Fig. 1
A 58-year-old female patient with no prior cardiac history. Single-energy CCTA reconstructed in a basal two-chambers short-axis, and b basal four-chambers transverse axial plane. The images demonstrate transmural hypo-attenuation in the inferobasal left ventricular myocardial segment (arrow) consistent with beam-hardening artifact in this region in the absence of significant coronary artery disease. Normal attenuation is identified in the remaining of the myocardium
In a recent study evaluating asymptomatic patients without history of or CT findings for obstructive CAD, beam-hardening related differences between the inferobasal myocardial segment and the reminder of the myocardium were identified in 72 % of patients, mimicking a perfusion defect (Rodríguez-Granillo et al. 2010a, b).
4 Technical Background of Dual-Energy technique in Myocardial perfusion
Dual-energy CT has been recently introduced into clinical practice offering the potential to help reduce these artifacts and their impact on image quality and diagnostic accuracy of CTP imaging. Currently, dual-energy CT has been commercially introduced with two separate mechanisms. One method implements a dual-source system, in which two X-ray tube-detector pairs are mounted onto the same rotating gantry at an angular offset of 90° (or 94° for the 2nd generation scanner), with one tube operating at a peak kilovoltage (kVp) of 80 or 100 kVp, and the other operating at 140 kVp (So et al. 2011; Karcaaltincaba and Aykut 2010).
A second approach has been more recently introduced, which generates single-source dual-energy X-rays based on ultrafast, submillisecond kVp switching (Ko et al. 2012; Silva et al. 2011). The fundamental basis of rapid kVp switching is the acquisition of two different tube voltages alternating on a view-by-view basis with a minimal delay between low (e.g., 80 kVp) and high (e.g., 140 kVp) projections during a single x-ray gantry rotation. This offers the potential for precise temporal registration of views, thereby minimizing the misalignment error from cardiac motion. This technique also relies on new detector and scintillator materials that have ultrafast decay times, enabling the reduction of image noise between projection views. The reduction in noise allows both high- and low-energy data sets to be acquired simultaneously for axial and helical acquisitions at the full 50 cm field of view (Chandra 2011; Wu et al. 2009).
5 Material Decomposition
In diagnostic imaging, X-ray photons interact with different tissues by two distinct mechanisms: the photoelectric effect and Compton scatter. The total attenuation of photons through a specific material is dependent on the contributions of these two effects. Depending on the tissue attenuation coefficient and the energy of the photons delivered, the relative contribution of these interactions to image generation will vary. Compton scatter predominates as the photon energy increases. On the other hand, the photoelectric interaction predominates when lower-energy photons interact with large atomic number materials such as iodine based IV contrast material. Thus, CT numbers (i.e., attenuation values, HU) will vary dramatically with high atomic number materials, whereas with soft tissues, blood and collagen, the CT numbers tend to be less variable and therefore display similar behavior at different photon energies. Recognizing different tissue atomic numbers, attenuation coefficients, and their behavior with variable photon energies, material differentiation can be achieved through spectral monochromatic photon energy (Johnson et al. 2007; Ko et al. 2012).
Owing to the tightly synchronized acquisition, dual-energy single-source/detector CT offers the potential of projection-based monochromatic imaging. To do this, the alternating 80 and 140 kVp raw data (i.e., projection views) are transformed into density (or amount) of the two selected basis materials: water and iodine (So et al. 2011), which can then be reconstructed into corresponding water and iodine images. These two materials are selected owing to their abundance in the typical imaged field of view and due to the significant differences in the way they interact with variable X-ray energy levels. From these material density images, projection-based monochromatic energy images can be generated from their linear combination (Matsumoto et al. 2011).
This process of converting the measured attenuations into density projections, reconstructing the density images, and further processing to obtain a monochromatic attenuation coefficient at any desired photon energy is known as “material decomposition”, which offers the potential for material quantification and characterization and may allow for dynamic quantified myocardial perfusion Fig. 2a, b (So et al. 2012).
