Fig. 1
Hypervascular liver metastasis (arrows) from an esophageal carcinoma depicted with B-mode gray-scale ultrasound (a) and contrast-enhanced ultrasound 16 s (b), 23 s (c), and 3 min 40 s (d) after injection of 2.5 ml SonoVue® (Bracco Imaging, Milano, Italy) and 10 ml NaCl-flushing. Note the increased conspicuity of the lesion especially in the delayed phase (d) as compared to plain B-mode ultrasound. The dynamic study shows the feeding vessel (small arrows) with an early peripheral enhancement (b) and ongoing complete enhancement of the lesion with two small necrotic spots (c), which are spared from enhancement. In the delayed phase (d) strong wash-out has taken place, being typical for liver metastases
Together with CT and with liver-specific MRI contrast-enhanced sonography in daily practice represents a modality that allows for the confident assessment of lesion vascularity and increases the detection of focal liver disease. Nowadays, the technique is also widely accessible.
2.2 Computed Tomography
Computed Tomography (CT) has developed dramatically with the introduction of the multi-detector technology and with newly explored features like dual energy CT. Especially the abdomen, where motion artifacts due to respiratory motion and bowel peristalsis are disturbing, takes great advantage from these techniques. While scanners with 64 or more detector rows are still most common in large community or university hospitals, scanners with 2–16 slices are widely available even in private practice or in small hospitals. With the introduction of multi-detector CT (MDCT) bi- or even tri-phasic examinations of the liver can be combined into a thoraco-abdominal CT examination without compromises with regard to spatial or temporal resolution. The acquisition of the liver with a 64-slice scanner for example only requires a few seconds despite a submillimeter collimation. Even patients with a compromised general state of health are able to tolerate these breath-hold times. However, even on single slice spiral CT scanners adequate image quality of the liver can be obtained. However, combination with thoraco-abdominal examinations is not possible without compromise in temporal and spatial resolution.
Recently developed PET/CT—scanners, which combine the advantages of PET (functional imaging with high sensitivity), with the advantages of CT (morphological imaging with high spatial resolution) within one examination and nearly perfect image co-registration are with regard to imaging in oncological patients a real contribution. The value of PET-CT for pretreatment evaluation of patients or for response evaluation will be discussed in “Nuclear Medicine Procedures for Treatment Evaluation” and “Radiological Detection and Assessment of Tumor Response” respectively.
Adequate examination technique is critical for sensitive detection and specific characterization of focal liver lesions. A bi-phasic examination of the liver with a late-arterial and a porto-venous phase can be regarded as standard today. For specific indications like the follow-up of hepatocellular carcinoma (HCC) after transarterial chemoembolization (TACE) or for the depiction of the arterial vessels prior to angiography an early arterial phase scan, which can be post-processed into a CT-angiography, is helpful (Fig. 2). The value of delayed scans (e.g., 5 min after contrast agent injection) is controversial in the literature; mainly centers with a focus on imaging in liver cirrhosis consider the use of late phase images as necessary whereas other authors see no added value for it (Hwang et al. 1997; Schima et al. 2006).
