Introduction
Proton beam radiation (PBR) has the potential to improve the therapeutic ratio in the treatment of several gastrointestinal malignancies by decreasing the dose to nontarget critical structures. In general, radiotherapy to the abdomen and pelvis is challenging because of the inherent sensitivity of gastrointestinal organs to radiation toxicity.
Gastric
The role of radiation therapy
Postoperative radiotherapy for gastric cancer was once the established standard of care on the basis of the Intergroup 0116 study showing the survival benefit of adjuvant fluorouracil-based chemoradiation (CRT) compared with surgery alone. When CRT is administered, the prescribed dose is 45 to 50.4 Gray (Gy) in 1.8-Gy fractions, with higher doses sometimes considered for positive margins or gross residual disease. However, patients treated at most centers in Western countries are more likely to receive perioperative chemotherapy, which has also been shown to improve survival over surgery alone. In Eastern countries, the practice is to routinely perform more extensive surgery, including D2 lymph node dissection, and chemotherapy is the preferred adjuvant treatment. Patients with node-positive disease and intestinal-type histology did seem to benefit from adjuvant CRT when compared with adjuvant chemotherapy, and this subset of patients is the subject of an ongoing trial.
Completion rates for adjuvant CRT and chemotherapy are historically poor, with 15% and 10% of patients not able to complete adjuvant CRT and chemotherapy, respectively, as a result of toxicity. , For this reason, a neoadjuvant therapy approach is currently favored at the University of Texas MD Anderson Cancer Center. In a cooperative group phase II trial, the pathologic complete response (pCR) rate and margin-negative resection (R0) rates were 26% and 77%, respectively, and 98% of patients were able to complete all therapy per protocol.
The rationale for proton therapy
Other efforts to decrease acute toxicity and improve the tolerability of CRT for gastric cancer have centered on the use of more conformal radiation techniques to minimize the normal tissue irradiated. Current US guidelines state that intensity-modulated radiation therapy (IMRT) may be used to reduce dose to organs at risk. Studies have shown IMRT to be feasible and well tolerated in both the neoadjuvant and adjuvant settings. , However, IMRT leads to a larger volume of normal tissue receiving low radiation doses, which raises the concern for increased secondary malignancy rates. PBR has the potential to improve nontarget tissue sparing and further improve the tolerability and therapeutic ratio for gastric cancer. Furthermore, PBR has the potential to reduce the secondary malignancy rates that have been reported in higher numbers after adjuvant CRT when compared with surgery alone on long-term follow-up.
Dosimetric studies
In the postoperative setting, PBR has been shown to reduce the volume of several normal organs receiving low to moderate doses. One treatment planning study showed the median volume of small bowel receiving 15 Gy (V15) was 133 cc with IMRT and 82 cc with 2- to 3-field double-scattered-uniform scanning technique proton therapy. Mean liver and kidney doses were also lower with PBR compared with IMRT. Perhaps more importantly, a significantly lower mean heart dose was achieved with PBR compared with IMRT (7.4 Gy relative biologic effectiveness [RBE], assuming an RBE of 1.1 for protons compared with photons, vs. 9.5 Gy). With data from long-term breast cancer survivors suggesting that the rates of major coronary events increase linearly with mean heart dose at a rate of 7.4% per Gy with no apparent threshold, this suggests a meaningful reduction in late toxicity may be afforded by PBR in this population. Dionisi et al. also showed the robustness of PBR in the postoperative treatment of gastric cancer by reporting that target coverage on repeat verification computed tomography scans was within ±2% of the initial simulation scan. This is important because one of the challenges of using PBR in the treatment of gastric cancer is the variability of gastric volume, contents, and the presence of gas. Appropriate volumes to account for uncertainties in day-to-day target volumes, as well as dietary management, such as following a low-residue diet and fasting for a defined period before treatment, are essential for the accurate and effective treatment of gastric cancer.
Clinical studies
The potential for both feasibility and clinically meaningful toxicity in the preoperative or inoperable settings can be extrapolated from work done in esophageal and gastroesophageal junction cancers. pCR rates and near-pCR rates were high with few severe toxicities in a prospective study performed at MD Anderson evaluating proton-based preoperative CRT to a dose of 50.4 Gy equivalents (GyE) for esophageal cancer. A retrospective study of definitive proton-based CRT to 60 GyE performed at the University of Tsukuba likewise showed good control rates and very low rates of serious acute toxicities. Two case reports in the 1990s demonstrate the feasibility of using definitive PBR for inoperable advanced gastric cancer , and showed clinical CRs after 61 GyE in 35 fractions and 83 to 86 GyE, respectively. The potential dosimetric and clinical benefits of PBR for gastric cancer certainly merit further study. The specific location, size, and extent of tumor will likely influence the magnitude of benefit ( Fig. 10.1 ).
