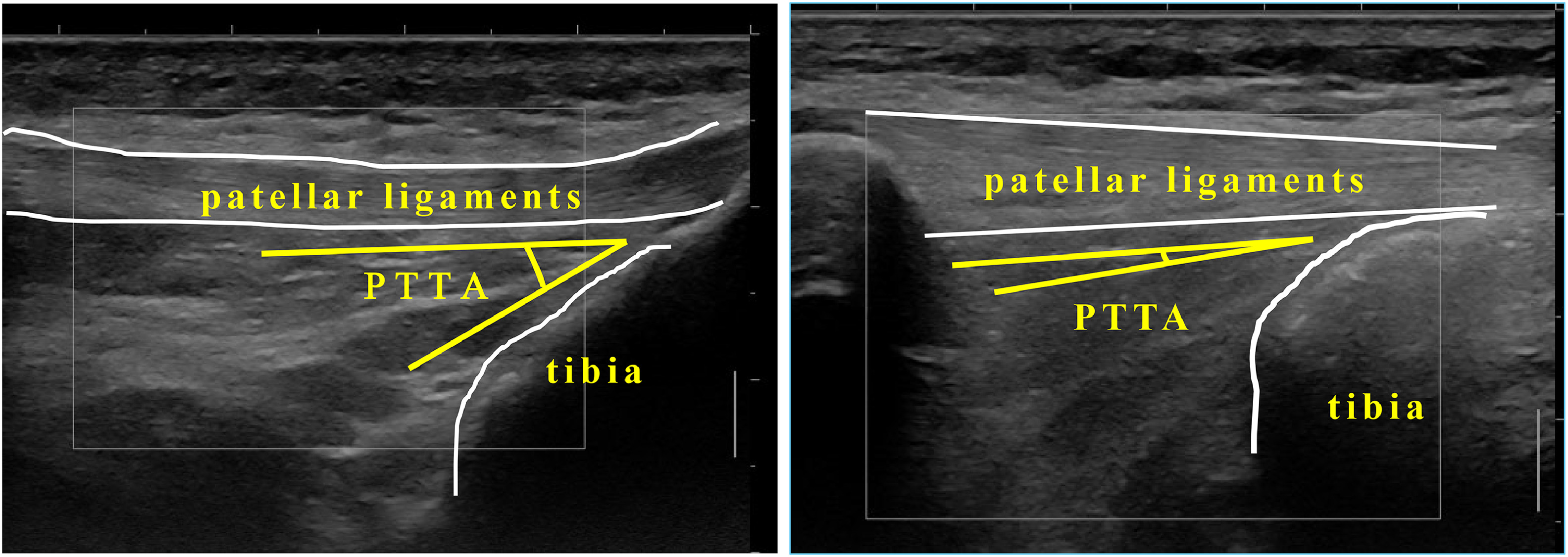
The measurement positions and knee immobilization method were similar to those in the stiffness measurement.
Confirmation of carryover effect
To confirm the presence of a carryover effect, pre-HICUS, and pre-placebo data were compared in terms of IFP stiffness and gliding.
Statistical analysis
The results are presented as the mean (standard error). Statistical analysis was performed using a two-way repeated measures ANOVA (2 × 3), with the intervention condition (HICUS and placebo conditions) and time of measurement (T1, T2, and T3) as the two factors. The Bonferroni method was used for posthoc tests of interaction and main effects. The presence of a carryover effect was analyzed using a paired t-test. The significance level was set at 5%. Intrarater and test-retest reliability in measuring IFP stiffness and gliding was calculated using the intraclass correlation coefficient (ICC). ICC (1, 2) indicates the reproducibility of two measurements taken by one examiner. All statistical analyses were performed using IBM SPSS Statistics 25 for Windows (IBM Corp., Armonk, NY, USA).
Results
Twelve participants were allocated to each intervention sequence, and 24 participants completed the experiment ( Fig. 3 ).
