Although ultrasound remains the screening modality of choice in evaluation of the fetal nervous system, magnetic resonance imaging with its multiplanar imaging ability and high signal-to-noise ratio is highly accurate in illustrating the morphologic changes of the developing brain and fetal brain abnormalities. Fetal magnetic resonance imaging is an established powerful tool for obtaining additional information in evaluation of anomalies of the fetal face, neck, and spine. It is helpful to patients and their health care professionals in making vital management decisions and aids in genetic counseling for future pregnancies.
Fetal neuroimaging
The primary imaging method for routine examination of the fetal nervous system is ultrasonography (US). Magnetic resonance (MR) imaging with its multiplanar imaging ability and high signal-to-noise ratio is highly accurate in illustrating the morphologic changes of the developing brain and fetal brain abnormalities. With the development of fast imaging and excellent anatomic detail provided by MR imaging, fetal MR imaging has become a valuable tool in prenatal evaluation. Furthermore, MR imaging can provide excellent anatomic resolution images even when US is limited by patient habitus, fetal presentation, or oligohydramnios. Evaluation of the fetal brain by MR imaging is not limited by the calvarium. MR imaging allows superior delineation of the cortex, ventricles, the subarachnoid spaces, and posterior fossa structures. This article of fetal neuroimaging is primarily focused on evaluation of fetal brain, spine, face, and neck using MR imaging.
Safety of Fetal MR Imaging
Since the initial use of fetal MR imaging in the mid-1980s, there has been no consistent or convincing evidence to suggest harmful effects from short-term exposure of the fetus to changing electromagnetic fields that occur during MR imaging. Experimental studies have not shown any deleterious side effects of MR imaging on the developing embryo or in the long-term outcome of children who had undergone fetal MR imaging between 21 weeks of gestation and term. MR imaging is now accepted as a safe and valuable imaging tool in prenatal evaluation of the fetus.
Even though it is considered safe, MR imaging is not performed in the first trimester of organogenesis. Fetal MR imaging is generally performed in the second trimester of gestation, usually from 18 weeks onwards. It is difficult to obtain good-quality images in very young fetuses by MR imaging. There is Food and Drug Administration approval for fetal MR imaging using magnets up to field strength of 1.5 Tesla.
Fetal Neuroimaging MR Techniques
Different types of coils can be used. A body coil alone or a body phased-array coil in combination with a surface coil positioned on the mother’s abdomen is generally used. Considering the developing brain, the imaging parameters have to be optimized. T2-weighted (T2W) images, in particular, are used to assess the changes in brain tissue water content in the developing brain. Images are routinely obtained in orthogonal planes (ie, sagittal, coronal, and axial planes) relative to the fetal head. Fast T2W images provide the majority of the diagnostic information. The half-Fourier single-shot turbo spin-echo (HASTE) sequence provides heavily T2W images with low susceptibility weighting in a very short time. Sequential slice capability and interleaving allow for good quality images even with physiologic fetal movement. The utility of T1-weighted (T1W) images is limited in the high water content of the developing nonmyelinated brain. T1W imaging is performed in addition to routine T2W imaging in fetuses older than 28 weeks of gestation, when satisfactory T1W signal-to-noise and contrast resolution can be achieved. Gradient-echo images especially the fast low-angle shot (FLASH) sequences allow for excellent differentiation between the cortical ribbon and white matter and the ventricular walls. Extremely rapid gradient-echo, echo planar imaging (EPI), with its high sensitivity to paramagnetic susceptibility, is used in identification of hemorrhage and mineralization as well as osseous and vascular structures. Diffusion-weighted imaging and apparent diffusion coefficient maps can also be obtained and are valuable in assessment of ischemic damage.
At the authors’ institution, fetal cine imaging is routinely included along with fetal morphologic imaging. Fetal cine imaging is useful in assessing dynamic movements, such as swallowing and fetal extremity movements. The entire duration of a typical fetal neuroimaging examination is approximately 30 to 40 minutes. No maternal or fetal sedation is used at the authors’ institution. Contrast agents are not used in evaluation of pregnant patients for fetal MR imaging, because gadolinium crosses the placenta. The toxicity of gadolinium to the fetus is not known.
Indications for Fetal Neuroimaging
MR imaging is not used as a screening tool in evaluation of the fetus. MR imaging is usually performed because of abnormal findings on US evaluation. The most common indications for fetal neuroimaging are listed in Box 1 in order of frequency with the most common indication, ventriculomegaly, listed first.
