14 Intraoperative magnetic resonance imaging (iMRI) was developed to allow neurosurgeons near-real time visual feedback during surgery. Applications of this technology have included image-guided biopsy, drainage of cysts, instillation of therapeutic agents, thermal ablations, laminectomies,1,2 and the most obvious application, the resection of brain tumors. Prognosis after the resection of high grade glial tumors is in part dependent upon the extent of resection;3,4 therefore maximal resection is likely worth the extra time and cost of intraoperative imaging.5,6 The relationship between prognosis and the extent of resection is somewhat more controversial in low grade glial tumors7,8 but is still likely to be directly related. Furthermore, the control of seizures, which are often present with low-grade tumors, can be related to extent of resection.9 The margins of low-grade tumors are often less distinct than those of high grade tumors, and adjacent structures may therefore be at greater risk for injury if resection is performed without some form of image guidance. iMRI can provide the surgeon with direct evidence of whether or not there is a radiographically complete resection prior to leaving the operating room (Figs. 14.1 and 14.2). Radiographic evidence that a gross total resection has been accomplished not only provides assurance of maximal resection, but also allows the surgeon to avoid unnecessary further resection of normal cortex adjacent to a tumor. In many cases, the limiting factor in an aggressive surgical resection of tumors is the presence of nearby functional cortex. For obvious reasons, accomplishing a maximal resection with minimal disruption of normal brain parenchyma is particularly relevant to the surgical treatment (biopsy or resection) of these tumors located near eloquent parts of the brain. Multiple strategies have been employed to assist the surgeon in the resection of tumors near eloquent cortex. Traditional neuronavigation techniques have allowed surgeons to operate with smaller, more accurate surgical corridors, whereas techniques such as direct cortical stimulation, other forms of neuromonitoring and performance of tumor resection while the patient is awake allow for some degree of real time or near-real time feedback regarding the integrity of surrounding eloquent areas. Frameless neuronavigation provides the neurosurgeon with precise data at the beginning of a procedure and thus is an excellent tool for planning a surgical corridor; however, as the surgery progresses, there may be a shift of normal brain tissue into the surgical bed as tumor is resected, or away from the surgical site if there is significant egress of cerebrospinal fluid (CSF) out of the cranium.10,11 Removal of the calvarium at the time of craniotomy combined with the loss of CSF when the dura mater is opened can result in significant amounts of brain shift within the cranium (Fig. 14.3). The result of this shifting of the brain is that the images that are used in determining completeness of resection (and the safety of further resection) may be inaccurate leading either to unexpected residual tumor or surgical incursion into functional areas of normal brain, depending upon the direction of the shift. Attempts that have been described to quantify and compensate for brain shift have utilized ultrasound or vascular markers.12 Cortical stimulation and other neuromonitoring techniques and awake neurosurgery allow for some degree of near-real time feedback regarding the integrity of functional areas during surgery. These techniques, however, require limitations to anesthesia, do not provide the neurosurgeon with image guidance, and often do not provide meaningful feedback until eloquent cortex has been breached. Furthermore, these techniques, while useful in preserving eloquent areas of brain, offer no information concerning the completeness of resection. Fig. 14.1 Preoperative 1.5 tesla axial turbo fluid-attenuation inversion recovery magnetic resonance imaging (FLAIR MRI) scan of a left frontal low-grade glioma before surgical manipulation of the brain or the occurrence of brain-shift. The brain activation scan for this patient is shown in Fig. 14.4 and demonstrates that the cortical location of finger tapping function was posterior to the tumor. (From Hall W, Kim P, Truwit C, Functional Magnetic Resonance Imaging-Guided Brain Tumor Resection. Philadelphia: Lippincott Williams and Wilkins; 2008. Reprinted with permission.) Fig. 14.2 Intraoperative 1.5 tesla axial turbo fluid-attenuation inversion recovery magnetic resonance imaging (FLAIR MRI) scan of the patient displayed in Fig. 14.1 that demonstrates that the entire preoperative tumor imprint has been resected and the goal of surgery has been achieved. (From Hall W, Kim P, Truwit C, Functional Magnetic Resonance Imaging-Guided Brain Tumor Resection. Philadelphia: Lippincott Williams and Wilkins; 2008. Reprinted with permission.) Fig. 14.3 Intraoperative turbo fluid-attenuation inversion recovery magnetic resonance imaging (FLAIR MRI) scan that was obtained at 1.5 tesla showing a complete radiographic resection of a right frontal low-grade glioma. The surgical cavity is filled with air and pneumocephalus is present over both frontal lobes. Brain-shift has resulted from the egress of cerebrospinal fluid during the surgical procedure. (From Ulmer S, Jansen O. FMRI – Basics and Clinical Applications. New York: Springer Science + Business Media; 2009. Reprinted with permission.) Functional magnetic resonance imaging (fMRI) is a technique in which patients undergo MRI while performing particular tasks to map precisely those areas of the brain that are being activated. Functional MRI is a noninvasive but also indirect method of mapping eloquent cortex. The mechanism that allows for the generation of fMRI is complex and depends upon the delivery, volume, and fractional oxygenation of blood.13 As discrete cortical areas are activated during each specific task, metabolic activity