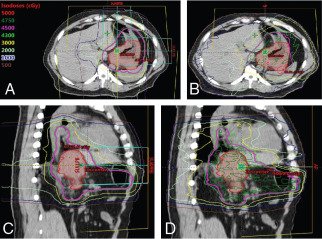
Pancreas
The role of radiation therapy
Pancreatic adenocarcinoma is a particularly aggressive disease with surgical resection as the only current curative option, but median overall survival (OS) time after surgery alone is only 20 months. Systemic therapy has been shown to improve disease-free survival, but the use of radiotherapy in general for pancreatic malignancies is somewhat controversial. , A currently open randomized trial is evaluating whether concurrent fluoropyrimidine-based CRT improves survival for patients who have received 5 months of adjuvant chemotherapy after surgical resection of pancreatic adenocarcinoma. Because completion rates of adjuvant therapy are low, interest is growing in a neoadjuvant approach. , This neoadjuvant treatment approach is currently favored at MD Anderson. Neoadjuvant CRT can help convert borderline-resectable cancer to potentially operative disease, improve the margin-negative (R0) resection rates, , and potentially reduce costs associated with care. Stereotactic body radiotherapy (SBRT) has emerged as an alternative to standard fractionated radiotherapy. A currently accruing trial by the Alliance for Clinical Trials in Oncology (A021501) is comparing neoadjuvant therapy consisting of either chemotherapy alone or chemotherapy followed by either SBRT (33–40 Gy in 5 fractions) or hypofractionated image-guided radiation (25 Gy in 5 fractions). Finally, despite the lack of an OS benefit demonstrated in the randomized trial evaluating consolidative CRT given after 4 months of induction chemotherapy, CRT did result in improved local control (LC) with no increased rates of grade 3 to 4 toxicity except for nausea. Studies have shown that local progression is the leading cause of death for patients with locally advanced pancreatic cancer after 15 months. As systemic therapy improves distant control, local treatment modalities, such as CRT, may have the chance to prove their value.
The rationale for proton therapy
The pancreas is located in an anatomically challenging part of the body surrounded by radiosensitive organs, such as the stomach, duodenum, jejunum, kidneys, and liver. Radiation to the pancreas can cause acute side effects, such as fatigue, nausea, vomiting, diarrhea, and abdominal cramping. Radiation is only one part of a multidisciplinary approach, so it is important to minimize the side effects of this component to improve the completion rates for all therapy. PBR may be able to improve the therapeutic ratio by both allowing dose escalation while minimizing toxicities from unintended dose to adjacent normal organs.
Dosimetric studies
One early dosimetric study showed the potential benefit of PBR for the treatment of inoperable pancreatic cancer necessitating large fields. Zurlo et al. showed that for four patients for whom IMRT plans conferred a 5% higher risk of toxicity to the kidneys, liver, or bowel, proton plans were able to deliver the same target coverage without the excessive risk of morbidity. Other treatment planning studies have also shown the benefit of PBR for reducing low to moderate doses to normal tissue. One small study showed the feasibility of PBR to safely dose escalate to 59.4 GyE while reducing mean dose to the spinal cord, left kidney, right kidney, and liver by 78%, 73%, 42%, and 55%, respectively. Another treatment planning study performed at MD Anderson showed that PBR could allow safe dose escalation to 72 GyE with reduction in the V15 of the stomach and small bowel (48% vs. 5% and 61% vs. 9%, respectively). In the postoperative setting, treatment planning studies have also shown the ability to reduce dose to the small bowel, stomach, and kidneys with PBR. , However, there are some concerns regarding the robustness of PBR plans for the treatment of pancreatic cancer. One study showed that proton plans were highly susceptible to interfractional anatomic change, with coverage of the clinical target volume reduced by 8% as a result of the daily variability. A worst-case optimization strategy, which has been studied in carbon ion therapy for pancreatic cancer, may be useful to mitigate risks posed by interfractional anatomic changes. Fig. 10.2 shows IMRT and IMPT comparison plans for a patient receiving neoadjuvant SBRT for borderline-resectable pancreatic cancer.