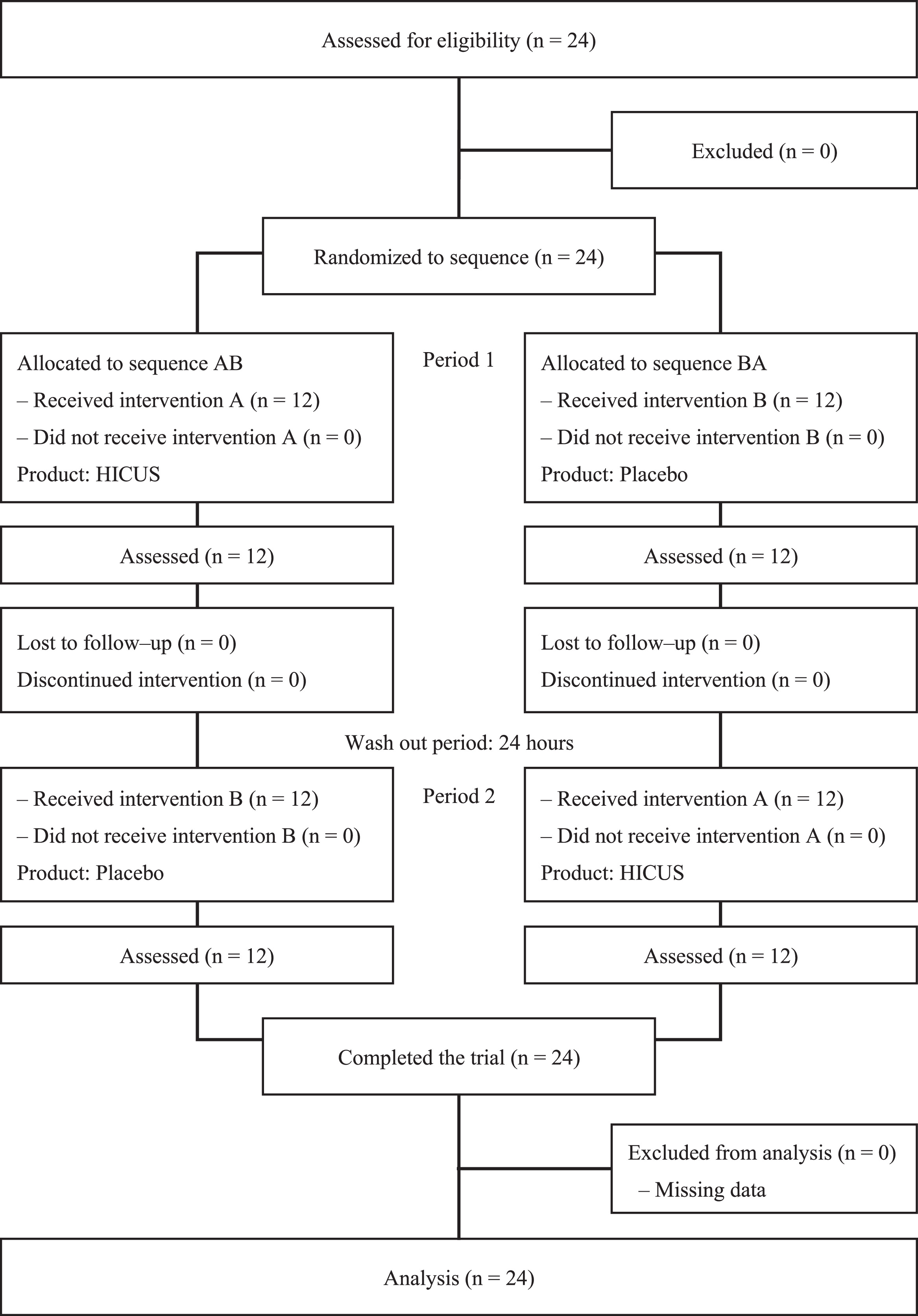
IFP stiffness
The IFP stiffness results are presented in Tables 1 and 2 . The two-way repeated measures ANOVA revealed an interaction between intervention condition and measurement time at 10 degrees of knee flexion (F = 7.57, p < 0.01, ηp² = 0.25). The post-test results showed that IFP stiffness was significantly lesser at 10 degrees knee flexion under the HICUS condition at T2 ( p < 0.001) and T3 ( p < 0.01) than that at T1. No interaction (F = 1.47, p = 0.24, ηp² = 0.06) or main effect was observed between the two factors at 120 degrees knee flexion.
a p < 0.001 (HICUS pre vs. HICUS post).