increases and oxygen is therefore extracted from hemoglobin molecules to an extent that is increased over baseline. The loss of the oxygen results in a focal increase in intracellular deoxyhemoglobin, with its unpaired iron electrons within the hemoglobin molecule, thus altering the local magnetic field, and a signal change relative to adjacent tissues on suitably tailored MR images. Cortical activation also results in an increase in local blood flow which compensates for this effect. Because of this combination of antagonistic effects, the degree of activation is not measured in fMRI. Unlike data from neurophysiologic techniques, fMRI data can be directly applied to preoperative imaging studies, allowing for determination of the surgical goals prior to taking the patient to the operating suite. The surgeon is additionally provided with a visual representation of the position eloquent cortex and its relationship to the tumor, which aids in both the preoperative and intraoperative surgical decision making (Fig. 14.4). fMRI has previously been used in conjunction with neuronavigation, and functional images can be coregistered with those that are used for neuronavigation. Because of the limitations caused by brain shift, as described above, fMRI used in this way was not fully protective against iatrogenic neurologic deficit. To provide more accurate delineation of functional areas during surgery, functional MRI has been combined with intraoperative scanning. In this chapter, the technique of intraoperative MRI (iMRI) with fMRI is described. Fig. 14.4 Axial turbo fluid-attenuation inversion recovery magnetic resonance imaging (FLAIR MRI) scan demonstrating a left frontal low-grade glioma. This brain activation study was performed at 3.0 tesla and the task being performed was finger tapping of the right hand. (From Ulmer S, Jansen O. FMRI – Basics and Clinical Applications. New York: Springer Science + Business Media; 2009. Reprinted with permission.) Fig. 14.5 Axial T1-weighted brain activation study performed at 3.0 tesla showing the area for finger tapping of the left hand. The tumor that is planned to be resected surgically is just anterior and medial to the cortical area for brain activation. The posterior aspect of the tumor is nicely delineated by a medial and lateral sulcus. (From Ulmer S, Jansen O. FMRI – Basics and Clinical Applications. Philadelphia: Lippincott Williams and Wilkins; 2008. Reprinted with permission.) Functional imaging as a surgical modality allows for the delineation of eloquent brain areas prior to surgery (Fig. 14.5). The identification of the location of brain function in turn allows surgery to be performed without particular consideration to the type of anesthesia used and without the need to spend additional time defining functional areas during surgery. Furthermore, the tasks performed during fMRI acquisition may be more complex than those tasks performed during awake craniotomy. Our paradigm for combining functional imaging with iMRI has been the preoperative acquisition of fMRI combined with surgical resection or biopsy using iMRI at high field strength (1.5 tesla). As with all imaging modalities, functional MRI and iMRI do not replace meticulous surgical planning and execution. The surgeon utilizing the algorithm described above must mentally extrapolate the relevant blood oxygen level-dependent (BOLD) fMRI to iMRI scans. Critical to the success of iMRI is the neurosurgeon’s ability to determine when intraoperative scanning should take place, based upon his or her recognition that a critical juncture in the procedure has been reached. Furthermore, he or she must be cognizant of underlying white matter tracts, which may be encroached upon while a surgeon is operating far from areas of brain activation. Diffusion tensor imaging (DTI) is a relatively new MRI modality that allows for the mapping of the location of white matter tracts and may be useful in combination with fMRI when it is felt that there is a high risk of undermining white matter tracts during the removal of a particular tumor. Ideal candidates for fMRI-guided brain tumor resection are those patients who harbor tumors that are located adjacent to eloquent cortex, who are able to cooperate with the tasks associated with brain activation, and who have minimal or no neurologic deficits caused by the tumor. Patients with significant preoperative neurologic deficits due to infiltration of tumor tissue into functional brain areas are not optimal candidates for fMRI-guided resection. Similarly, patients who are severely claustrophobic and thus require strong sedation for MRI are not candidates for fMRI-guided neurosurgery. Although seizure activity affects BOLD imaging, a history of seizures is not a contraindication to fMRI. Low-grade and high-grade glial tumors, as well as metastatic lesions, have all been resected using our paradigm of iMRI with fMRI. Of the original 346 procedures performed at the University of Minnesota using iMRI, 103 were craniotomies with resection of brain tumors. Of these cases, 14 (14%) were deemed to be close enough to eloquent cortex to necessitate preoperative fMRI. Additionally, we have used fMRI preoperatively to determine whether a patient is not a candidate for image-guided resection, choosing iMRI-guided brain biopsy instead (Fig. 14.6). Fig. 14.6 Axial fluid-attenuation inversion recovery magnetic resonance imaging (FLAIR MRI) brain activation scan for left finger tapping at 3.0 tesla demonstrating the activation is immediately lateral to a presumed low-grade glioma. Based on the results of the brain activation study, the patient underwent an MRI-guided brain biopsy, which disclosed an astrocytoma. Because of the infiltrative nature of this tumor type, the patient was subsequently treated with adjuvant radiation therapy. (From Hall W, Kim P, Truwit C, Functional Magnetic Resonance Imaging-Guided Brain Tumor Resection. Philadelphia: Lippincott Williams and Wilkins; 2008. Reprinted with permission.)
