Introduction
Organ transplantation has become the treatment of choice for end-stage renal and liver disease with the exception of cases with diffuse metastatic liver disease or with hepatocellular carcinoma that has extended beyond the liver. Advances in organ procurement and delivery, human leukocyte antigen (HLA) matching, surgical technique, and immunosuppression regimens have substantially improved both mortality and morbidity and the quality of life following organ transplantation. This chapter reviews the role of ultrasound in the pretransplant evaluation and posttransplant workup of graft dysfunction following renal and hepatic transplantation.
Renal Transplantation
Renal transplantation has become the gold standard treatment for patients with end-stage renal disease. Improvements in surgical technique, coupled with advances in immunosuppressant therapy, postoperative imaging, and clinical graft surveillance have resulted in improved patient and graft survival and improved quality of life when compared with dialysis alone. It is currently estimated that deceased donor (DD) renal transplantation extends the average life expectancy of the recipient by 7 to 10 years and living related donor transplantation by 15 to 20 years. These benefits are reflected in a 31% increase in the number of renal transplants performed in the United States from 1998 to 2007 and an 86% rise in the number of candidates on the renal transplant waiting list. The United Organ Procurement and Transplant Network reported that 16,291 renal transplants were performed in the United States in 2015, and 100,434 patients remained on the waiting list awaiting transplantation.
Despite utilization of living related, living nonrelated, and DD transplants, organ shortage remains the major rate-limiting factor for renal transplantation in the United States. Hence, the criteria for organ donation have been recently extended to include transplantation of kidneys from older and sicker patients. However, transplantation of kidneys from such sources has resulted in an increased incidence and severity of postoperative acute tubular necrosis (ATN), which will be discussed later in this chapter.
Causes of allograft failure are numerous, ranging from rejection to drug toxicity, urinary tract obstruction, and vascular thrombosis or stenosis. However, the clinical presentation of these conditions overlap, and findings such as renal failure, low-grade fever, elevated white blood cell count, and pain are nonspecific. Hence, time course since transplantation, imaging, and ultimately renal biopsy play key roles in determining patient management by differentiating between underlying pathology that requires surgical or percutaneous intervention from conditions that can be managed medically. Ultrasound has become the imaging modality of choice for the evaluation of both the immediate and long-term complications of renal transplants largely because ultrasound is noninvasive, radiation-free, and relatively inexpensive. In addition, ultrasound may be helpful in guiding interventional diagnostic and therapeutic procedures. Other imaging modalities that may be used to evaluate patients following renal transplantation include radionuclide scintigraphy, magnetic resonance angiography (MRA), and computed tomography (CT). These modalities are typically used to delineate further an abnormality first identified on ultrasound imaging or to investigate a particular clinical question following a negative or equivocal sonogram.
Pretransplant workup
Renal transplantation may be performed with HLA-matched DD or HLA-matched living donor (LD) transplants that are either related or unrelated. Survival outcomes are best for LD renal transplants. In addition to the history, physical examination, and laboratory workup, the pretransplant evaluation of the living renal transplant donor generally includes cross-sectional imaging to evaluate the renal anatomy for congenital anomalies and to exclude underlying pathology. The vascular anatomy of the donor kidney is particularly important, as preoperative knowledge of the number, length, location, and branching patterns of the renal arteries aids the surgeon to design the surgical approach. In the past, conventional angiography was the procedure of choice, but technical advances have made computed tomography angiography (CTA) and MRA viable alternatives, both showing good correlation with surgical findings. In general, the left kidney is preferred for harvesting because the left renal vein is longer than the right.
Surgical technique
The renal transplant is preferentially placed in the extraperitoneal space in the right iliac fossa primarily because the sigmoid colon limits space on the left. Intraperitoneal placement may occasionally be performed in children. For DD renal transplants, a small oval portion of the aortic wall surrounding the renal ostium, known as a Carrel patch, is often harvested along with the intact main renal artery and anastomosed in an end-to-side fashion with the external iliac artery ( Fig. 31.1 ). With LD renal transplants, the donor main renal artery is directly anastomosed preferentially end-to-side with the recipient external iliac artery or less commonly end-to-end with the internal iliac artery because an end-to-end anastomosis is more likely to stenose or to thrombose. The main renal artery is implanted into a region of the recipient external iliac artery without significant atherosclerotic burden as determined by palpation or preoperative imaging with noncontrast CT ( Fig. 31.2 ). In the case of multiple renal arteries, a variety of surgical approaches can be used, including multiple individual end-to-side anastomoses, harvest of a larger Carrel patch including the origins of all the main and accessory renal arteries, or creation of a Y-graft from the dominant renal artery to the smaller accessory artery(ies). The main renal vein (MRV) is anastomosed end-to-side with the external iliac vein (see Fig. 31.2E ). Urinary drainage is created via a ureteroneocystostomy, whereby the ureter is tunneled into the dome of the bladder, resulting in an orifice superior to the native ureterovesical junction, although variations such as implantation of the ureter into an interposed bowel segment are also sometimes performed. A temporary plastic stent is commonly placed in the ureter (see Fig. 31.2F ) to reduce the incidence of ureteral stricture and extravasation of urine. The ureteral stent is usually removed between 2 weeks and 3 months after transplantation. The placement of perinephric drains has been reported to decrease the incidence of urinoma formation (personal communication, Yale Transplant Service).
