Fig. 3.1
Histone deacetylases are responsible for counteracting the reaction of HATs by removing the acetyl groups from acetyl-lysine residue. However, they also target non-histone proteins that control cellular processes such as cell cycle, DNA repair and gene expression. Thus, inhibitors of HDACs impact a wide range of cellular function
Histone acetylation typically correlates with an increase in general transcription activity [17] while providing a platform for protein binding. Various transcription factors contain a bromodomain motif , which can recognize and bind to acetylated lysines on the histone tails [18, 19], serving to activate gene transcription by attracting further transcription complexes. On the contrary, histone deacetylation results in more closely condensed chromatin and the formation of more stable nucleosome interactions, leading to an overall inhibition of transcription [20–22]. Thus, HDACs function as enzymes, removing the acetyl group from a lysine of the histone tail. HDACs can also complex with transcriptional corepressors or protein-modifying enzymes to limit the accessibility of chromatin to transcription factors [23, 24] or to directly switch target transcription factors to their inactive form [25].
Given the effects of histone acetylation on chromatin dynamics , it is unsurprising that it has been implicated in the regulation of DNA repair, which is of utmost importance when considering targets for radiosensitization [26, 27]. The DNA damage response in human cells was first associated with histone acetylation when studies revealed that histones are very rapidly acetylated after ultraviolet radiation (UV) exposure [28]. This hyperacetylation of histones was accompanied by a more rapid repair of the DNA damage caused by the UV, leading to the hypothesis that the relaxing of chromatin initiated by acetylation allows the DNA repair machinery better access to the lesion [29]. The DNA damage caused by UV radiation is primarily thymine dimer formation, which is repaired differently from DNA double-strand breaks, the principal lesion caused by ionizing radiation (IR). As mentioned, one of the first chromatin events upon the formation of a DNA double-strand break in mammalian cells is the phosphorylation of H2AX to form γH2AX [7]. Acetylation of H2AX has also been observed to impact DNA repair after IR-induced DNA damage [30]. For example, the HAT TIP60 has been shown to acetylate H2AX on lysine 5 upon DNA damage [31]. TIP60 activity is regulated by SIRT1 which, when depleted, leads to hyperacetylation of H2AX lysine 5 and defective DNA damage response [32]. Deacetylation of histones has been shown to impact IR-induced DNA repair as well. Depletion of H3 lysine 56 acetylation has been shown to promote the nonhomologous end joining DNA repair pathway following IR [33].
Histone Acetylation and Metabolism
While alterations in global histone modification signatures are a hallmark of cancer, so is the metabolic reprogramming of tumor cells. Normal cells, under conditions of normal nutrient and oxygen levels, will generate energy (ATP) by utilizing the oxidation of pyruvate derived from glucose to carbon dioxide in the mitochondria via the tricarboxylic acid (TCA) cycle and the electron transport chain. In the absence of oxygen, cells are able to continue to produce energy via glycolysis and lactic acid fermentation in the cytosol. Cancer cells, as well as other populations of rapidly proliferating cells, preferentially employ glycolysis and lactic acid fermentation to produce ATP, even in the presence of sufficient levels of oxygen. This phenomenon is known as the Warburg effect , as it was first described by Otto Warburg in 1956 [34]. This process is far less efficient at generating ATP, but it may be advantageous for neoplastic cells to overutilize glycolysis, as it leads to increased biomass.
Interactions between metabolism and epigenetics are only beginning to be explored, but it is not surprising that the two are connected, as most histone-modifying enzymes require a substrate or cofactor that is a metabolic intermediate. Examples of this include the requirement for S-adenosyl methionine (SAM) during the transfer of methyl groups to histones by histone methyltransferases, the Jumanji-containing histone lysine demethylases dependence upon α-ketoglutarate, or the dependence of histone acetyl transferases on acetyl coenzyme A (acetyl-CoA) [35].