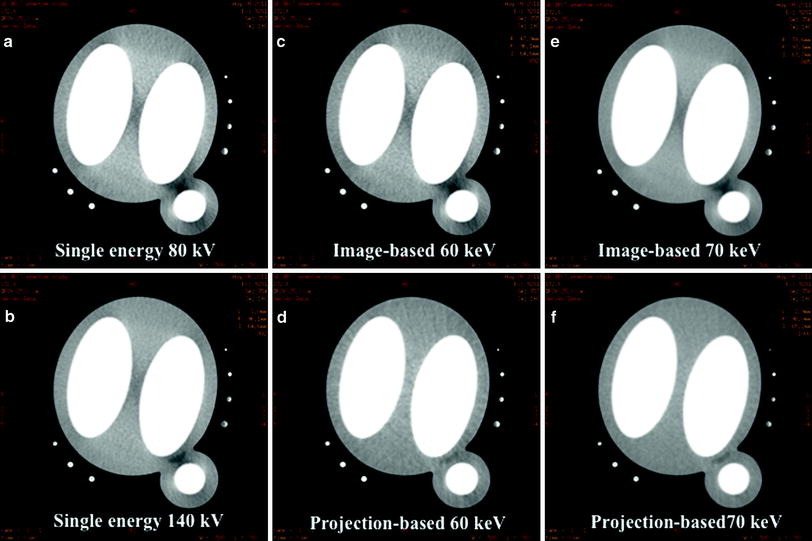
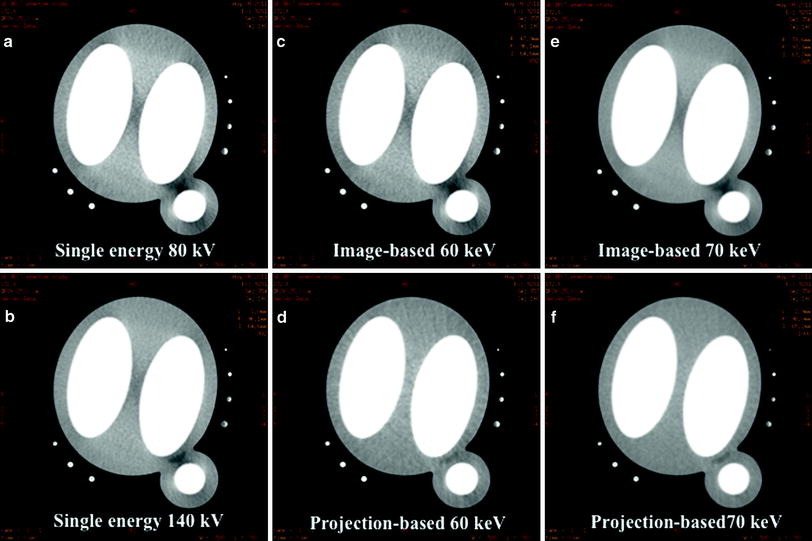
Fig. 2
Transverse axial images of a myocardial phantom consisting of two inner chambers filled with contrast representing the ventricular chambers and a contrast-filled cylinder for the aorta. a, b Beam-hardening artifact became evident within the myocardial area when the images of the phantom were obtained with 80 kVp single-energy CT and 140 kVp single-energy CT. c, e The same artifact was constant with image-based reconstructing mono energies at 60 and 70 keV. d, f However, the hypo-attenuation area became less evident at projection-based reconstructing mono energies of 60 and 70 keV, which show homogenous enhancement of the myocardial phantom
Importantly, these monochromatic images are reconstructed from raw projection data, which is unique to the single-source dual-energy platform. In addition, the projection-based technique applied in single-source dual-energy CT requires the 80 kVp and 140 kVp projection sets to be obtained from the same angle, which is only possible for single-source dual-energy systems (So et al. 2012).
Essentially, each monochromatic dataset is a representation of how the imaged object would appear if the X-ray source produced only monochromatic X-ray photons.
6 Iodine Mapping and Quantitative Measurement of Myocardial perfusion with Rapid kVp Switching Dual-Energy CT
Initial experiments in both phantom and animal models have shown great promise for the reduction of beam-hardening artifacts utilizing single-source rapid-kVp-switching dual-energy scanning. Additionally, there is the capacity for material density imaging from dual-energy data to quantify iodine as a surrogate for myocardial blood, facilitating quantification of myocardial perfusion. Ting Lee and colleagues recently used a myocardial phantom consisting of two inner chambers filled with contrast, representing the ventricular chambers, and a contrast-filled cylinder for the aorta to evaluate the efficacy of single-source dual-energy scanning to reduce beam-hardening artifacts Fig. 3 (So et al. 2011). Using the phantom, a region of simulated ischemic defect was placed in the basal lateral wall of the left ventricle. Both chambers and the aortic cylinder were placed in water-filled or diluted contrast-filled rings. The images were acquired with two single-energy techniques at 80 and 140 kVp using the dual-energy scan protocol with rapid kVp switching, allowing projection-based reconstruction monochromatic energy imaging.