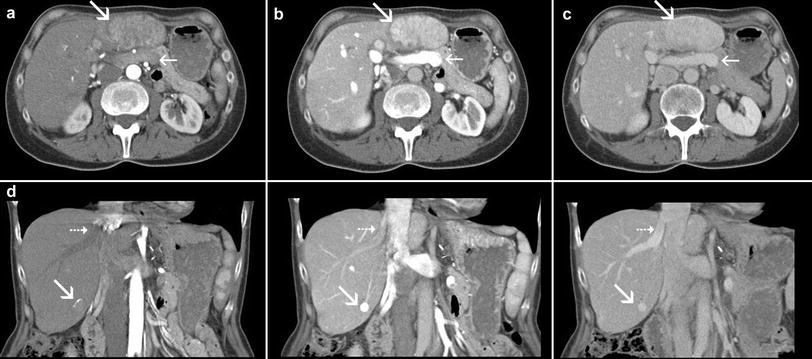
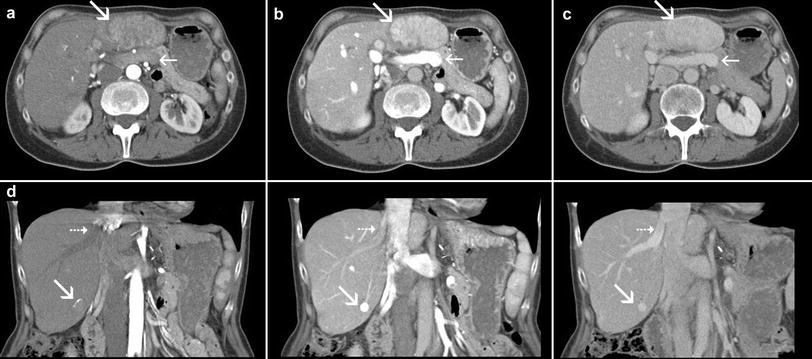
Fig. 2
Axial and coronal sections in the early arterial phase (left), late-arterial phase (middle), and porto-venous phase (right) in a female patient suffering from a hepatocellular carcinoma (HCC) under treatment with transarterial chemoembolization (TACE). In the early arterial phase only the liver arteries are properly enhanced, the portal vein and also the liver parenchyma are not yet opacified. The two HCC nodules in segment 2/3 and segment 6 (marked by large arrows) are also both not properly demarcated. The early arterial phase is, therefore, not suitable for detection of hypervascular tumors; it is rather a CT angiographic phase and can be omitted in most cases. The most important phase for detection of hypervascular tumors is the late-arterial phase (also called arterial-dominant phase or phase of porto-venous inflow). In this phase there is already enhancement in the portal vein (small arrow in the upper row) and in the liver parenchyma. The liver veins are not yet opacified in this phase (small arrow in the lower row). Most hypervascular tumors reach their highest attenuation in this phase. In the porto-venous phase enhancement of the liver parenchyma is highest, the vascular enhancement in the portal-venous system and in the hepatic vein is similar. Hypervascular tumors show decreased attenuation compared to the late-arterial phase, depended on the degree of wash-out they can be still hyperdense (as in this case), isodense (see Fig. 4), or even hypodense
With the short acquisition times of MDCT, contrast agent timing has become critical, since the optimal enhancement phase has to be included within a very short acquisition window. Therefore, the use of modern contrast agent power injectors and bolus timing are mandatory (Schima et al. 2005). For bolus timing automatic bolus triggering is recommended which is available from all CT vendors. Non-ionic iodine-based contrast agents with a concentration of 300–400 mg iodine/ml have become standard. Depending on the iodine concentration fast flow rates up to 5 or 6 ml/second are recommended (Schima et al. 2005). The dosing of the contrast agent should be related to the body-weight with 1.5–2 ml per kg body-weight (for a concentration of 300 mg iodine/ml) (Baron 1994). Whereas the enhancement in the arterial phases can be optimized with help of high-concentration (370–400 mg iodine/ml) contrast agents, the enhancement in the porto-venous phase is not dependent on the iodine delivery per time but rather on the total amount of iodine (Brink 2003). With dual-head power injectors a saline-chaser of 30–50 ml can be injected directly after contrast agent injection allowing for a somewhat reduced contrast agent dose despite optimal contrast enhancement in the arterial phase (Schoellnast et al. 2003).
For focal liver lesions, detection rates of biphasic spiral CT typically ranging from roughly 60 to 75 %, and differentiation of benign and malignant lesions in around 70 % have been reported in literature (Kondo et al. 1999; Semelka et al. 1999; Reimer et al. 2000; Bartolozzi et al. 2004; Oudkerk et al. 2002). CT (even with recent MDCT technology) has shown to be inferior with regard to lesion detection (and lesion characterization) in trials which compared it directly to Gadolinium-enhanced MRI (Semelka et al. 2001) or liver-specific MRI (Reimer et al. 2000; Bartolozzi et al. 2004; Oudkerk et al. 2002). This was especially seen in the subgroup of lesions smaller than 1 cm in diameter. This limitation is presumably due to the limited contrast resolution of CT which is not compensated by the excellent geometric spatial resolution.