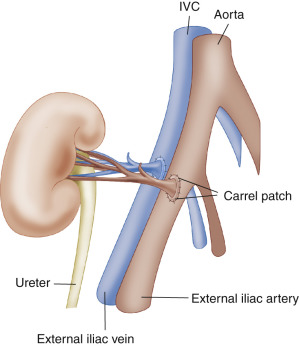
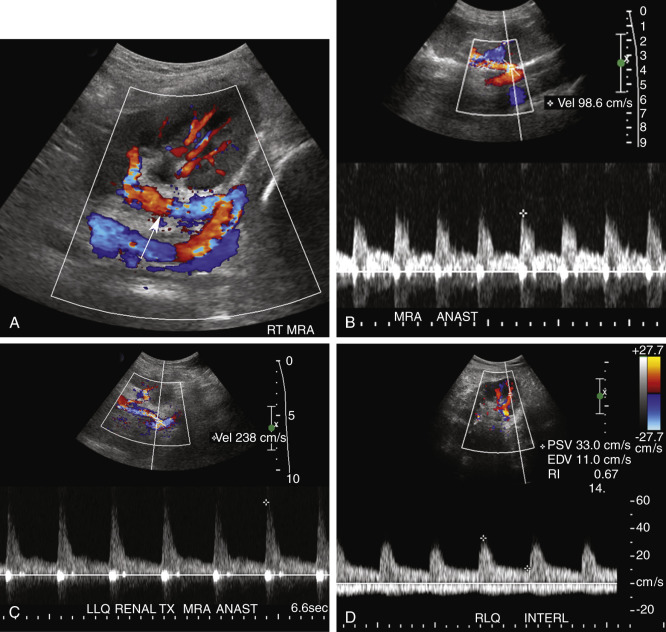
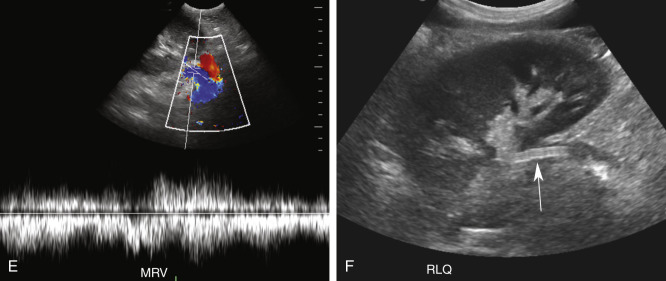
An alternative surgical technique involves the en bloc placement of paired pediatric cadaveric kidneys into adult recipients. With this technique, the donor kidneys are harvested en bloc along with their ureters, arteries, and veins and portions of the aorta and inferior vena cava (IVC). The kidneys are then placed in an extraperitoneal location (typically right iliac fossa) and the suprarenal segments of the donor aorta and IVC are oversewn. The most caudal aspect of the donor aorta and IVC are attached to the recipient external iliac artery and vein, respectively, and the ureters are implanted to the recipient’s bladder, either through separate or a common ureteroneocystostomy ( Fig. 31.3 ). Transplantation of paired pediatric kidneys en bloc into adult recipients not only provides sufficient functional renal tissue to maintain renal function in the adult, but also overcomes potential technical surgical difficulties owing to the small size of the donor pediatric main renal arteries and veins.
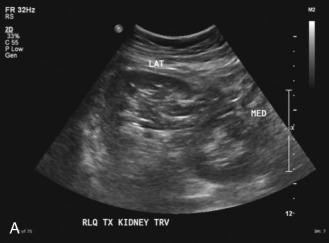
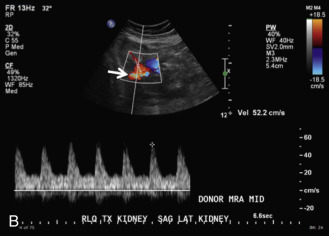
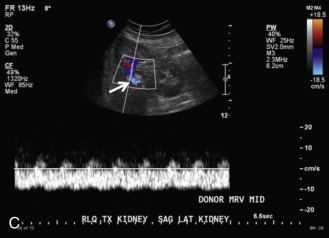
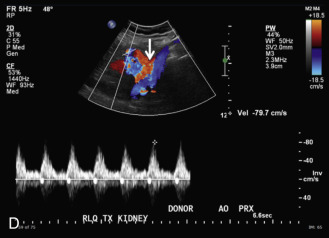
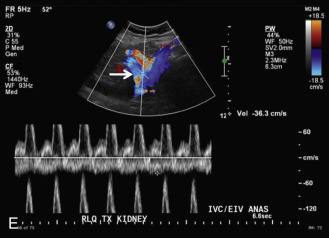
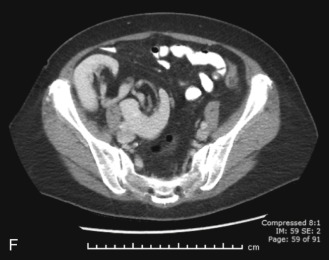
Normal ultrasound findings
The renal transplant is evaluated with gray-scale, color and pulsed Doppler imaging. A relatively high frequency transducer (5 MHz) can often be used owing to the more superficial location of the renal allograft in the extraperitoneal space in comparison with the native kidney. The renal length is measured in maximal sagittal dimension and may increase following transplantation because of compensatory hypertrophy. However, changes in size from one examination to the next may indicate underlying pathology. Cortical medullary differentiation is typically more pronounced with the renal cortex relatively echogenic and the medullary pyramids more hypoechoic in comparison with the native kidney. The renal pelvis is usually slightly dilated, a finding thought to be secondary to multiple factors, including increased volume of urine production (one kidney doing the work of two), denervation of the autonomic nervous system, and perhaps minor dysfunction at the surgical ureterovesical junction. However, dilatation of the infundibulum and/or calyces should raise the suspicion for a distal ureteral obstruction. An evaluation for perinephric collections is also performed.