Ventriculomegaly
Suspected malformation
Potential destructive lesion
Multiple malformations in the fetus
Malformation in siblings/known family history of genetic disease
Maternal infection
A special note should be made of the indications when a fetal MR evaluation is obtained even when brain US study is normal. These include possibility of potentially destructive brain lesions related to maternal coagulation disorders, maternal hypoxia, maternal trauma, and fetal demise in multiple gestation. In all these indications, the role of MR imaging is to assess for brain damage, which is difficult to be evaluated by US. Other indications include maternal infection or a fetus presenting with extracerebral multiple malformations, because of the high incidence of extracerebral lesions associated with brain lesions. Known central nervous system (CNS) malformations or chromosomal aberrations in siblings and family history of genetic disorders involving the CNS also warrant MR evaluation, even with a preceding normal US examination.
MR imaging is usually not indicated in cases of intrauterine growth restriction. However, MR imaging can provide useful information when intrauterine growth restriction is associated with progressive microcephaly or other abnormalities, such as fetal hydrops or arthrogryposis.
Development of the Fetal Brain on MR Imaging
MR imaging appearance of the developing fetal brain reflects changes of histogenesis and myelination. Subsequent changes are seen in the brain volume, surface configuration (ie, sulcation), and internal configuration ( Figs. 1–3 ).
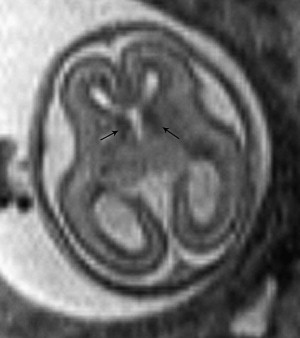
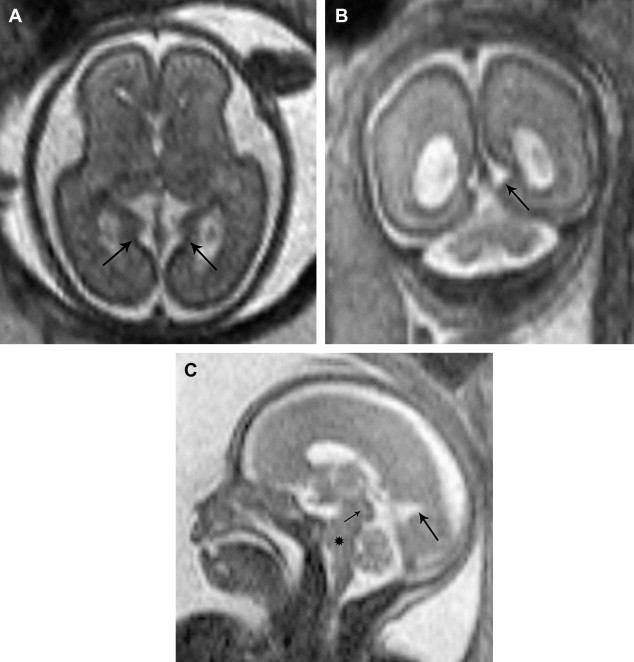
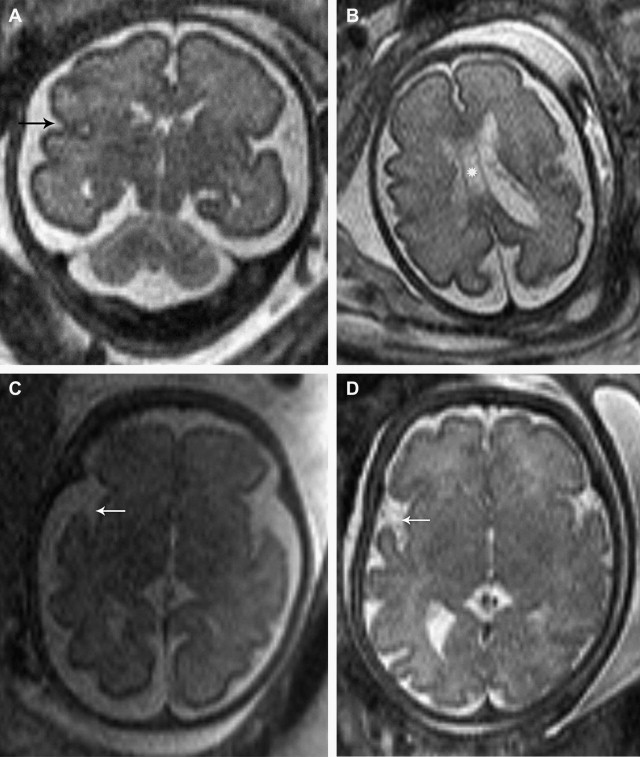
Table 1 describes cortical landmarks demonstrated in almost 75% of developing fetal brains with respect to gestational ages.