Benign solid liver lesions, such as Focal Nodular Hyperplasia (FNH) or liver cell adenoma can be detected in CT by the characteristic tumor blush to be seen in the late-arterial phase (Grazioli et al. 2001; Carlson et al. 2000). In addition to the contrast agent behavior, morphological features like the central scar recognizable in FNHs or fatty or regressive changes and hemorrhage in adenomas contribute to establish the correct diagnosis (Fig. 3). Further criteria can be delineated with help of MRI; these criteria will be described in Sect. 2.3.
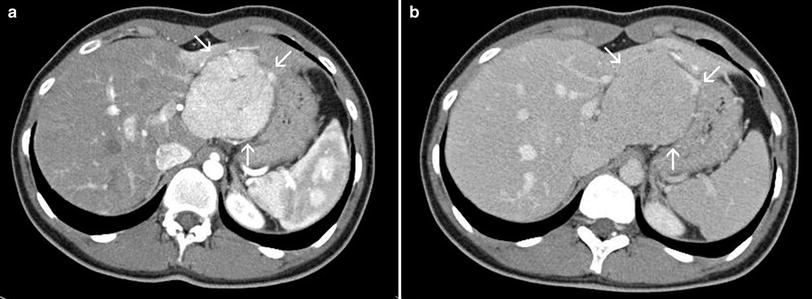
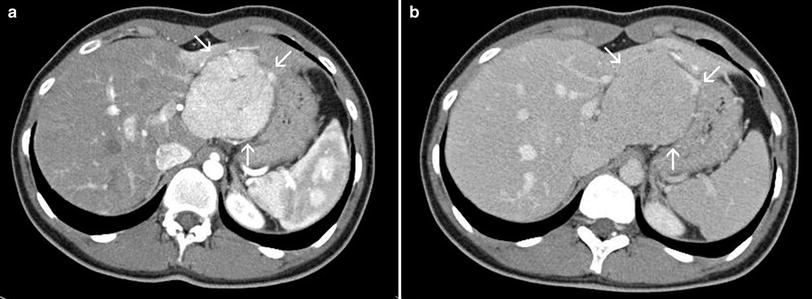
Fig. 3
Focal nodular hyperplasia of the liver in a young female patient in the late-arterial (a) and porto-venous phase (b). Note the strong, homogenous enhancement of the lesion in the arterial phase with a spot of hypodensity in the central parts, representing the central scar. The adjacent liver parenchyma is rather compressed than infiltrated by the lesion. Together with additional features like the wash-out to isointensity and the lobulated, well-defined margin the diagnosis of a FNH can be made with confidence in this case
According to the literature liver metastases are detected with spiral CT with a sensitivity ranging from 58 to 85 % (Lencioni et al. 1998; Ward et al. 1999; Valls et al. 2001). Data from single-row spiral CT and MDCT show that the optimal reconstructed slice thickness for reading CT examinations of the liver on transversal sections is in the range of 2.5–5 mm (Weg et al. 1998; Haider et al. 2002). Since a reconstructed slice thickness of 2.5 mm is difficult to obtain without motion artifacts on single-row scanners, the use of MDCT can be regarded as helpful and advantageous per se. As mentioned above, modern scanners allow for submillimeter collimations, so that slices with <1 mm can be obtained without problems. Our own experience and the data from the literature, however, showed that such thin slices are not superior in the detection of liver metastases. On the contrary, the large number of slices to be reviewed and the strong increase in image noise are rather disadvantageous (Haider et al. 2002; Kawata et al. 2002).