Color and spectral Doppler evaluation of the renal parenchyma, the main renal artery and vein, including their anastomoses, and the recipient iliac vessels are routinely obtained (see Fig. 31.2A–E ). On color or power Doppler interrogation, a normal transplant should demonstrate parenchymal blood flow arborizing out to the renal capsule without focal areas of decreased vascularity. The peak systolic velocities (PSV) of the MRA and external iliac artery are measured. The PSV in the transplanted main renal artery may be elevated in comparison with the native main renal artery, with an upper limit of normal of approximately 200 to 250 cm/s and possibly as high as 350 cm/s in a few instances, likely because of a combination of factors such as acute angle of takeoff from the external iliac artery, tortuosity of the main renal artery, and increased flow volume. The Doppler spectral tracing of the normal renal artery (see Fig. 31.2B–D ) in the transplanted kidney has a sharp systolic upstroke and continuous forward diastolic flow, reflecting the presence of a low-resistance peripheral vascular bed, and is similar in appearance to the Doppler spectral tracing of a normal native renal artery. The resistivity index (RI) of the intraparenchymal renal arteries is measured at the upper, mid, and lower poles in the segmental, and/or interlobar arteries. Some centers routinely take samples from the segmental, interlobar, and arcuate branches. The normal resistivity index should be less than 0.8 (see Fig. 31.2D ). An RI of 0.8 or greater is considered abnormal. On the other hand, the recipient external iliac artery should demonstrate a high-resistance waveform pattern with absent or reversed end-diastolic flow. The MRV is interrogated to demonstrate patency and exclude renal vein thrombosis (RVT). Peak velocity in the MRV is recorded, and phasicity is commented on. At our institutions, a baseline examination is performed immediately following surgery and subsequently as clinically indicated.
Common parenchymal causes of graft dysfunction
The most common causes of renal transplant failure include ATN, rejection (hyperacute, acute, and chronic), and drug toxicity. Clinical presentation is typically nonspecific except with regard to time of onset. Hyperacute rejection, for example, typically presents in the recovery room, and ATN is most commonly noted within the first 3 days after transplantation, whereas acute or chronic rejection and drug toxicity present later. Similarly, sonographic findings are also nonspecific and unpredictable. Both increased and decreased echogenicity of the renal parenchyma, indistinct but also increased or decreased cortical medullary differentiation, decreased visibility of the renal sinus, and allograft swelling with or without increased cortical thickness have all been described in the setting of most of these entities. Doppler spectral findings may include an elevated RI greater than 0.80, a nonspecific finding of renal dysfunction that likely reflects increased peripheral vascular resistance from a myriad of factors, including any cause of interstitial edema. Conversely, an RI of less than 0.8 does not exclude any underlying pathology. Although serial measurements of the RI may be helpful to determine progression or therapeutic efficacy of a given intervention, biopsy with serologic correlation remains the gold standard for diagnosis of the underlying cause of graft failure.
ATN is the most common cause of graft dysfunction in the immediate postoperative period, occurring in up to 34% of DD renal transplants. Ischemic insult to the renal transplant parenchyma before revascularization is typically the primary cause of ATN. Risk factors include prolonged ischemic time (>24 hours), hypotension or excessive blood loss during surgery, prolonged illness or intensive care unit stay of the donor, a donor without spontaneous cardiac activity, and reperfusion injury. ATN is unusual in live donor renal transplants unless problems, such as hypotension, occur during surgery. In the past, ATN most commonly developed 2 to 3 days postoperatively and was typically relatively mild, resolving with supportive therapy. Although some degree of ATN can persist for up to 3 months, most cases should resolve within 2 to 3 weeks, although 10% to 30% of patients will require temporary dialysis. However, in today’s climate of extended donor criteria for renal transplantation that include kidneys from sicker donors, ATN can occasionally be quite severe and present immediately postoperatively. It is believed that the process of tubular repair increases the risk for later development of acute rejection. Although the surgeon knows preoperatively which donor kidneys are at risk and can recognize a lack of “pinking up” of the kidney in the operating room once it is revascularized, the clinical presentation of renal failure and decreased urine output in patients with ATN is nonspecific. Gray-scale ultrasound findings are nonspecific. The Doppler ultrasound waveforms of the parenchymal renal arteries in a transplanted kidney with ATN often exhibit decreased diastolic blood flow with an increased RI ( Fig. 31.4 ), but this nonspecific finding is also seen in many other causes of graft dysfunction. In general, the higher the RI, the worse the ATN, and reversal of diastolic flow may be observed in extreme cases. An RI greater than 0.73 30 minutes postoperatively has been described as strongly predictive of the development of ATN.
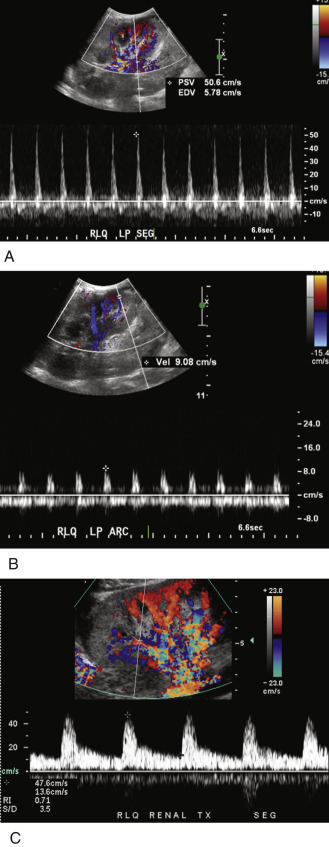
Hyperacute rejection occurs in the immediate (<24 hours) postsurgical period, usually in the recovery room, and is extremely rare in today’s practice because of preoperative HLA matching. Parenchymal blood flow may not be detectable on Doppler interrogation because of intense vasospasm and interstitial edema. Hence, findings may mimic renal artery thrombosis (RAT). If blood flow is seen, diastolic blood flow is typically absent or even reversed, and the RI is markedly elevated.
Acute rejection is estimated to occur in up to 20% to 40% of all renal transplants and usually develops within 1 to 3 weeks to months after transplantation. However, new immunosuppressant regimens have decreased the incidence of acute rejection. Most patients are asymptomatic, but flu-like symptoms, graft tenderness, and low-grade fever along with deteriorating renal function are occasionally reported. Patients may present with a rapid rise (>25%) of serum creatinine level over a 1- to 2-day period. Swelling of the transplant kidney, pruning of cortical blood flow, and an increased RI are nonspecific findings in moderate to severe cases. However, final diagnosis requires biopsy because the ultrasound findings are nonspecific. In most cases, acute rejection can be treated with steroids or by increasing immunosuppression. However, an episode of acute rejection is predictive of future transplant failure.