Pre | Post | Post 15 min | p | F | ηp² | ||
---|---|---|---|---|---|---|---|
HICUS | (kPa) | 46.7 (10.8) | 36.4 (9.3) | 38.8 (10.5) | n.s. | 1.47 | 0.06 |
Placebo | (kPa) | 49.2 (12.1) | 48.7 (11.6) | 46.6 (11.3) |
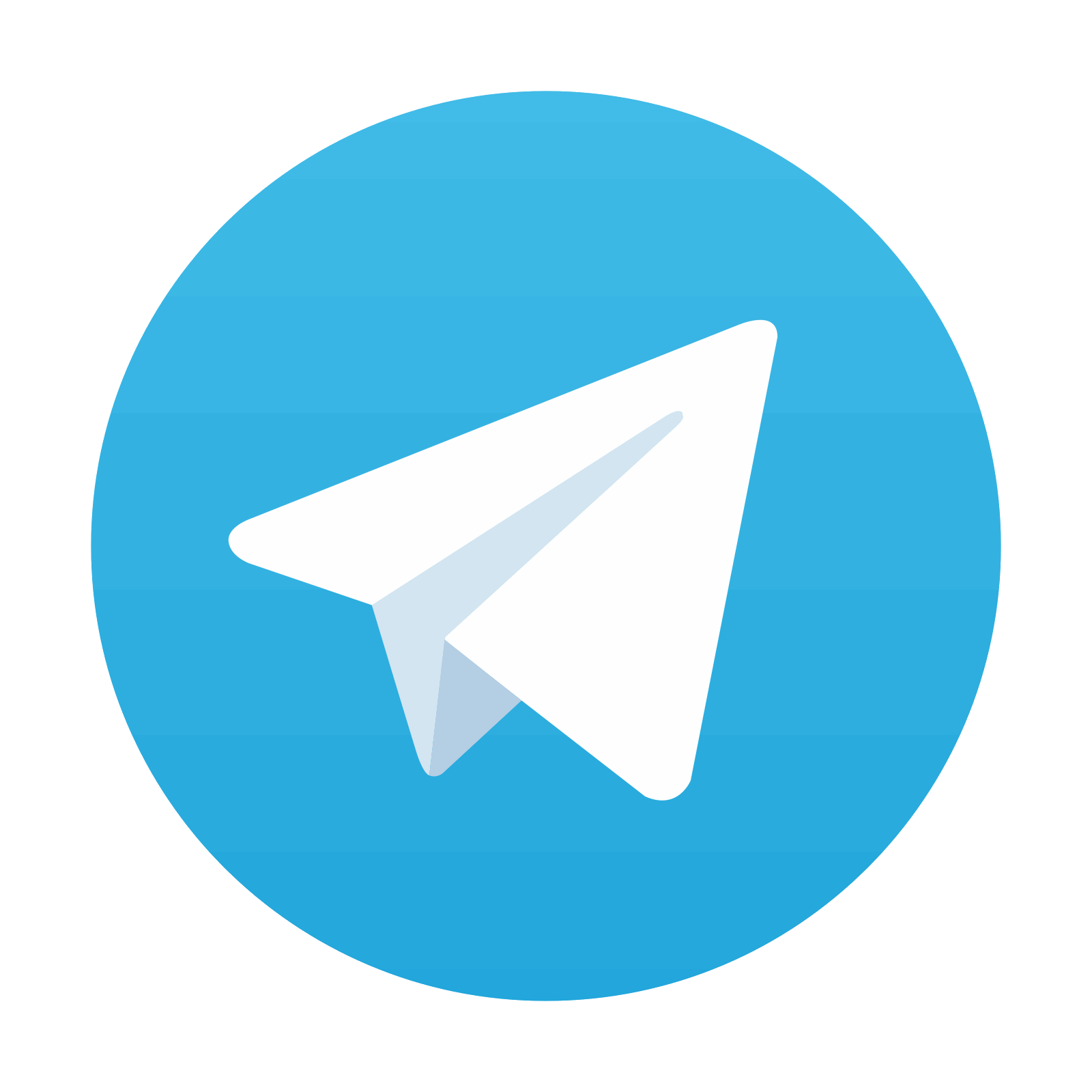
Stay updated, free articles. Join our Telegram channel

Full access? Get Clinical Tree
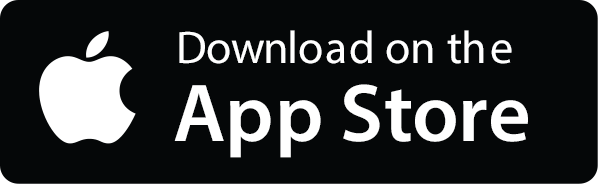
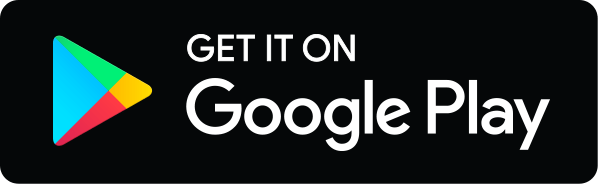