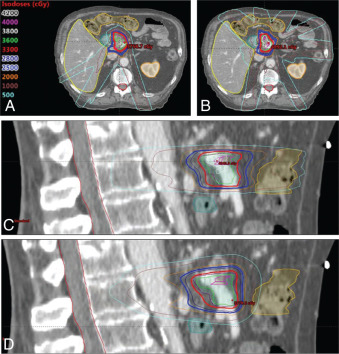
Clinical studies
Results from a phase I study at Massachusetts General Hospital showed no dose-limiting toxicities at any of the four dose levels of PBR tested in patients with localized, resectable adenocarcinoma of the head of the pancreas. Radiation regimens ranged from 3 Gy(RBE) × 10 fractions (dose level 1) to 5 Gy(RBE) × 5 fractions over 1 week (dose level 4), and 5 Gy(RBE) × 5 fractions was established as the maximum tolerated dose (MTD). Concurrent capecitabine was given, and surgical resection was performed 4 to 6 weeks after completion of radiotherapy. Of the 15 patients enrolled, 4 had G3 toxicities (pain, stent obstruction, and infection), and 11 went on to undergo surgical resection. There were no unexpected postoperative complications. The same group performed a subsequent phase II study on which 35 additional patients were enrolled and treated with the established MTD of 5 Gy(RBE) × 5 fractions. The treatment was well tolerated with an acute G3 toxicity rate of 4.1%. The median progression-free survival time was 10 months, and the median OS time was 17 months. Locoregional failure occurred in 16.2% of patients, and distant failure was the predominant pattern of failure at 72.9%. These LC and OS results are slightly better than those reported in photon-based SBRT series (median survival time of 14.5 months and 1-year LC rate of 61%), but this may be related to patient selection, and randomized clinical studies are still needed to compare the two modalities.
Primary liver
The role of radiation therapy
Transplant and surgical resection are considered to be the only curative options for patients with hepatocellular carcinoma (HCC), but a minority of patients are anatomically and medically eligible for a curative surgical approach at diagnosis. The National Comprehensive Cancer Network guidelines list ablation, arterially directed therapies, and external beam radiation therapy (EBRT) as locoregional therapy options for those patients for whom resection is not feasible or a bridge to transplant is desired. EBRT is recommended with the category of 2B (based on lower-level evidence, with consensus that the intervention is appropriate). However, current patterns of practice data suggest that radiation is an underused modality for these patients. Data from the Surveillance, Epidemiology, and End Results (SEER) program suggest that only 9% of Medicare patients diagnosed with HCC between 1998 and 2007 were even seen by a radiation oncologist. Radiation is also not a first-line treatment in the treatment of intrahepatic cholangiocarcinoma (IHC). The mainstay of curative treatment is surgical resection, with no conclusive data to guide adjuvant therapy recommendations. Chemotherapy consisting of cisplatin and gemcitabine remains the mainstay for metastatic or unresectable disease, but a currently active trial is evaluating the role of radiation therapy for patients in this setting.
The rationale for proton therapy
One barrier to the use of radiotherapy in the treatment of HCC and IHC is concern about radiation-induced liver disease (RILD) and other toxicities to nearby organs, such as the bowel, bile duct, and kidneys. However, more recent data from the current treatment era suggest radiation can be delivered safely by using more conformal techniques and may provide valuable LC benefits that may translate into improved OS survival for patients with unresectable HCC and IHC. , The physical properties of PBR allow a more favorable therapeutic ratio by reducing exit dose through healthy, functional liver tissue. Thus PBR can offer a better therapeutic ratio, particularly for IHC HCC patients who might otherwise have unacceptable hepatic toxicity from photon-based radiation.
Dosimetric studies
The use of PBR for tumors in the liver has been studied more extensively than for cancers in any other gastrointestinal site. Early treatment planning studies confirmed that PBR had the ability to significantly reduce the dose to the normal liver as well as to other organs at risk compared with both three-dimensional (3D)-conformal techniques and IMRT. Dose escalation on the order of 20% to 30% was also possible with PBR while achieving lower doses to nontarget tissues.