Chronic rejection manifests itself as a progressive decline in renal function beginning at least 3 months after surgery and may ultimately lead to renal failure. A prior episode of acute rejection is the most common predisposing risk factor. Gray-scale imaging may demonstrate a decrease in kidney length with a thinned and echogenic cortex. The RI may be increased on Doppler spectral interrogation.
Cyclosporine and tacrolimus (calcineurin inhibitors) are extremely effective immunosuppressive agents. However, both are nephrotoxic, causing renovascular constriction and interstitial fibrosis. Reactivation of polyomavirus is associated with these drugs and is reported to cause nephropathy. Because the clinical presentation, time course, and Doppler ultrasound findings of drug toxicity and chronic rejection are indistinguishable, differentiation between these two causes of renal failure requires biopsy and careful correlation with serum drug levels.
Pyelonephritis may cause diffuse or focal areas of either increased or decreased cortical echogenicity. A segmental infarct may cause a similar focal appearance, although an infarct is often more sharply marginated and wedge-shaped. A segmental infarct will also be avascular on color or power Doppler interrogation ( Fig. 31.5 ).
- •
The normal transplant main renal artery PSV ranges from 60 to 200 cm/s although higher velocities can occur as a result of angulation of the renal artery anastomosis or be transiently elevated up to 250 cm/s in the early postoperative period.
- •
Normal transplant renal artery RI is ≤0.8.
- •
An abnormal RI of 0.8 or more can be seen with ATN, acute or chronic rejection, immunosuppressive agent toxicity (i.e., Cyclosporine), obstructive hydronephrosis, or pyelonephritis. Time course related to transplantation and clinical findings guide the differential diagnosis.
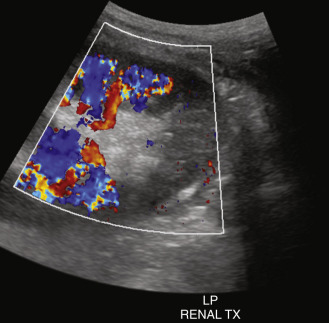
Vascular complications
Doppler ultrasound is considered the primary screening modality for the diagnosis of vascular complications following renal transplantation. Vascular complications occur in less than 10% of renal allograft recipients, but are often treatable causes of graft dysfunction. RAT and RVT tend to occur in the immediate postoperative period, whereas stenoses of these vessels typically develop more than 5 to 6 months following transplantation. Post biopsy complications include arteriovenous fistulas (AVFs) and pseudoaneurysms (PSAs).
RAT typically occurs very early in the postoperative period. Immediate diagnosis is critical so that thrombectomy or thrombolysis can be performed to prevent graft loss. RAT has a reported incidence of less than 1% and is more common in live donor transplant recipients, in patients with complex arterial anastomoses, and in pediatric transplants because of the small size of the main renal artery. Acute and hyperacute rejection, underlying hypercoagulable states, arterial kinks, arterial dissections, vessel size mismatch, ATN, prolonged ischemic time, or faulty surgical technique have all been reported as underlying causes or risk factors for RAT. Clinically, patients with RAT present with acute anuria and tenderness over the graft. On color, power, or spectral Doppler ultrasound, neither arterial nor venous blood flow will be seen distal to the site of occlusion ( Figs. 31.6 and 31.7 ). a
a References .
The kidney may appear relatively hypoechoic and enlarged on gray-scale imaging. Focal or segmental infarcts occur most commonly in the setting of embolus, acute rejection, cytomegalovirus (CMV) infection, vasculitis, thrombosis of a small polar artery with a separate anastomosis, or scarring as a result of injury from the perfusion catheter. On ultrasound, focal infarcts typically manifest as well-defined wedge-shaped to round avascular masses or areas. Echogenicity is variable depending on the time course and whether or not hemorrhage into the infarct has occurred (see Fig. 31.5 ). Focal pyelonephritis may have a similar appearance.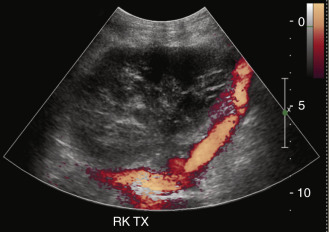
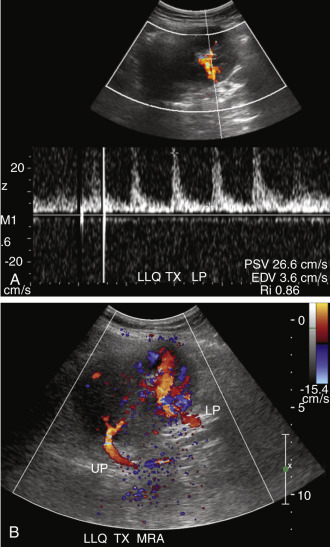
RVT has a reported incidence of approximately 5%. RVT usually occurs within the first postoperative week, and patients most commonly present with abrupt onset of oliguria, graft swelling, and tenderness. If detected early, thrombectomy may prevent graft loss. However, nephrectomy and retransplantation are required if infarction has already occurred by the time of diagnosis. Predisposing risk factors include hypotension, hypercoagulable states, acute rejection, anastomotic stenosis, venous compression from perinephric fluid collections, and propagation of an underlying clot in the iliac vein ( Fig. 31.8 ). RVT occurs slightly more commonly in transplants placed in the left iliac fossa, possibly because of compression of the left iliac vein by the overlying artery and/or aorta. Gray-scale findings are nonspecific and include enlargement of the graft with decreased parenchymal echogenicity. The MRV may be enlarged and filled with hypoechoic thrombus, although acute thrombus can be anechoic. Doppler interrogation will demonstrate absence of venous blood flow associated with increased resistance in the renal arteries often to the point of reversed diastolic blood flow ( Fig. 31.9 ). However, reversal of diastolic arterial flow alone (i.e., without demonstration of concomitant absence of venous flow) is a nonspecific finding and can be seen in patients with hyperacute rejection, severe ATN, and compression of the renal parenchyma by surrounding fluid collections resulting in the Page kidney phenomenon ( Fig. 31.10 ).