Sulci/Fissures | Appears | Deepens | Developed |
---|---|---|---|
Parieto-occipital | 16 to 17 wk | 18 to 20 wk | 20 to 22 wk |
Calcerine | 16 to 17 wk | 18 to 20 wk | 24 wk |
Central sulcus | 24 to 25 wk | 31 wk | 35 wk |
Precentral sulcus | 26 to 27 wk | 31 wk | 35 wk |
Postcentral sulcus | 28 to 29 wk | 31 wk | 35 wk |
There is ongoing research to develop MR-based findings for assessing brain maturation and development. One study has developed a semiquantitative scale and scoring system for assessing brain maturation. Defined visual imaging indices, including specific areas of myelination, presence, and location of germinal matrix, cortical infolding, and sulcal depth, have been used.
Anomalies of the fetal brain
Ventriculomegaly
Ventriculomegaly is one of the most common indications for performing fetal brain MR imaging. Ventriculomegaly may be due to an apparent cause, which can be detected by imaging in approximately 60% of cases. In approximately 40% of cases no apparent cause can be detected on imaging.
Ventriculomegaly is defined as ventricular size, measured at the atrial level, greater than 10 mm. Ventriculomegaly can be divided into mild (10 to 15 mm) ( Fig. 4 ), moderate (>15 mm with >3 mm of adjacent cortical thickness), and severe ventriculomegaly (with <2 mm of adjacent cortical thickness) ( Fig. 5 ). The causes of ventriculomegaly may be several. If it is mild, it could be transient and possibly a normal finding. Ventriculomegaly may be related to cerebral dysgenesis example corpus callosal anomalies. It could be the result of an ex vacuo phenomenon secondary to atrophy, infections, or infarction. It may also be related to hydrocephalus associated with disorder of cerebrospinal fluid (CSF) dynamics.
The prognosis of ventriculomegaly depends on the cause of the ventricular dilatation, the gestational age at which occurs, and its progression. Mild isolated ventriculomegaly (10 to 12 mm) is not associated with adverse postnatal outcomes in majority of the cases. Developmental delay is associated with mild isolated ventriculomegaly, however, in the range of 19% to 36%. Ventriculomegaly with dysmorphic appearance is usually a feature of neural tube defects. A box-shaped configuration of the frontal horns is often associated with absence of septum pellucidum. Colpocephaly, which is disproportionate dilatation of the atria and occipital horns with small frontal horns, is often present with hypogenesis of the corpus callosum. Enlarged ventricles, with effacement of the extracerebral CSF spaces along with large head size, usually denote an obstructive component to the ventriculomegaly. When there is preservation of extracerebral CSF spaces or prominence with ventriculomegaly, however, hypogenesis is usually the cause. In some cases, hypogenesis and hydrocephalus can coexist.
Corpus Callosum Abnormalities
Agenesis of the corpus callosum could be complete or partial (hypogenesis). This could result either from a vascular or inflammatory insult occurring in the early (before 12 weeks) gestation. In agenesis of the corpus callosum, the interhemispheric axonal fibers do not cross the midline. As a result the fibers are distributed longitudinally as Probst bundles and located along the superomedial margin of the lateral ventricles. Colpocephaly is present with a high-riding third ventricle. Associated findings include ventriculomegaly, parallel orientation of the frontal horns, absence of the septum pellucidum, and small head size. The cingulate sulcus is absent in complete agenesis of corpus callosum ( Fig. 6 ).
Corpus callosum agenesis is often associated with other malformations, chromosomal abnormalities, and genetic syndromes. Corpus callosal agenesis may be present along with Dandy-Walker malformation as well as other neural tube defects, such as Chiari II malformation. Interhemispheric clefts and cysts with hypogenesis of the corpus callosum have also been documented ( Fig. 7 ).