Clinical studies
Most of the early data on the use of PBR for HCC has come from Japan, where HCC is endemic. Results from hypofractionated regimens (16–25 fractions) to ablative doses for large tumors are similar to those after surgical resection, with 5-year LC and OS rates of 90% and 50%, respectively. The group at Tsukuba University has published extensively on the use of PBR to safely treat larger tumors to larger doses per fraction. The same group published on a more recent cohort of 318 patients with HCC treated between 2001 and 2007, and the 5-year OS rate was 44.6%. Treatment regimens were more standardized in this cohort, with patients with peripheral tumors away from the gastrointestinal mucosa and porta hepatis receiving 66 Gy(RBE) in 10 fractions, tumors within 2 cm of the porta hepatis receiving 72.6 Gy(RBE) in 22 fractions, and tumors within 2 cm of the gastrointestinal mucosa receiving 77 Gy(RBE) in 35 fractions. These regimens were very well tolerated, with no reported cases of RILD and few serious toxicities. Other case series from Asian centers show similar outcomes, , which have been shown to vary based on patient and tumor characteristics, including liver function, disease burden, and portal vein tumor thrombus (PVTT). Encouraging response rates and acceptable toxicity profiles were seen as well when PBR was used for advanced HCC with PVTT. A more recent report showed feasible and promising results from a risk-adapted simultaneous integrated boost technique using PBR for patients with HCC and PVTT. Median OS time was 34.4 months in this cohort of 41 patients treated with 50 to 66 Gy(RBE) in 10 fractions depending on gross tumor volume and distance from gastrointestinal mucosa.
Several prospective studies have also been conducted and published in this setting. Mizomoto and colleagues reported on 266 patients treated in three prospective protocols developed at the Proton Medical Research Center in Tsukuba, including 66 GyE in 10 fractions for tumors more than 2 cm away from the portal region, 72.6 GyE in 22 fractions for tumors within 2 cm of the hilum, and further reduction to 77 GyE in 35 fractions for tumors adjacent to the gastrointestinal tract. Most of the tumors were less than 5 cm. The average 3-year LC and OS rates were 87% and 61%, respectively, with no significant differences in LC among the three different fractionation schemes used. Toxicity rates were low, suggesting that appropriate selection of dose and fractionation based on tumor location can improve the therapeutic ratio. Long-term outcomes for patients treated at Tsukuba were recently reported and showed favorable long-term control with no grade 3 or higher toxicities. This update also showed 5-year LC and OS rates of 90% and 34%, respectively, for patients with PVTT, suggesting that PBR may be a viable treatment strategy for this subset of patients with historically poor outcomes.
In the United States, a phase II trial from Loma Linda evaluated PBR for 76 patients with inoperable HCC and cirrhosis. Some 18 patients in this cohort eventually underwent liver transplant, and 33% of those explants showed a pCR after 63 Gy(RBE) delivered over a 3-week period. MD Anderson participated in a multi-institutional trial headed by Massachusetts General Hospital, which included patients with unresectable HCC or IHC. PBR was used to deliver up to 67.5 Gy(RBE) in 15 fractions to tumors from 2 to 12 cm (median 5 cm). Most of the included patients had cirrhosis, and 79.5% were Child-Pugh B. Despite the unfavorable characteristics of this cohort, LC rates at 2 years were 94.8% for HCC patients and 94.1% for IHC patients; OS rates at 2 years were 63.2% and 46.5%, respectively.
Two randomized clinical trials involving PBR for HCC are currently underway. At Loma Linda, patients with HCC who met either the Milan or the San Francisco transplant criteria were randomized to receive PBR or transarterial chemoembolization (TACE). In the PBR arm, the dose was 70.2 Gy(RBE) in 15 fractions over 3 weeks. In an interim analysis reported recently, the 2-year OS rate was 59% for the cohort, with no difference between the groups. Approximately one-third of the patients in each arm went on to transplant and the pCR rate after PBR was 25% compared with 10% after TACE, although the difference was not statistically significant. A trend toward was noted improved LC rates in the PBR arm (88% vs. 45% at 2 years; P = .06). There were fewer hospitalization days also after PBR when compared with TACE, which the authors suggest may indicate reduced toxicity. The NRG Oncology cooperative group recently opened a phase III trial randomizing patients with HCC to receive definitive treatment with either photons or protons. With a primary outcome of improved OS, hopefully this trial will generate the necessary data to cement the benefit of PBR for this population of patients.