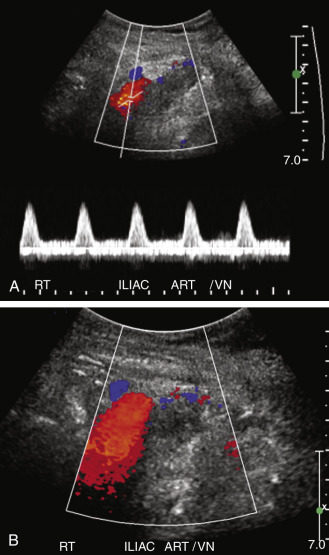
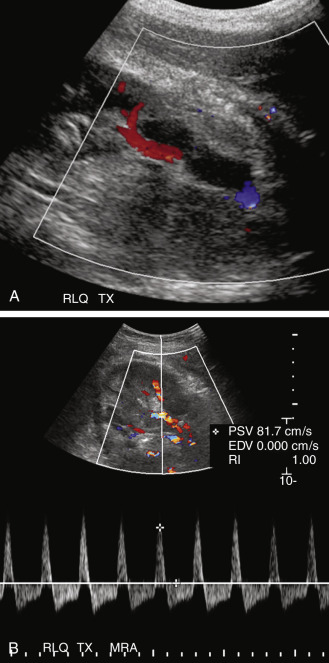
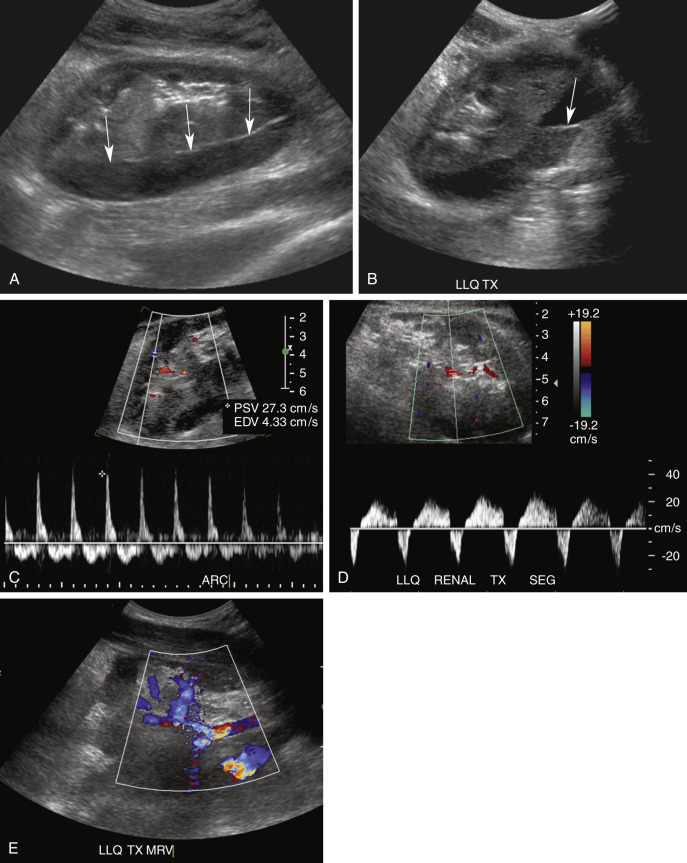
Renal artery stenosis (RAS) is the most common vascular complication in renal transplants, with a prevalence ranging from 1% to 23%. This wide range in the reported prevalence of RAS is believed to be because of a lack of consensus in the definition of a hemodynamically significant stenosis and from differences in diagnostic modalities used to assess for RAS. More recent data obtained from the United States Renal Data System (USRDS) registry suggest a lower incidence of 2% at 3 years, with an overall incidence of 8.3 cases per 1000 patient-years. RAS is more common in live than DD transplants because harvesting of the main renal artery with a Carrel patch likely protects the renal ostium in a DD transplant. End-to-end anastomoses have the highest risk for developing RAS. As with RAT, the incidence of RAS is higher in patients with complex arterial anastomoses and in pediatric transplants. Stenosis generally occurs after 3 months and within the first 1 to 3 years after transplantation. Patients present with new onset or worsening of severe, refractory hypertension. On physical examination, a bruit may be audible over the transplanted kidney. Causes of RAS are postulated to include intimal fibrosis/scarring, faulty surgical technique, vessel injury from the perfusion catheter, allergic reaction to the suture material, and rejection. 2,18–20,27–29 Stenoses occur most commonly at the main renal artery anastomosis. Distal stenoses are infrequent and may be a sign of allograft rejection or prior dissection and/or endothelial damage from the perfusion catheter. In the early postoperative period, compression, kinking, or twisting of the renal artery may cause a functional stenosis, particularly in patients with an excessively long or redundant vascular pedicle (see later). In such cases, stenosis of the MRV with or without elevated Doppler velocities is also usually observed. Stenosis in the native external iliac artery near the arterial anastomosis as a result of atherosclerosis or clamp injury can mimic RAS clinically and on Doppler ultrasound examination. Elevated velocities in the main renal artery may be seen in the immediate postoperative period from arterial spasm/edema and follow-up examination in 3 to 7 days is recommended to exclude significant RAS in the absence of other findings.
On color Doppler imaging, focal color aliasing indicates high-velocity blood flow and localizes the site of the stenosis. Pulsed Doppler criteria include (1) PSV greater than 250 cm/s, (2) PSV ratio of at least 2 : 1 to 3.5 : 1 between the PSV at the stenosis and the arterial segment proximal to the stenosis, (3) poststenotic turbulence (shaggy waveform or “picket fence” abnormality of the arterial waveform immediately distal to the stenosis), and (4) a tardus-parvus waveform in the distal intraparenchymal arteries ( Fig. 31.11 ). a
a References .