Partial hypogenesis of corpus callosum could result from from incomplete formation or from a postformational destructive process. When there is massive ventriculomegaly, it may be difficult to visualize the corpus callosum. Fetal MR imaging in corpus callosal agenesis can delineate other findings, such as pericallosal lipoma, interhemispheric cysts (see Fig. 7 ), and gyral abnormalities as well as heterotopias. Retrospective studies have demonstrated that 43% of cases of confirmed corpus callosal agenesis were detected by fetal MR but not by US.
Disorders of Dorsal Neural Tube Development
Disorders of dorsal neural tube development associated with defects of primary neurulation include anencephaly, cephalocele, myelomeningocele, and the Chiari II malformation.
Cephaloceles
Cephalocele is protrusion of intracranial contents via a bony defect of the skull. Locations of the cephaloceles, in order of occurrence, are occipital, frontal, parietal, and basal. The protrusion may include only meninges and CSF or, in addition, varying portions of brain parenchyma ( Fig. 8 ). Fetal MR imaging is valuable in characterizing the contents of the cephaloceles and detecting any underlying or associated brain abnormalities.
Chiari II malformation
Typical stigmata of Chiari II malformation include small posterior fossa and downward herniation of the hindbrain through the foramen magnum into the upper cervical spinal canal. This is best depicted on midline sagittal MR images ( Fig. 9 ). Additional findings include varying degrees of ventriculomegaly and dysmorphic appearance of ventricles. There may be concurrent corpus callosal abnormalities. Associated findings of gray matter heterotopias ( Fig. 10 ) may be present and can be detected on MR imaging.
Myelomeningocele (MMC) is associated with Chiari II malformation. Fetal MR imaging is important in selecting and triaging patients with MMC and Chiari II malformation, who may be potential candidates for in utero surgical repair.
Spinal findings of neural tube defects on fetal MR imaging are discussed separately in the spine section of the article.
Disorders of Ventral Neural Tube Development
Disorders of ventral neural tube development occur due to insults to the rostral end of the embryo (which contribute in formation of the face and the brain during 5 to 10 weeks of gestational age). Abnormalities of frontal induction include the holoprosencephalies, septo-optic dysplasia, cerebral and cerebellar hypoplasia, and the Dandy-Walker spectrum.
Holoprosencephaly
In the alobar form, the interhemispheric fissure and the falx are absent. There is a large monoventricle and the thalami are fused ( Fig. 11 ). There is microcephaly and abnormal frontal sloping with protuberant orbits. Facial anomalies are usually associated with the alobar holoprosencephaly, such as cyclopia, hypotelorism, anophthalmia, proptosis, and cleft lip and palate. In the semilobar form, there is partial separation of the cerebral hemispheres posteriorly, but there remains a single ventricular cavity and varying degrees of fusion of the basal ganglia and thalami. Lobar holoprosencephaly is the least severe form in which there is near complete separation of the hemispheres but there is fusion at the level of the cingulate gyrus and frontal horns of the lateral ventricles ( Fig. 12 ). The septum pellucidum is usually absent. It may be difficult to distinguish between lobar holoprosencephaly and septo-optic dysplasia. Fetal MR imaging can distinguish holoprosencephaly with large monoventricle from agenesis of the corpus callosum with associated large midline clefts or cysts. MR imaging is particularly useful in demonstrating subtle findings of lobar holoprosencephaly, which are likely to be missed by US. Fusion of the frontal lobes and continuity of the cortex across the midline are depicted on the MR imaging in lobar holoprosencephaly.
Dandy-Walker Spectrum
Although US is adequate in demonstrating severe forms of the Dandy-Walker malformation, MR imaging is superior in delineation of dural structures, position of the tentorium, and other associated abnormalities. The Dandy-Walker malformation is characterized by large posterior fossa cyst in continuity with the fourth ventricle along with vermian agenesis or hypogenesis and elevation of the torcula ( Fig. 13 ). Hydrocephalus is usually present. The cerebellar hemispheres are usually hypoplastic and compressed laterally by the large posterior fossa cyst.
Posterior fossa cystic malformations also includes the Dandy-Walker variant, mega cisterna magna, Blake pouch cyst, and retrocerebellar arachnoid cyst. The midline sagittal view is helpful in characterizing these abnormalities and also in characterizing the cerebellar vermis ( Figs. 14 and 15 ). It may sometimes be difficult to distinguish Dandy-Walker variant from Blake pouch cyst or retrocerebellar arachnoid cyst.