Depending on the primary tumor, liver metastases can present with different morphological and enhancement characteristics, which mainly correspond to the consistency (cystic, mucinous, solid) and the vascularity (hypovascular or hypervascular). Some primary tumors usually have hypervascular liver metastases. These include thyroid carcinoma, carcinoid tumors, neuro-endocrine tumors, and renal cell carcinoma (Danet et al. 2003). Metastases from pancreatic carcinoma, breast carcinoma, and colonic carcinoma may sometimes also be hypervascular (Danet et al. 2003). In liver metastases from a cancer of unknown primary (CUP) hypervascular lesions can be seen occasionally. The hypervascular nature of these lesions can be best appreciated in the late-arterial phase of the liver. The pronounced vascularity leads to a fast wash-out of the contrast agent in later phases, which underlines the need for a proper timing of the late-arterial phase as described above (Fig. 4). Hypovascular metastases appear hypodense in both arterial and portal-venous phases. One should be aware of the fact that this classification reflects only the degree of lesion enhancement compared to the normal liver parenchyma in the arterial and portal-venous phases. It is known that even hypovascular lesions have a considerable amount of vascularization (which is a basic presumption for the Radioembolization treatment of hypovascular metastases) and show contrast uptake, but to a lesser degree compared with the surrounding liver parenchyma (Danet et al. 2003).
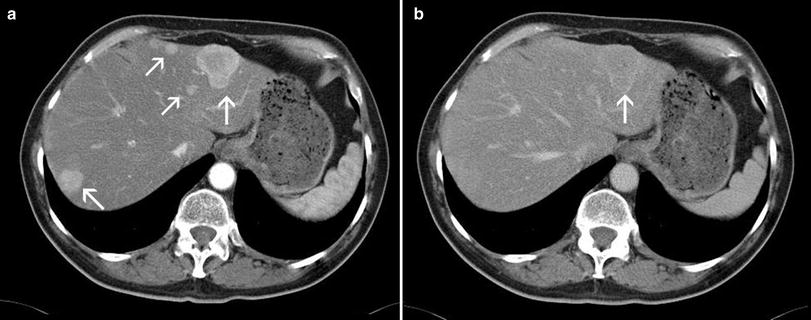
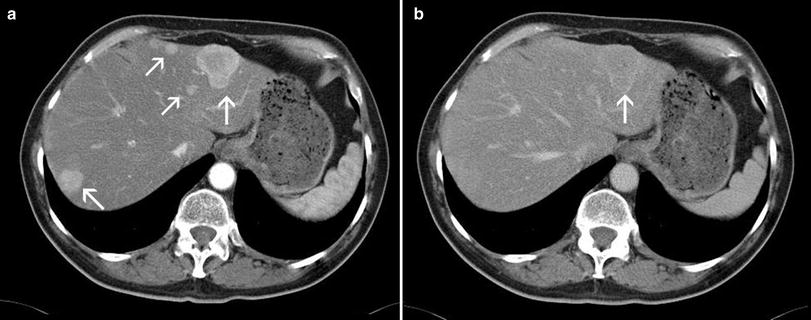
Fig. 4
MDCT in the late-arterial a and porto-venous phase b in a male patient suffering from a neuro-endocrine carcinoma with liver metastases (arrows). Note the strong wash-out of the metastases to nearly isointensity, so that even the larger lesions can retrospectively not be properly detected in the porto-venous phase in contrast to the excellent conspicuity of the lesions in the arterial phase. This example strikingly demonstrates the importance of a correctly timed late-arterial phase
Imaging of the cirrhotic liver is a challenging task for every modality. The detection rates of HCC reported in the literature are highly variable. One trial with a very stringent methodology showed a sensitivity of 61 %, a specificity of 66 %, and a negative predictive value of 30 % for the detection of HCC (Burrel et al. 2003). A subgroup analysis in this trial revealed a strong influence of the lesion size. While lesions >2 cm were detected in 100 %, lesions smaller than 1 cm were only detected in 10 % (Burrel et al. 2003). Another trial with a 4-row MDCT demonstrated an overall sensitivity of 73 % for lesion detection, with also markedly reduced detection rates (33 %) for lesions smaller than 1 cm (Kawata et al. 2002). Since CT can only depict the vascularity of lesions, it is difficult to distinguish between simple regenerative nodules, high-grade dysplastic nodules, and early HCC in the cirrhotic liver (Burrel et al. 2003). The advantages of MRI in this respect are the possibility of tissue characterization based on different contrast weightings of the pulse sequences (T1, T2) and the availability of liver-specific contrast agents (see Sect. 2.3).