However, the PSV at the main renal artery anastomosis is often much higher than the PSV at the origin of the native main renal artery possibly because of tortuosity of a redundant artery, acute angle of take-off from the external iliac artery, or increased flow volume. Therefore some authors advocate using a PSV of 300 to 350 cm/s as a diagnostic threshold for RAS in the transplanted kidney. Even so, increased PSV is not as specific a finding of RAS in the transplanted kidney as it is in the native renal artery. Tardus-parvus waveforms, either identified subjectively or by measuring acceleration time greater than 70 to 100 ms, add specificity to the diagnosis, although the presence of this waveform pattern merely indicates a proximal stenosis, which could be at the level of the external iliac artery, aorta, or even aortic valve. The sensitivity of Doppler ultrasound for the detection of transplant RAS has been reported to range from 87% to 94% with specificities of 86% to 100%, but these vary depending on the specific Doppler criteria. Higher thresholds increase specificity at the expense of sensitivity. Therefore because of the lack of specificity of Doppler ultrasound findings and the fact that most hypertensive renal transplant recipients do not have RAS, confirmatory imaging with non–contrast-enhanced MRA or catheter arteriography is often suggested. Administration of gadolinium is generally avoided in renal transplant recipients, especially those with reduced renal function, because of the risk of nephrogenic systemic fibrosis. Similarly, iodinated intravenous contrast is also avoided because of the risk of developing contrast-induced nephropathy and renal failure. RAS may be treated via percutaneous transluminal angioplasty, arterial stenting, or less commonly, surgical revascularization.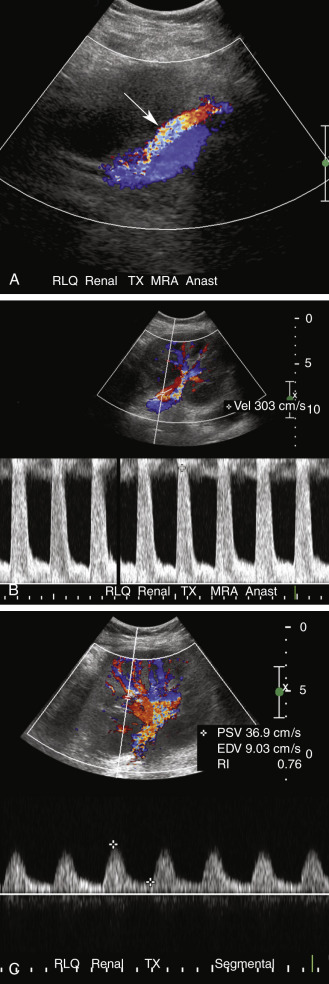
Renal vein stenosis (RVS) is an uncommon vascular complication and is usually caused by extrinsic compression from an adjacent perinephric fluid collection or perivascular fibrosis. Color Doppler and duplex ultrasound findings include narrowing of the vessel with focal color aliasing and high velocities in the region of the stenosis ( Fig. 31.12 ). No specific Doppler or gray-scale criterion has been established to grade RVS, and the clinical significance of narrowing of the renal vein is debatable, although a threefold to fourfold increase in velocity at the stenosis has been reported as possibly clinically significant in a patient with renal dysfunction. By imaging criteria alone, it may be difficult to differentiate a true RVS from a caliber mismatch between the donor and recipient vein. Stenting is the treatment of choice.
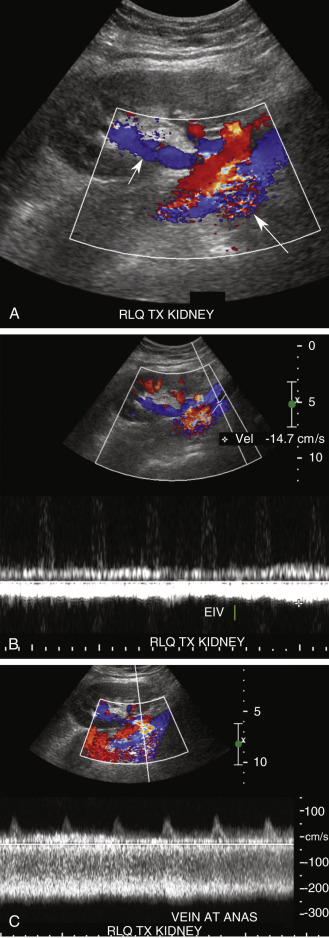
Renal cortex biopsies are frequently performed to establish the etiology of allograft dysfunction. Complications of this procedure include hematomas, AVF, and PSA. Most perinephric hematomas seen following transplant biopsy are small and do not require treatment unless they are expanding and/or compressing the renal parenchyma or vascular pedicle. Most PSAs and AVFs are also small, clinically insignificant, and will resolve spontaneously. PSAs greater than 2 cm in diameter and large AVFs resulting in renal ischemia, hematuria, or congestive heart failure will require percutaneous embolization. The incidence of clinically important PSAs and AVFs is significantly reduced if ultrasound guidance is used during the biopsy because it avoids laceration of the larger segmental arteries and veins in the renal sinus. A tangential approach through the cortex is safest. Extrarenal AVFs and PSAs are usually a result of faulty surgical technique, breakdown of the anastomosis or infection, and generally require intervention because of their large size and risk for rupture ( Fig. 31.13 ).
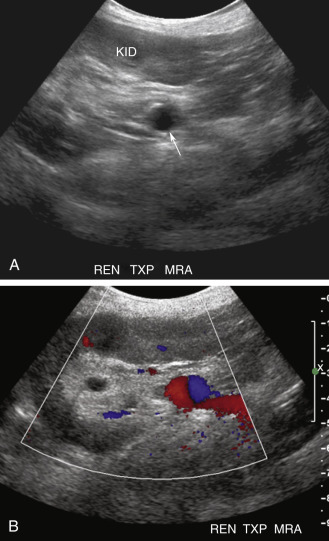
Damage to the artery and vein may result in an AVF between these two structures. Color Doppler imaging may demonstrate disorganized color signals outside the normal borders of the vessels. This has been termed a soft tissue bruit and is attributed to perifistula soft tissue vibration. Focal color aliasing will also be observed as a result of high-velocity blood flow within the feeding artery and the fistulous connection. Doppler spectral interrogation demonstrates a characteristic high-velocity, low-resistance waveform within the feeding artery and pulsatile high-velocity flow in the draining vein ( Fig. 31.14 ). Dilatation of the draining vein may mimic a cystic structure on gray-scale imaging.