Dandy-Walker malformations are usually associated with other CNS or systemic abnormalities, including chromosomal abnormalities, corpus callosal agenesis, holoprosencephaly, and disorders of cortical migration organization, such as the Walker-Warburg syndrome, which can be seen on MR imaging.
Disorders of Proliferation, Differentiation and Histogenesis
Disorders of neural, glial, and mesenchymal proliferation can be divided into those arising in the premigration stage, the migration, and the postmigration stage. These disorders are not commonly evident on US; hence, MR imaging is most appropriate technique for detection of such malformations. This information from MR imaging is useful for genetic counseling for future pregnancies because the majority of these malformations have a genetic basis of origin.
Neuronal migrational disorders
Neuronal migrational disorders occur during neuronal migration characterized by the disturbance of normal neuronal arrangement. This group of abnormalities consists of lissencephaly, including type I (agyria and pachygyria) and type II (Walker-Warburg syndrome) ( Fig. 16 ); polymicrogyria; gray matter heterotopias (nodular and laminar heterotopia); and microcephaly.
Lissencephaly type I is characterized by thickening of the cortical ribbon with broad flat gyri and primitive shallow sulcation ( Fig. 17 ). This may be associated with corpus callosal hypogenesis. The type II lissencephaly (for example, cobblestone lissencephaly) has thickened and severely disorganized cortex. Meninges are thickened and densely adherent to the cortex and obliterate the subarachnoid space. There may be associated subcortical heterotopias. Other associated abnormalities include callosal hypogenesis, micropthalamia, and cerebellar vermian hypoplasia. Muscular dystrophy, congenital eye malformations, and posterior cephaloceles are some other known associations. Fetal MR imaging can characterize and detect all these findings in these cases.
Posterior fossa histogenetic disorders include cerebellar hypogenesis, cerebellar cortical dysplasias, and heterotopias. These abnormalities result from nonformation of one or both cerebellar hemispheres. In hypoplasia the cerebellar hemispheres may be formed but are small. In some cases there is vermian hypogenesis with or without cerebellar hypogenesis. Many cases of fetal ventriculomegaly are usually associated with cerebellar hypogenesis.
Postmigratory Organization Disorders
Polymicrogyria
The most common entity in this category is the polymicrogyria, which is characterized by multiple small and irregular sulci associated with enlarged overlying subarachnoid spaces ( Fig. 18 ). Ventricular dilatation may be present. Polymicrogyria due to an infectious cause, such as cytomegalovirus (CMV) infection, can be suspected when calcifications may be evident.
Schizencephaly
Schizencephaly, although included in the postmigratory group of abnormalities, is mostly considered to be of destructive origin resulting from insult developing before 16 weeks of gestation. Schizencephaly is characterized by a CSF-filled, gray matter–lined cleft that extends from the cortex to the wall of the lateral ventricle ( Fig. 19 ). Associated malformations include ventriculomegaly, polymicrogyria, heterotopias, corpus callosal agenesis, and absence of the cavum septum pellucidum.
Neuronal heterotopias
Heterotopias represent gray matter in abnormal locations as result of abnormality in the stage of migration. Several causes are postulated, including vascular and environmental causes, such as trisomy 13, fetal alcohol syndrome, and maternal infections. Most patients with subependymal heterotopias have seizures (see Fig. 10 ). Those patients with laminar or band heterotopias may have developmental delay and earlier onset of seizures. It may be necessary in young fetuses to repeat the MR imaging examination in few weeks after the initial examination to better appreciate the findings of polymicrogyria.
Clastic Abnormalities of the Developing Fetal Brain
Clastic lesions include hemorrhage, infarction, porencephaly, encephalomalacia, leukomalacia, atrophy, and hydranencephaly.