In emergency cases, such as acute hemorrhage within the liver (e.g., in a pre-existing tumor or after liver biopsy), acute vascular occlusion, or inflammatory lesions as abscesses, MDCT is the modality of choice, since it is broadly available, allows for very fast scanning and is based on well-established examination techniques. Following surgical and interventional procedures, MDCT allows for reliable diagnosis of complications, such as hematomas, abscesses, or biliomas (Romano et al. 2005) which can be treated percutaneously with CT-guidance, if required. In the follow-up after transarterial chemoembolization (TACE), the accumulation of Lipiodol is readily visualized with CT, even on non-contrast scans without contrast enhancement, contributing to predict the success of the therapy (Guan et al. 2004; Takayasu et al. 2000).
2.3 Magnetic Resonance Imaging
Magnetic resonance imaging (MRI) is used since the early 1990s as a standard procedure in abdominal and liver imaging. Since then various technical developments have taken place, which helped to further increase the diagnostic value of the method and to further improve image quality. Important milestones toward a fast and robust utilization of MRI in abdominal imaging have been the development of gradient-echo (GRE) sequences, single-shot techniques, and phased-array coils. However, only recently MRI has reached a quality, in which respiratory or motion artifacts are negligible and spatial resolution is considered sufficient. Among various other factors the development of 3D sequences, respiratory triggering, and parallel imaging strategies were important milestones toward robust high quality liver imaging with MRI (Zech et al. 2004a). The introduction of high-field systems might allow an additional improvement in spatial resolution and image quality.
The evolution of 3D sequences has been of special value for applications requiring a very high spatial resolution and dedicated post-processing as for example MR angiography. Dynamic studies after bolus injection of contrast agents with T1-weighted (w) 3D gradient-echo sequences are also very useful in liver imaging (Lee et al. 2000). The inherent higher SNR of 3D sequences as compared to 2D sequences allows increasing spatial resolution in 3D sequences without substantial loss in SNR in comparison to an equivalent 2D sequence (Rofsky et al. 1999). Therefore, with this sequence type the whole liver can be covered within an acceptable breath-hold time of 15–20 s with a slice thickness down to 2 mm. In contrast to MDCT image quality of these thin slice examinations is not degraded by increased image noise, which represents a major limitation in MDCT with less than 3 mm reconstructed slice thicknesses (see Sect. 2.2). Artifacts from respiratory motion are an important problem, which results in degradation of image quality in MR examinations of the liver. In T2-w sequences the introduction of T2-w FSE and single-shot sequences in the 1990s have made breath-hold examinations of the liver feasible in more or less acceptable image quality. Respiratory triggering for T2-w sequences have also been explored for the compensation of breathing artifacts (Katayama et al. 2001; Augui et al. 2002; Zech et al. 2004b). According to Katayama et al. one advantage of respiratory triggering is a better T2 contrast with superior signal-to-noise ratio of the liver parenchyma and improved liver-to-lesion contrast in comparison to breath-hold T2-w FSE and single-shot sequences. Our own results (Zech et al. 2004b) also indicate that respiratory triggering is superior over breath-hold strategies, being one cornerstone for minimized artifacts in T2-w sequences (Fig. 5). The balance between spatial resolution, signal-to-noise ratio (SNR), and acquisition time is crucial for liver MRI. Parallel imaging as a universal tool for accelerated acquisition is therefore of high interest for liver imaging. The positive effect of reduced acquisition times with parallel imaging can be utilized both in T1-w and T2-w sequences without a loss in image quality (McKenzie et al. 2004; Zech et al. 2004b). With the use of parallel imaging techniques in single-shot sequences, as for example HASTE or EPI––sequences for diffusion-weighted imaging, the decreased number of phase-encoding steps enables shortened echo trains leading to an improved definition of edges and reduced blurring.