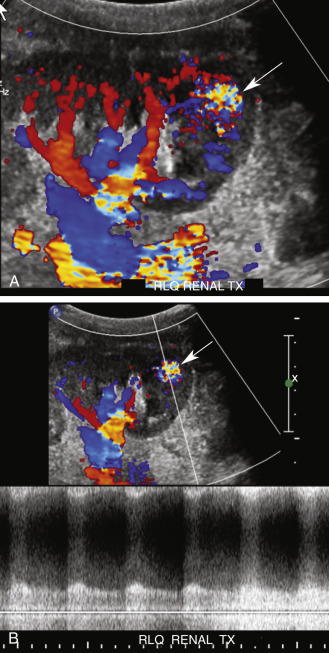
Blood escaping through a puncture in the arterial wall may lead to formation of a PSA. Extra-arterial blood is contained by a pseudocapsule formed by compression of the surrounding soft tissues. Gray-scale imaging will demonstrate an anechoic cyst-like structure that may contain intraluminal thrombus. On color Doppler imaging, a “yin-yang” pattern (red on one side and blue on the other) of swirling blood flow will be seen in the PSA. A characteristic to-and-fro pattern with blood flow above the baseline heading toward the PSA in systole and reversed blood flow, below the baseline, heading away from the PSA in diastole will be observed in the channel connecting the PSA to the artery, also called the neck ( Fig. 31.15 ).
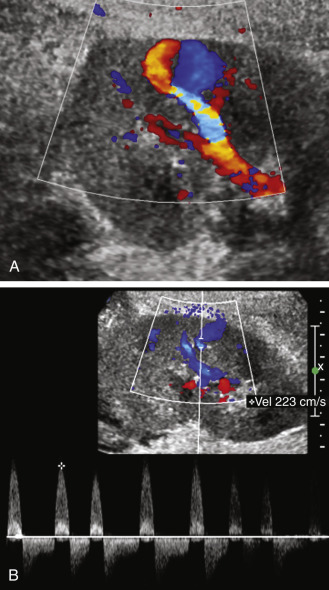
Torsion of the vascular pedicle is a rare complication occurring when the transplant is placed within the peritoneal cavity or when the main renal artery and vein are excessively long and redundant. Torsion presents early in the postoperative period and may be complete or partial. Clinical signs are nonspecific, with patients complaining of abdominal tenderness and swelling. Early recognition of this complication is of paramount importance as surgical revision is necessary to improve blood flow to the transplanted kidney and to prevent the development of RAT, RVT, or ATN, although long-term outcomes remain poor. Minimal degrees of torsion may resolve spontaneously as the kidney settles in the iliac fossa and surrounding swelling/hemorrhage dissipates. Gray-scale imaging may demonstrate malrotation of the kidney with the hilum pointing anteriorly rather than posteriorly. Twisting of the ureter may result in hydronephrosis and thickening of the uroepithelium. Doppler spectral waveforms taken in the renal arteries are variable, ranging from normal to showing reversed diastolic flow. Torsion should be suspected in the immediate postoperative period if increased blood flow velocity is noted in adjacent portions of the main renal artery and vein distal to the anastomoses. If torsion is complete, Doppler findings may be indistinguishable from RAT and RVT, and no blood flow signals will be detected in the transplant. Decreased peak systolic velocities in the transplant arteries may indicate inflow obstruction requiring repositioning of the kidney.
Compression of the kidney by overlying subcutaneous tissue or placing a large kidney in a small iliac fossa (which is more likely to occur in pediatric transplants) may also reduce parenchymal blood flow resulting in a decrease of peak systolic and end-diastolic velocities in the transplant renal arteries. If compression of the kidney is associated with decreased renal function or a markedly increased RI, the kidney may need to be unroofed or placed within the peritoneal cavity.
- •
Normal transplant artery:
- •
main renal artery PSV from 60 to 200 cm/s
- •
normal RI is ≤0.8
- •
- •
Indicators of transplant RAS:
- •
PSV of 250 cm/s or more
- •
a PSV ratio greater than 2 : 1 to 3.5 : 1 between the site of the stenosis and the normal renal artery segment
- •
an acceleration time longer than 70 to 100 ms (0.07 to 0.10 s)
- •
poststenotic turbulence
- •
tardus-parvus waveform in the intrarenal arterial branches
- •
- •
RVS:
- •
likely as a result of extrinsic compression by a fluid collection
- •
a velocity elevation of a factor of 3 to 4 at the site of compression/stenosis
- •
- •
Transplant PSA:
- •
yin-yang pattern in vascular mass
- •
to-and-fro blood flow in the communicating neck
- •
- •
Transplant AVF:
- •
high-velocity and low-resistance blood flow in the main renal artery
- •
arterialized blood flow pattern in the MRV
- •
Perinephric fluid collections
Perinephric fluid collections are extremely common and include hematomas, seromas, lymphoceles, urinomas, and abscesses. Because ultrasound is useful in delineating the size and presence of a fluid collection and the significance of any mass effect on the renal allograft or vascular pedicle, the gray-scale and color Doppler appearances are nonspecific. Differentiating between these entities is typically based on the time course of presentation and symptoms, although definitive diagnosis may require percutaneous aspiration. These fluid collections may become infected and form an abscess. Hence, correlation with white blood cell count, fever, and tenderness is critical, although signs and symptoms of infection may be masked by immunosuppression.