Hemorrhage
Hemorrhage intracranially could occur in the extra-axial space, intraventricular or within the brain parenchyma. Although the timetable of evolution of fetal hemorrhage is not precisely established, the blood products can be seen as high signal intensity on T1W images and low signal intensity on T2W images. If the hemorrhage is remote, then presence of blood products could be identified by secondary findings of focal ventriculomegaly, porencephalic cyst, cavities, or atrophy. The EPI gradient sequences can identify remote blood products as focal increased susceptibility from hemosiderin staining ( Fig. 20 ). There are many reports that show MR imaging identifying hemorrhage, which is not seen on US. Intraventricular hemorrhage could be identified as irregular T2 hypointensity of the choroid plexus itself or in ventricles besides the choroid plexus. The cause of hemorrhage may not be evident by imaging alone; however, information about underlying causes, such as vascular malformations or mass lesions, can be demonstrated on MR imaging. MR imaging is also more sensitive in identifying sequelae of hemorrhage, such as porencephaly and encephalomalacia. Isolated small germinal matrix hemorrhage may be visualized on MR imaging, which may not be seen by US. The clinical significance of isolated fetal germinal matrix hemorrhage, however, is not known.
Ischemia/infarction
Fetal MR imaging is the preferred modality to demonstrate fetal ischemia or infarction. This may not be apparent by US. Diffusion-weighted imaging can further aid in demonstration of acute to subacute ischemic lesions. The classic ischemia of arterial distribution is not the common pattern seen in utero. Ischemia in the fetal brain may involve the cortical ribbon and white matter or just the white matter alone. Hemorrhagic infarction is more common. Ischemic cerebral changes in utero may result in white matter necrosis or leukomalacia. On fetal MR imaging, particular attention should be paid to the morphology of the germinal matrix to identify presence of any focal areas of T2 hypointensity or increased susceptibility on gradient images. Sometimes this may be evident only as a focal irregular dilatation of the ventricle. In contrast, larger areas of ischemia and the sequelae with diffuse necrosis are easy to identify on MR imaging ( Fig. 21 ).
Hydranencephaly
Hydranencephaly is considered to be a sequel of ischemic event in the distribution of internal carotid arteries, which has occurred after the basic brain structure was formed. Large portions of the involved cerebral hemispheres are replaced with fluid-filled cavities ( Fig. 22 ). When these areas of involvement are large, it can be confusing to differentiate hydranencephaly from alobar holoprosencephaly. The differentiating feature, however, is presence of an intact falx, which is noted in hydranencephaly that distinguishes it from alobar holoprosencephaly (see Figs. 11 and 22 ). Another helpful feature to identify hydranencephaly is foci of susceptibility from hemorrhage or blood products, which may be seen in most of the cases.
Porencephaly and encephalomalacia
These are sequelae that may result from hemorrhage, ischemia, infection, or trauma. Fetal MR imaging is helpful in identifying these lesions and in several cases may provide clues regarding their origin. Information obtained from fetal MR imaging is important in estimating the extent of brain injury and predicting outcome.
Infections
CMV infection is the most common congenital CNS infection. Fetal MR imaging reflects the neuropathologic changes of microcephaly, parenchymal destruction, periventricular necrosis, ventricular dilation, cysts, and disorganized cortex and heterotopias ( Fig. 23 ). Periventricular calcifications are not readily detected on MR but may be evident on gradient-echo EPI sequences.
Aqueductal Stenosis
Aqueductal stenosis may be sporadic or hereditary, as seen with X-linked hydrocephalus. There is characteristic enlargement of the lateral ventricles and the third ventricle with a normal-sized fourth ventricle ( Fig. 24 ). Aqueductal stenosis may be associated with other abnormalities, such as corpus callosal agenesis, and Dandy-Walker malformation. It may result as a sequela of prior hemorrhage or infection.
Neurocutaneous Disorders
The most common neurocutaneous disorder identified in utero is tuberous sclerosis. Tuberous sclerosis is an autosomal dominant multisystem disorder characterized by the triad of epilepsy, mental retardation, and characteristic adenoma sebaceum. This disorder comprises multiple angiomyolipomas, cardiac rhabdomyomas, and intracranial stigmata as well. The intracranial lesions consist of cortical tubers, subependymal nodules, and mixed cell astrocytomas at the foramen of Monro. Cortical tubers as well as subependymal nodules appear as focal T2 hypointensities ( Fig. 25 ). When calcified, they may demonstrate focal increased susceptibility. The intracranial stigmata are better identified with MR imaging, especially in the younger fetus.
In Utero Fetal CNS Mass Lesions
Congenital brain tumors are rare. The most common tumors in this category are teratomas followed by glioblastomas ( Fig. 26 ). Fetal MR imaging is helpful in characterizing the location, extent, morphology, and its effect on surrounding structures.