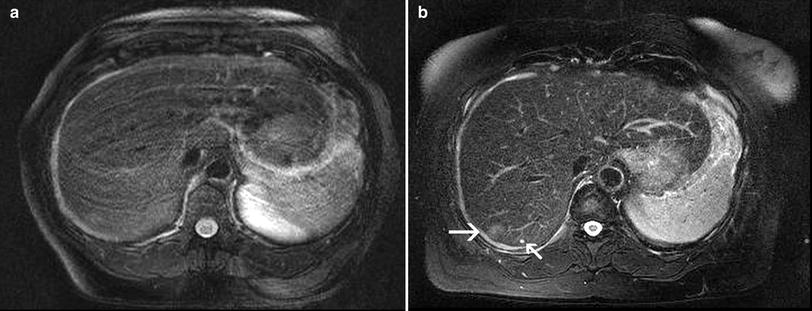
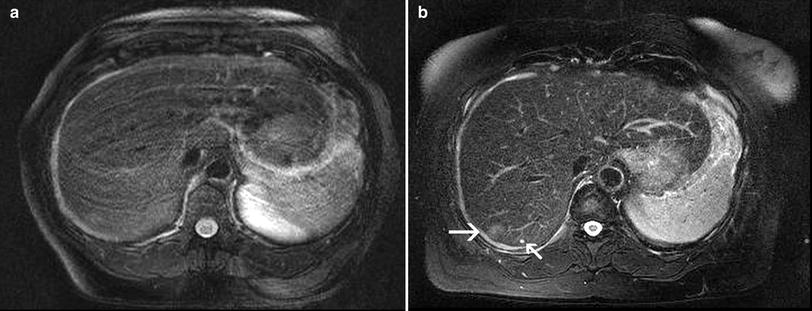
Fig. 5
T2w fast spin echo sequence in breath-hold technique a and with respiratory triggering b in a female patient suffering from breast cancer, in whom liver metastases had to be ruled out. Although the patient has been in a good general state of health, she was not able to hold her breath properly, which resulted in severe breathing artifacts in the breath-hold T2-w fast spin echo sequence with fat saturation. These artifacts also obscure the focal liver lesion in segment 7. In the corresponding slice of the respiratory-triggered T2-w fast spin echo sequence the focal lesion with slight hyperintensity (arrow), suspicious of a metastasis, is clearly depicted. Note also the tiny liver cyst adjacent to the metastasis (small arrow). Both sequences are acquired with parallel imaging (acceleration factor R = 2)
With modern scanner generations and the above mentioned applications, MRI provides a high and quite robust image quality in imaging of the liver. This high image quality is a prerequisite to make use of the inherent advantages of MRI over CT, which are on the one hand the high soft tissue contrast, on the other hand the ability to discriminate different tissue compounds (e.g., fat, mucin, blood, water, but also hepatocytes, bile ducts) based on different signal behavior in T1- and T2-weighted (w) sequences and also based on tissue-specific MR contrast agents.
Extracellular Gadolinium-based contrast agents are still highly important in liver imaging. However, there are also tissue-specific contrast agents available, which allow for an increased detection rate and more specific characterization of focal and diffuse liver diseases. Liver-specific contrast agents can be basically divided into two groups: On the one hand there are iron-oxide particles (SPIO = Superparamagnetic Particles of Iron Oxide), which are targeted to the reticulo-endothelium-system (RES), specifically to the so-called Kupffer cells. These agents cause a signal decrease in T2/T2* weighted sequences by inducing local inhomogeneities of the magnetic field. On the other hand there is the group of hepato-bilary contrast agents, which are targeted directly to the hepatocytes and are excreted via the bile. These agents cause signal increase in T1-weigthed sequences by shortening of the T1 relaxation time.