Small postoperative perinephric hematomas are extremely common, perhaps ubiquitous, and most resolve spontaneously. Hematomas may also occur following biopsy or pelvic trauma. Large or expanding hematomas may compress the vascular pedicle or ureter and can result in vascular compromise or hydronephrosis, respectively ( Fig. 31.16 ). A subcapsular hematoma may compress the renal parenchyma ( Fig. 31.17 ), resulting in renal dysfunction or ischemia, and require aspiration or surgical debridement. Rarely, the entire kidney may be compressed by a surrounding hematoma resulting in a Page kidney with renal ischemia and/or hypertension. In this setting, the increased peripheral vascular resistance caused by extrinsic compression of the renal parenchyma can diminish or even reverse diastolic flow in the parenchymal renal arteries (see Fig. 31.10 ). On gray-scale imaging, acute hematomas are usually echogenic, becoming more anechoic and complex over time (see Figs. 31.10, 31.16, and 31.17 ). Layering of blood products with a “hematocrit level” (see Figs. 31.10 and 31.16 ) may occasionally be seen, most often in the acute setting. A subcapsular collection will distort, compress, or scallop the renal parenchyma (see Fig. 31.17 ). The subcapsular collection will not demonstrate internal vascularity on Doppler interrogation.
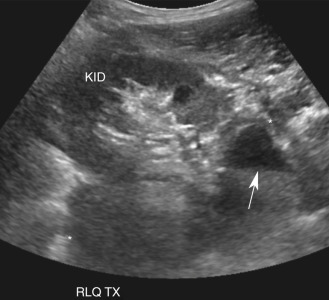
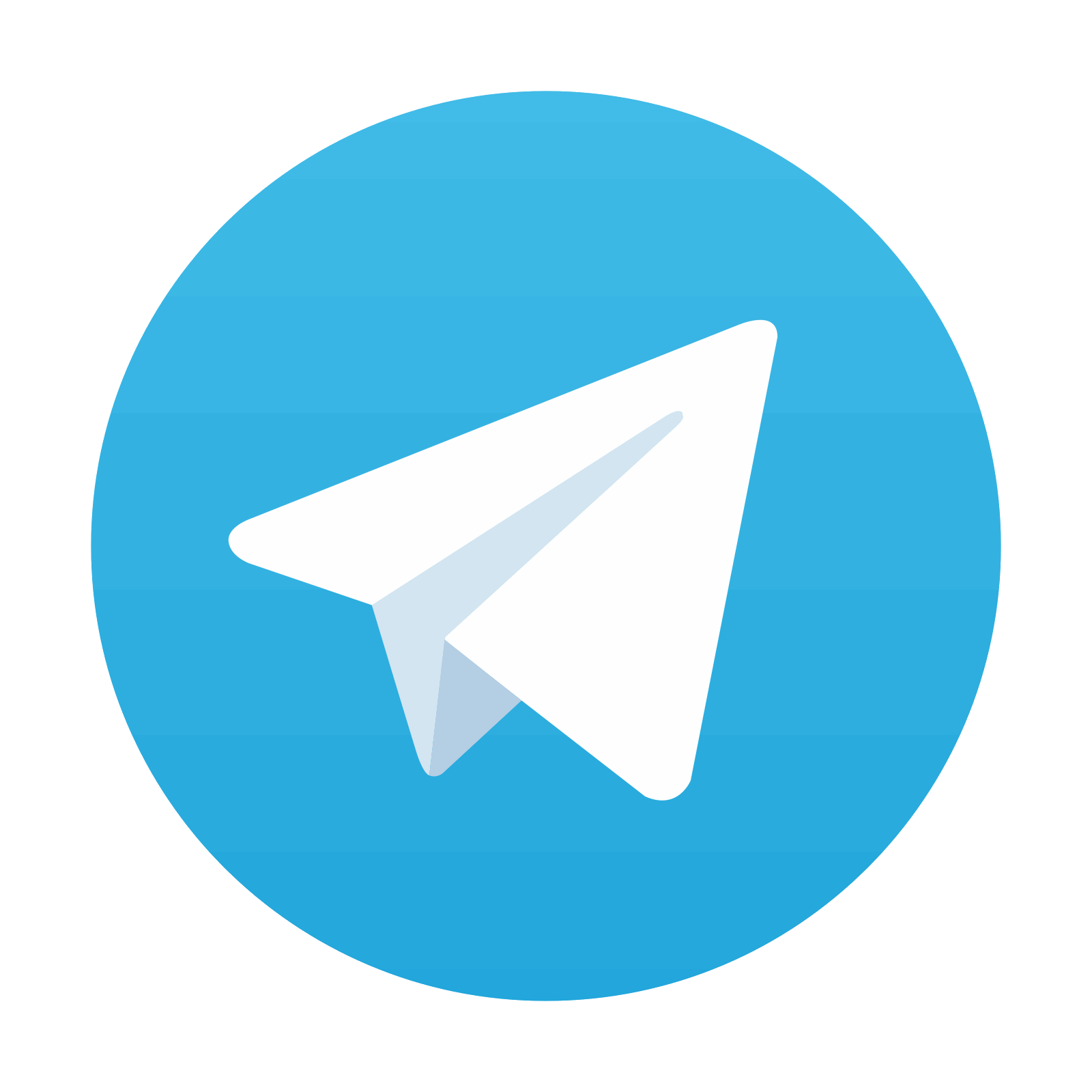
Stay updated, free articles. Join our Telegram channel

Full access? Get Clinical Tree
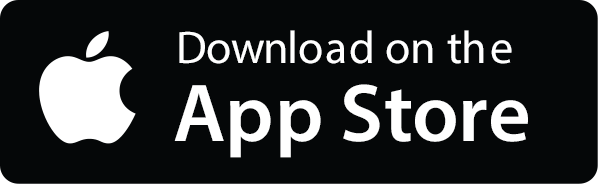
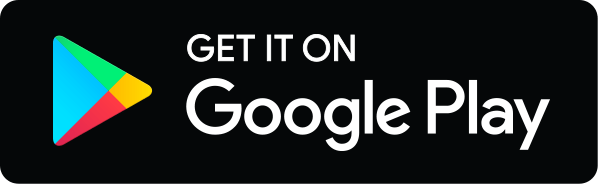