Given this relationship between histone acetylation and acetyl-CoA, a natural question to ask is if the levels of acetyl-CoA generated by glycolysis have an impact on chromatin structure and consequently transcription and DNA repair. Experiments have shown that feeding cell’s elevated glucose concentrations will increase levels of glycolysis, leading to an increase in cytosolic acetyl-CoA [35], which has been directly linked to histone acetylation [36]. Inversely, depletion of ATP-citrate lyase results in a decrease in histone acetylation [36]. This data suggests that the cytosolic level of acetyl-CoA produced during glycolysis is an important driver of histone acetylation. Furthermore, Liu and colleagues [37] explored the relationship between metabolism and DNA repair by inhibiting glycolysis using the inhibitor 2-deoxyglucose (2-DG) as well as siRNA against two rate-limiting enzymes in glycolysis (hexokinase I and pyruvate kinase) in a human lung carcinoma cell line. The inhibition of glycolysis in these cells produced a more compact chromatin structure in the nucleus. Since a more condensed chromatin assembly is often associated with histone deacetylation, they examined levels of protein acetylation in these cells and showed that acetylation of multiple lysine sites on histones H3, H4, H2A, and H2B were significantly decreased upon glycolysis inhibition, which was reversible with the addition of an HDAC inhibitor [37]. Furthermore, cells that were treated with inhibitors of glycolysis showed a decrease in DNA repair following treatment with a DNA-damaging agent, presumably because the condensed conformation of the chromatin prevented the DNA repair machinery from accessing the damage [37]. This is not the only study to examine the relationship between metabolism and DNA repair. Efimova and colleagues show that inhibition of glycolysis leads to the persistence of DNA double-strand breaks following DNA damage via ionizing irradiation as well as the acceleration of senescence [38]. Interestingly, this study was also able to show that the disruption of glutaminolysis impaired the DNA damage response of cells. Amplified glutamine uptake in cancer cells is, like glycolysis, thought to occur in order to increase the proliferating cell’s demand for biomass. This study indicates that glutamine metabolism, too, may be important for genome repair.
This relationship between glycolytic metabolism and the modulation of histone acetylation has implications for the radiosensitization of cells. We have already established that the DNA damage response and DNA repair mechanisms are of utmost importance when considering the radioresponse of cells and that HATs are key regulators of the DNA damage response as well as transcription. Additional studies have shown that glycolysis inhibition can sensitize cancer cells to DNA-damaging chemotherapeutics and radiation [39, 40]. Taken together, it is clear that targeting HDACs for inhibition could both increase the efficacy of DNA-damaging chemotherapeutics as well as be used as a strategy to preferentially target cells that rely heavily upon glycolysis , such as tumor cells .
Inhibitors of Histone Deacetylation
Aberrant expression of HDACs has been repeatedly demonstrated in human tumors, and certain HDACs (1, 5, and 7) have been shown to act as molecular biomarkers for tumor tissue [41]. Additionally, the overexpression of individual HDACs in several types of cancer correlates with a significant decrease in both disease-free and overall survival in patients and was predictive of poor patient prognosis independent of variables such as tumor type and disease progression [42–45]. Given this, considerable focus has been put into the development of clinically applicable HDAC inhibitors.
While new inhibitors are being generated continually, the most commonly used HDAC inhibitors are divided into general classes based on their chemical structure: hydroxamates , carboxylic acids (also known as short-chain fatty or aliphatic acids ), cyclic peptides , aminobenzamides , epoxyketones , and hybrid molecules [46]. The hydroxamic class of HDAC inhibitors includes suberoylanilide hydroxamic acid (SAHA) , and trichostatin A (TSA) . These compounds chelate zinc in the active site and contain a hydrophobic backbone that spans the active site of the hydrophobic pocket [47]. The carboxylic acids , which include sodium butyrate (NaB) and valproic acid (VPA) , target the active site zinc and, due to their smaller size and lack of hydrophobic backbone, are significantly less potent than the hydroxamic acids [48]. The cyclic peptide class contains the drugs depsipeptide , apicidin , and romidepsin ; all of which contain a cyclic ring. Aminobenzamides include MS-275 (entinostat), CI-994 (tacedinaline), and mocetinostat and function by attaching to the catalytic zinc ion as well [49]. Epoxyketones , which comprise trapoxins and 2-amino-8-oxo-9,10-epoxydecanoic acid, have an epoxyketone group that binds irreversibly to the catalytic site of the HDAC [50]. The final class, hybrid molecules, includes synthetic combinations of known compounds, specifically hydroxamic-acid-containing peptides having features of both hydroxamic acids and cyclic tetrapeptides [51]. Examples of this are tubacin and CUDC-101 [52].