The basic principle behind SPIO is the fact, that there are usually no Kupffer cells in malignant liver tumors, whereas normal liver parenchyma and solid benign liver lesions contain variable amounts of Kupffer cells (Namkung et al. 2007). Therefore, in the liver-specific phase high contrast is achieved between malignant liver lesions and normal liver parenchyma. Due to the signal loss in normal liver parenchyma the malignant lesions are highlighted as hyperintense lesions in T2*w and T2w sequences compared to the dark liver parenchyma. In most countries SPIO agents are currently not available on the market.
The basic principle of hepato-biliary contrast agents is the specific uptake directly into the hepatocytes. Since all these agents shorten the T1-relaxation times, they cause a signal increase in normal liver parenchyma and in solid benign lesions, whereas malignant lesions like metastases lack specific uptake of hepato-biliary contrast agents. Thus malignant lesions contrast as hypointense lesions against the bright liver parenchyma. In Europe the manganese-based agent mangafodipir trisodium (Teslascan®, Amersham Health, Nydalen, Norway), and the gadolinium-based agents gadobenate-dimeglumine (MultiHance®, Bracco, Milano, Italy) and gadoxetic-acid (Primovist®, Bayer, Berlin, Germany) are approved. Mangafodipir has the drawback that it has to be administered as a short infusion and not as a bolus; therefore, dynamic studies are not possible with mangafodipir. However, the liver specificity is high and the high uptake in normal liver parenchyma enables imaging of e.g., metastases with high contrast to the surrounding liver parenchyma. Gadobenate-dimeglumine and gadoxetic-acid are allowed to be injected as a bolus. With both of these contrast agents early dynamic examinations can be performed, allowing to differentiate lesions with regard to their vascularity into hyper- or hypovascular lesions (Petersein et al. 2000; Huppertz et al. 2005). Due to the lower liver specificity of gadobenate-dimeglumine the imaging time-point of the liver-specific phase starts about 40 min after injection, whereas gadoxetic acid allows for imaging 20 min after injection, with implications mainly on the work-flow of the MR department.
Following injection of unspecific extracellular gadolinium-based contrast agents, solid benign liver tumors (e.g., FNH, adenoma) exhibit a blush-like hypervascularization with fast wash-out to isointensity in MRI which parallels the pattern in contrast-enhanced CT. While central scars are characteristic for FNH, fatty changes, or hemorrhage are typical findings in adenoma. Due to the superior soft tissue contrast of MRI, these morphological details are more often detected with MRI than with CT. Enhancement characteristics with administration of liver-specific contrast agents also greatly contribute to specific characterization of these entities. With hepatobiliary contrast agents such as gadobenate-dimeglumine (MultiHance®) and gadoxetic acid (Primovist®), hypervascularity as well as the presence of hepatocytes in FNH can be assessed (Grazioli et al. 2012; Huppertz et al. 2005; Zech et al. 2008a). Adenoma, on the other hand, usually does not show uptake of hepato-biliary contrast agents and, therefore, can be differentiated from FNH (Grazioli et al. 2012). The challenge in adenomas is nowadays rather the differentiation between adenoma on the one hand and hypervascular metastases or HCC-lesions in non-cirrhotic liver on the other hand. Moreover, by now it is known that adenoma is not a uniform entity and that based on immunohistopathology subtypes can be differentiated which may have a different risk profile (Grazioli et al. 2012).