Functional Magnetic Resonance Imaging-Guided Brain Tumor Resections
Functional MRI
Patient Selection
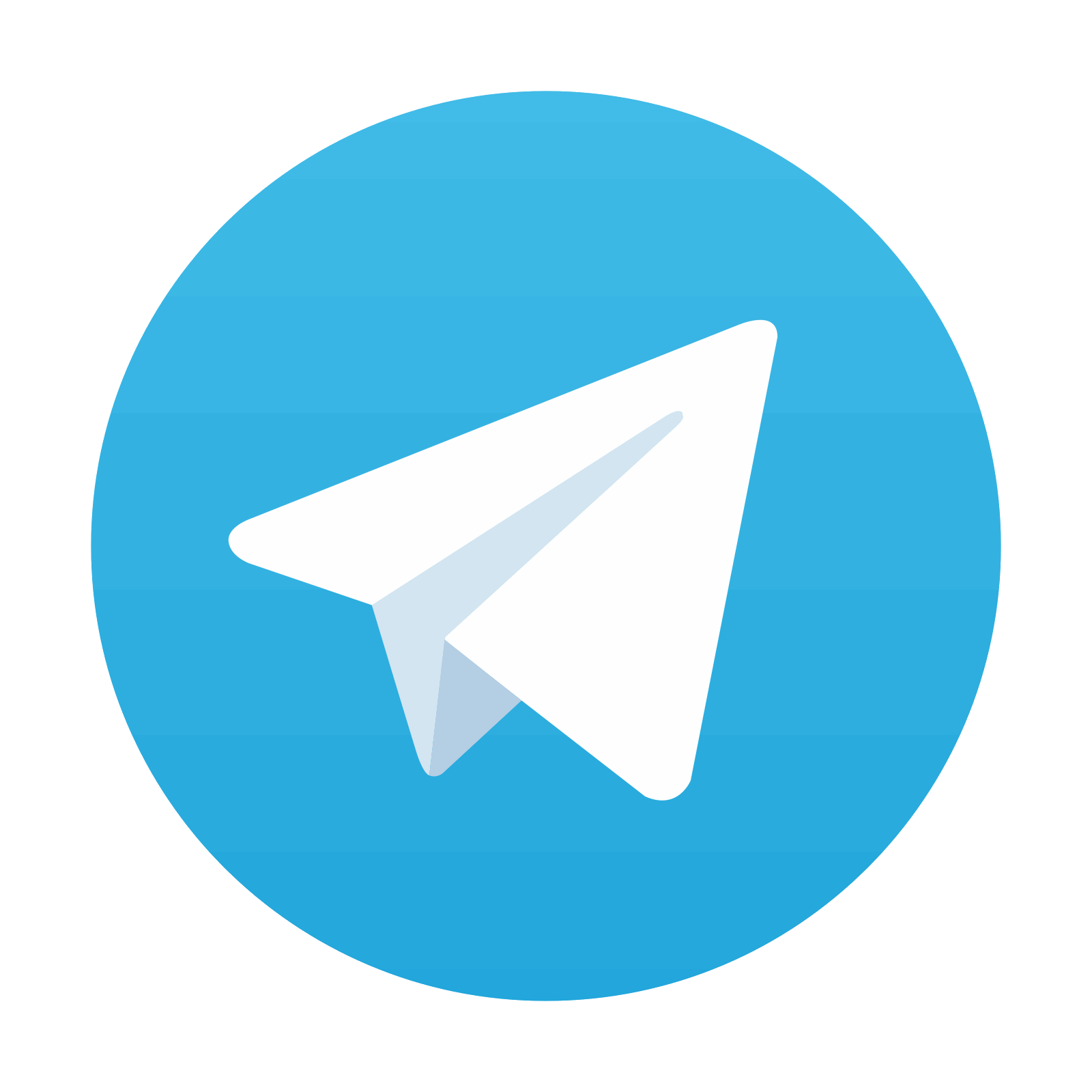
Stay updated, free articles. Join our Telegram channel

Full access? Get Clinical Tree
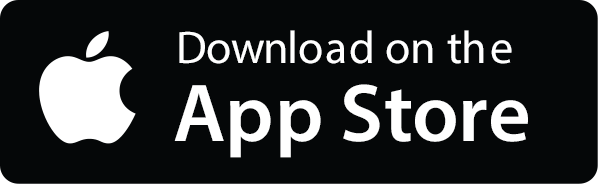
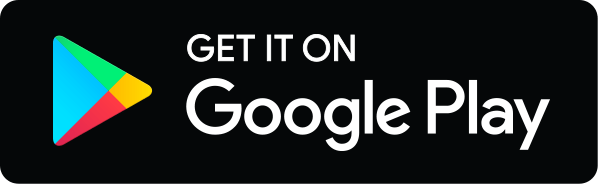