The earliest inhibitors generated were not specific for a given HDAC but instead show slight preferences to either class I or II [53]. Despite this, current efforts to develop more advanced HDAC inhibitors trend toward isoform-selective deacetylase inhibitors [54]. Illustrations of this include tubacin, which was found to selectively inhibit HDAC6 deacetylation of α-tubulin [52], and PCI-34051, which was able to induce apoptosis in T-cell lymphomas by selectively binding HDAC8 [55]. Another focus of HDAC inhibitor development is the combination of inhibiting HDACs and other oncogenic proteins in the same molecule. These molecules allow the HDAC inhibition element to remain nonspecific, while targeting select oncogenic pathways like tyrosine kinases [56] or phosphatidylinositol 3-kinase (PI3K) [57]. The drug CUDC-101 , which inhibits EGFR-2 and EGFR in addition to HDACs, has been shown to enhance the radiosensitivity of GBM cells in vitro [58]. Other hybrid expansions include pairing an HDAC inhibitor with a topoisomerase II inhibitor (fusing daunorubicin with SAHA) or with a nuclear receptor target via a vitamin D receptor agonist [59]. While these technologies provide a promising future for the treatment of cancers, it is important to note that compounds from the primary classes of HDAC inhibitors have been clinically evaluated for antitumor activity [60].
HDAC Inhibitors and Radiosensitization
In Vitro Radiosensitization
Inhibition of HDACs results in the hyperacetylation of histones, which loosens chromatin structure and has effects on the radiosensitivity of cells. Studies of HDAC inhibition combined with radiotherapy date back to the 1980s, when J.T. Leith and colleagues demonstrated that the HDAC inhibitor sodium butyrate (NaB) increased the radiosensitivity of human colon carcinoma cell lines at relatively nontoxic concentrations [61]. At that time, NaB was considered a “differentiation-inducing agent ,” and its mechanism of action was yet to be elucidated. Radiation cell kill was attributed to “cell maturation ,” and the histone acetylation status of the tumor cells studied was not examined [62]. Because of its very short half-life and low achievable serum concentration, NaB has limited clinical applicability [63–65], but another HDAC inhibitor, trichostatin A (TSA) , was also initially shown to produce a significant increase in the in vitro radiosensitivity of human colon carcinoma cell lines [66]. Like NaB, TSA showed excessive cytotoxicity, apparently due to actions involving the acetylation on nonhistone proteins, and is unstable under in vivo conditions [16, 67]. Thus, although the earliest studies done with agents that inhibit HDACs were promising in that they were able to enhance the level of radiation-induced cell death, the challenge remained to find drugs suitable for clinical use.
Advances in drug discovery have produced a number of HDAC inhibitors with more promising in vivo pharmacokinetic and toxicity profiles. One of the first clinically applicable HDAC inhibitors with respect to radiosensitizing potential is the benzamide entinostat (MS-275). MS-275 is a potent HDAC inhibitor, has been reported to have in vivo antitumor activity in a number of preclinical models [49], and was the first clinically relevant HDAC inhibitor to be evaluated as a radiosensitizing agent [68]. Utilizing a human prostate carcinoma line (DU145) and human glioma line (U251), the effect of MS-275 on histone acetylation status was determined by exposing the cells to the drug for 6–48 h. An increase in levels of acetylated histones could be detected after 6 h of drug treatment , reaching a maximum level between 24 and 48 h in both cell lines. As histone acetylation is a dynamic process with some species of histones having an acetylation half-life of minutes [69]. The investigators then determined the dependence of the elevated acetylation levels on the presence of MS-275. This was achieved by exposing the cultures to the HDAC inhibitor for 48 h to induce maximum acetylation levels , followed by removal of the drug . The result was that histone hyperacetylation was significantly reduced by 6 h following drug removal, with a reduction close to control levels by 16–24 h. The rapid decline in histone hyperacetylation following drug removal has a significant role in the radiosensitization mechanism of HDAC inhibitors.