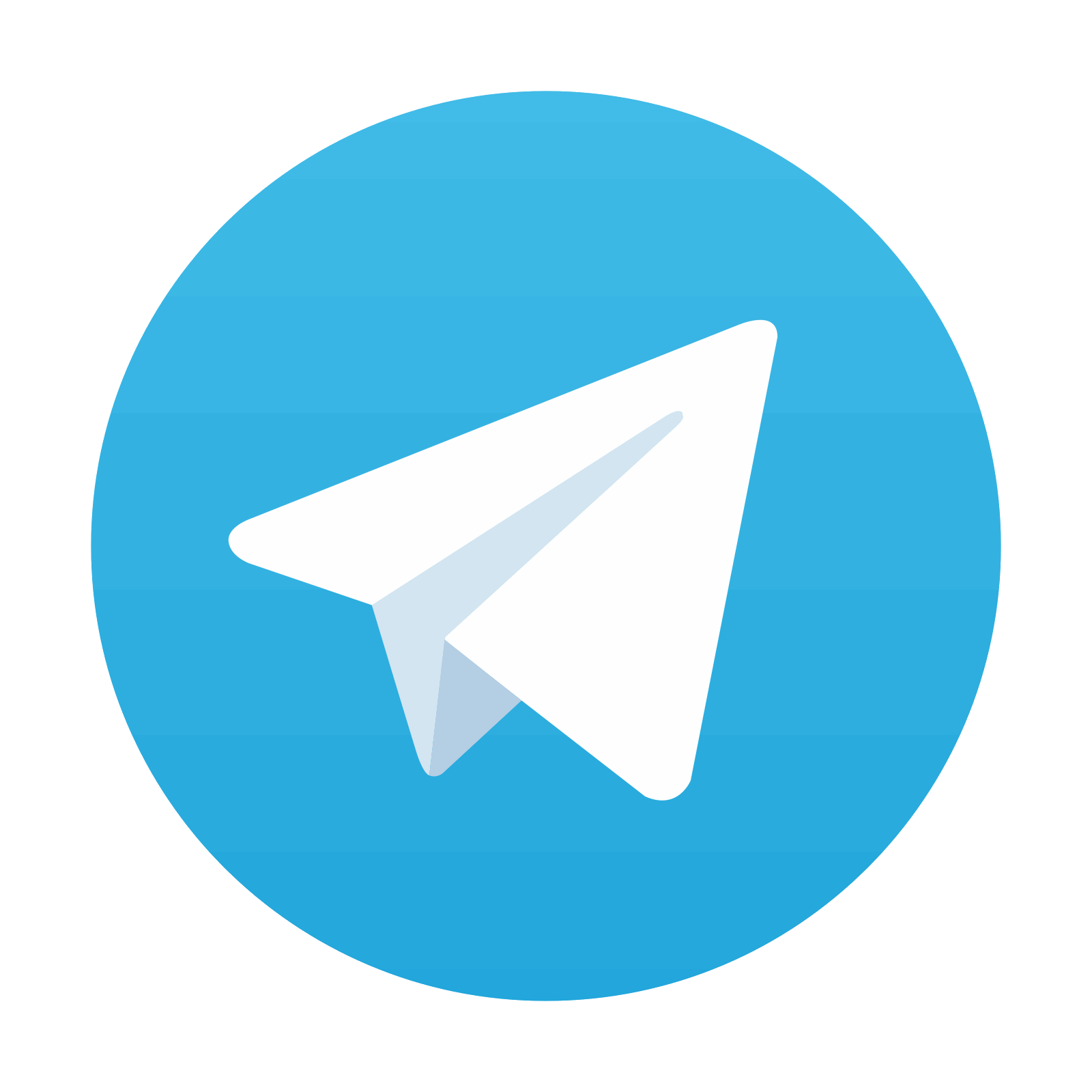
Stay updated, free articles. Join our Telegram channel

Full access? Get Clinical Tree
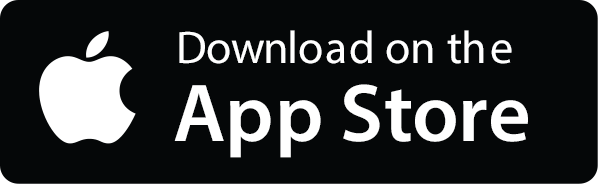
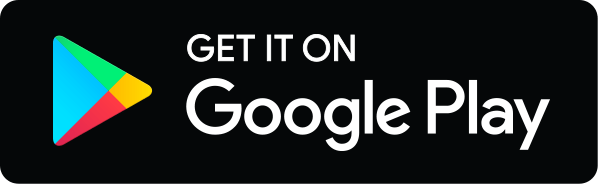