Metastatic liver
The role of radiation therapy
The definitive management of liver metastases is common practice for treating patients with colorectal primaries, as long-term survival, and even cure, are possible. With surgical resection of liver metastases coupled with the development of more effective chemotherapy regimens, the median survival time for these patients has increased to 20 months, with 20% to 25% of patients still alive 10 years later. However, not all patients are surgical candidates, either because of the location of the disease, medical comorbidities, or the hepatoxicity of prior chemotherapy or other liver-directed therapies. Thus interest has been growing in the use of radiotherapy for the treatment of these patients. SBRT is of particular interest, given the relative radioresistance of colorectal metastases to standard dose/fractionation regimens. ,
The rationale for proton therapy
Most SBRT studies have primarily included patients with small metastatic tumors less than 3 to 6 cm in maximum dimension. The limiting factor for treating larger volumes remains the risk of RILD and other toxicity of nontarget tissues. As is the case with primary liver tumors as described above, PBR has the potential to allow to dose escalation while sparing nontarget liver and other at-risk organs.
Dosimetric studies
Dosimetric benefits of PBR for the treatment of hepatic metastases are largely extrapolated from treatment planning and clinical studies of primary liver tumors. However, given the potential for long-term survival after successful treatment of isolated hepatic metastases, there is additional concern for radiation-induced secondary malignancies that result from unnecessary dose to nontarget tissues. A group from the University of Stockholm performed a treatment planning study that showed using IMPT for liver SBRT significantly decreased the calculated risk of secondary malignancy by decreasing dose to skin, lungs, esophagus, and healthy functional liver.
Clinical studies
A single-arm phase II trial was recently completed in which 89 patients with liver metastases from a variety of primary tumors were treated with PBR-based SBRT to 30 to 50 Gy(RBE) in 5 fractions depending on the effective volume irradiated. No G3–5 toxicities were reported, and the median survival time was 18.1 months. LC rates at 1 and 3 years were 71.9% and 61.2%, respectively. SBRT was found to be successful in this population, even when tumors were larger than 6 cm. At MD Anderson, we have also used PBR to perform a “radiation hepatectomy” for patients with bilobar colorectal liver metastases who were initially deemed candidates for a two-stage hepatectomy but who were not able to undergo the second stage of treatment. For this unique group of patients, ablative doses of PBR (biologically effective dose >89.6 Gy[RBE]) were delivered to the entire right hemiliver. All five patients treated with this regimen experienced a partial or complete radiographic response and had in-field LC at latest follow-up. Two patients remain alive without evidence of disease, and two experienced distant progression outside the liver.
A currently accruing phase I–II trial at Loma Linda is evaluating toxicity and LC rates after proton-based SBRT for liver metastases. The radiation regimen consists of 3 fractions to total doses of 36, 48, or 60 Gy(RBE) in an escalating fashion. The hope is that the dosimetric advantages of PBR in the liver can help to safely escalate dose while maintaining a safe toxicity profile.
Rectal
The role of radiation therapy
For patients with T3, T4, or node-positive rectal adenocarcinoma, the current standard of care in the United States is to administer neoadjuvant CRT to a total dose of 45 to 50.4 Gy in 25 to 28 fractions before total mesorectal surgical excision followed by adjuvant chemotherapy. An alternative strategy is to administer 25 Gy in 5 fractions followed by surgery. Radiation is also sometimes used for recurrent disease, either before reresection or definitively to palliate symptoms of the local recurrence. Hyperfractionation is commonly used for reirradiation, with the regimen commonly used at MD Anderson consisting of 39 Gy in 1.5-Gy fractions delivered twice daily with a 6-hour interfraction interval.
The rationale for proton therapy
Similar to what has been demonstrated for other pelvic malignancies, PBR has the potential to reduce dose to organs at risk while maintaining adequate dose coverage to the intended target.