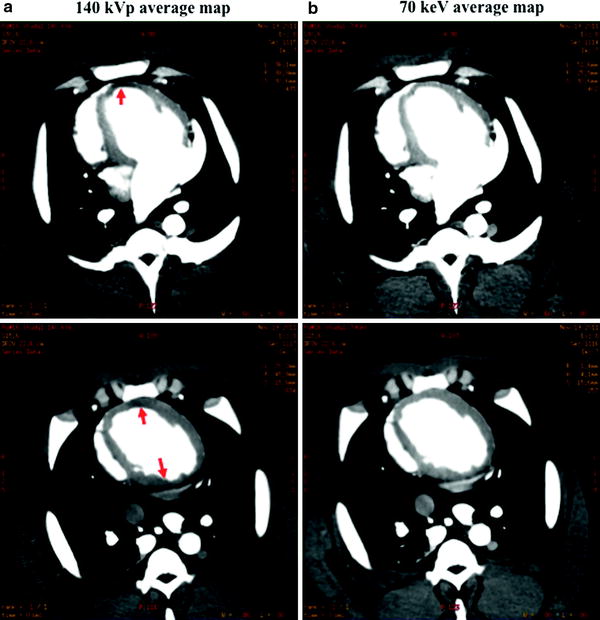
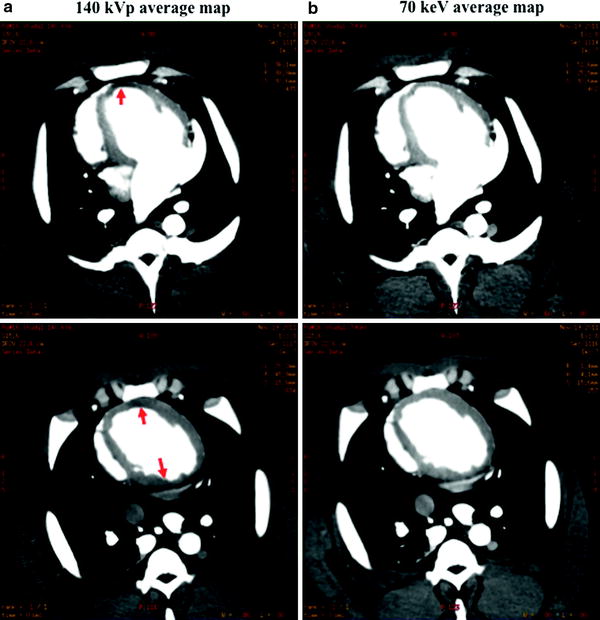
Fig. 3
Myocardial perfusion map in a nonatherosclerotic swine a using 140 kVp single-energy CT and b perfusion map of projection-based single-source dual-energy 70 keV. Note the significant reduction in beam hardening (arrows) at 70 keV as compared to the other reconstructions
Projection-based dual-energy CT showed significantly greater uniformity of the mean attenuation throughout the myocardium suggesting a significant reduction of beam-hardening. Subsequently, this same group performed similar experiments using a Gammex tissue characterization phantom then followed this experiment to determine optimal imaging parameters for iodine sensitivity detection or contrast-to-noise ratio. Rather than using a single iodine concentration, this phantom model consisted of multiple iodine-filled chambers of variable concentrations of iodine solutions. Using the same imaging techniques that were applied to the myocardial phantom, projection-based monochromatic energy imaging (i.e., 70 kilo electron volt ‘keV’) showed the highest sensitivity for iodine detection and also achieved the highest contrast-to-noise ratio compared to imaged-based single-energy or dual-energy techniques (So et al. 2011).
Building on this, additional studies have been performed with a porcine model, in which normal (non-ischemic) pigs were scanned and evaluated with rapid kVp switching dual-energy CT with the use of a Discovery CT750 HD scanner (GE Health care, Waukasha, WI). Two scans were performed, using 140 and 80 kVp alternating at 0.2-ms intervals, 630 mA, and 0.5-s gantry rotation period initiated at 3–4 s after IV contrast injection Fig. 4. The projection-based dual-energy 70 keV images and the corresponding 140 kVp single-energy imaging sets were then analyzed using CTP software. Myocardial average attenuation maps and corresponding aortic and myocardial time-density curve perfusion maps and values were derived and calculated across the maximal cross section of the left ventricular lateral, apical, and septal walls, as well as the aorta. Beam-hardening artifacts were greatly reduced, with the projection-based dual-energy 70 keV images resulting in uniform enhancement throughout the myocardium; this allowed more robust and accurate time-attenuation curve derivation and ultimately accurate quantification of myocardial perfusion defects.