Dosimetric studies
Several benefits, including bone marrow, bowel, and bladder sparing, have been demonstrated when using PBR for whole-pelvis irradiation for gynecologic and prostate malignancies. , Similarly, Colaco et al. from the University of Florida demonstrated that PBR was able to reduce the volume of bone marrow receiving 5, 10, 15, and 20 Gy(RBE). Also, the volume of small bowel receiving 30 and 40 Gy(RBE) and the volume of bladder receiving 40 Gy(RBE) could also be reduced with PBR when compared with traditional 3D conformal RT plans delivering 45 Gy to the pelvis followed by a boost of 5.4 Gy to the tumor plus margin. Other treatment planning studies have found similar benefits. Some dosimetric analyses have suggested that the decreased volume of small bowel irradiation may make treatment escalation possible, either with increased radiation dose or with an intensified chemotherapy regimen, which may improve pCR rates and increase LC. ,
Clinical studies
For recurrent rectal cancer, some studies have shown the potential benefits of PBR. At the University of Pennsylvania, seven patients with recurrent rectal cancer after prior CRT and surgery were treated with PBR to a mean dose of 61.2 Gy with concurrent fluorouracil. Only one patient experienced a CR, and the toxicity rates were significant, with three cases of G3 diarrhea, one case of G3 abdominal pain, two cases of small bowel obstruction, and one rectovaginal fistula. Data were also presented in abstract outlining the treatment of six patients with pelvic recurrences after prior radiation with a more modest dose of 39 to 45 Gy using PRB with a single posteroanterior beam or opposing lateral fields. No G3+ toxicities were reported with a median follow-up time of only 1 year, and no in-field failures were reported. One trial is currently active and recruiting patients at the Samsung Medical Center that is prospectively evaluating the efficacy and toxicity of salvage CRT with PBR for patients with previously irradiated recurrent rectal cancer. The primary outcome measure is 3-year LC rate, with secondary objectives that include adverse events, response rate, survival, and quality of life.
Anal
The role of radiation therapy
The current standard of care for squamous cell carcinoma of the anal canal was established by Radiation Therapy Oncology Group (RTOG) 9811 and includes definitive CRT with fluorouracil and mitomycin-C (MMC). However, studies have shown equivalent results from fluorouracil with cisplatin as concurrent treatment, so that regimen is also used at some centers, including MD Anderson. IMRT emerged as the new standard of care in the treatment of anal cancer when RTOG 0529, a phase II study, prospectively showed that IMRT could significantly reduce G2+ hematologic and G3+ dermatologic toxicity.
The rationale for proton therapy
The landmark studies establishing CRT as the standard of care used 3D treatment techniques. As such, large volumes of bowel, bladder, external genitalia, and skin received significant doses. On the MMC arm of RTOG 9811, G3–4 hematologic and nonhematologic acute toxicity rates were 61% and 74%, respectively. Although IMRT was shown to reduce toxicity, patients receiving IMRT with concurrent fluorouracil and MMC on RTOG 0529 still experienced 77% G2+ gastrointestinal, 73% G2+ hematologic, and 23% G3+ dermatologic toxicity rates. Emerging data also suggest that bone marrow dose may actually be higher with IMRT. The importance of bone marrow dose in predicting the risk of hematologic toxicity for patients receiving CRT for anal cancer has been established. Specifically, the volume of pelvic bone marrow receiving 5, 10, 15, and 20 Gy were all significantly associated with decreased white blood cell and absolute neutrophil count nadirs. PBR has the potential to preferentially spare bone marrow from unnecessary dose and potentially increase the tolerability of treatment. Additionally, PBR has the potential to decrease dose to the external genitalia, bowel, and bladder, which may further improve acute and long-term quality of life for patients receiving CRT for anal cancer. Hematologic, gastrointestinal, and dermatologic toxicity are common reasons patients need breaks during treatment. Because prolonging the overall treatment duration with breaks can worsen oncologic outcome, PBR thus has the potential to improve the efficacy of treatment.
Dosimetric studies
Two small treatment planning studies published in 2015 evaluated the potential benefits of pencil beam scanning proton beam therapy (SPBT) for anal cancer. , The first, performed at the Mayo Clinic, compared plans for eight patients with squamous cell carcinoma of the anal canal. The gross tumor volume plus margin received 54–60 Gy, and the elective nodal volumes received 45–50.4 Gy. They found no difference between target coverage between IMRT and SPBT. The SPBT plans were also robust to uncertainties, as evidenced by acceptable coverage even with the worse-case dose values. However, the mean doses to the bone marrow, bladder, small bowel, and genitalia were all significantly lower with SPBT than with IMRT. Specifically with regard to the bone marrow, SPBT reduced the pelvic bone marrow V10 by 54% ( P = .008), V20 by 56% ( P = .008) and V30 by 44% ( P = .008). 94 Another treatment planning study performed at the University of Pennsylvania also compared IMRT and pencil beam SPBT plans for patients with anal cancer. They similarly found no difference in target coverage, but they showed significant reductions in doses of up to 35 Gy to the small bowel ( P = .008), up to 29 Gy to the genitalia ( P = .008), and up to 30 Gy to the bone marrow ( P = .008). Fig. 10.3 shows the bone marrow and anterior viscera sparing achievable with IMPT compared with IMRT.