The investigators then asked if MS-275-induced hyperacetylation was correlated with alterations in tumor cell radiosensitivity. To answer this question, DU145 and U251 cells were exposed to MS-275 for 48 h, irradiated, trypsinized, and plated sparsely as a single-cell suspension to determine colony formation efficiency and radiation cell survival. The result was a minor increase in DU145 cell radiosensitivity , with no effect on U251 cell radiosensitivity . Because the mechanism behind HDAC-induced radiosensitization was unknown, it was possible that the hyperacetylation needed to be maintained after irradiation to increase radiation-induced cell death. When radiation clonogenic studies were performed with MS-275 both pre-radiation treatment and post-radiation treatment, the result was a significant increase in the radiosensitivity of DU145 and U251, with dose enhancement factors (DEFs) at a surviving fraction of 0.1 and 1.9 for DU145 and U251, respectively, compared to no increase in sensitivity when it was given only pre-radiation treatment. This work was significant because it was the first to demonstrate that there is a correlation between HDAC inhibitor-induced hyperacetylation and an increase in radiation sensitivity of tumor cells, as well as the first to show that the timing of the drug and radiation treatments were important.
These results led to parallel studies that examined other known HDAC inhibitors. One compound that had been studied extensively and quickly became of interest was valproic acid (VPA) . Its clinical values were discovered in 1963, as an antiseizure drug [70], and it has since become a well-established treatment of epilepsy and other seizure disorders [71, 72]. In general, VPA is well tolerated by patients, has few serious side effects, and is highly effective, making it a standard therapy for chronic epilepsy [71]. It was not until 2001 that VPA’s HDAC inhibitor activity was identified when Gottlicher and colleagues looked at VPA-treated hyperacetylationhyperacetylation of histone species H3 and H4 in human teratocarcinoma and HeLa cells [73]. Treatment with concentrations of VPA as low as 0.25 mM caused a significant increase in acetylated histone H4. Shortly thereafter, Phiel et al. reported that VPA activates Wnt-dependent gene expression through a pathway that involves directly inhibiting HDAC1 [74]. The chemical structure of VPA is similar to NaB, an eight-carbon branched-chained fatty acid, but in place of the 30 min half-life of NaB, VPA exhibits a serum half-life of 9–18 h and can be administered orally [71]. Importantly, VPA’s efficacy as an antiseizure medication proves that it can penetrate the blood-brain barrier and that it can be chronically administered with minimal toxicity. Combined, these factors generated considerable interest in VPA as a potential HDAC inhibitor for cancer patients.
Several studies have been done since, suggesting that VPA causes radiosensitization in vitro and in vivo. One study characterized the histone acetylation status of two human brain tumor lines, SF539 and U251, and showed that levels of acetylated H3 and H4 increased in a concentration-dependent manner after VPA treatment, reaching peak acetylation levels by 24 h [75]. Like MS-275, removal of VPA from cell culture media resulted in rapid loss of histone acetylation, indicating that histone hyperacetylation is dependent upon the continued presence of the HDAC inhibitor. In order to determine if VPA exposure, like MS-275, needed to be both pre- and post-irradiation in order to radiosensitize SF539 and U251 cells, experiments were carried out that mirrored the MS-275 study. The SF539 cells plated without post-irradiation VPA exhibited enhanced radiosensitivity with a DEF at a surviving fraction of 0.1 of 1.3, while the effect on U251 cells was negligible. However, the SF539 and U251 cultures that were plated with media containing VPA post-irradiation obtained DEFs of 1.6 and 1.5, respectively, demonstrating that exposure to VA, like MS-275, is required both before and after irradiation in order to increase tumor cell radiosensitivity. This effect of VPA on radiation sensitivity has been validated for other tumor types, including human erythroleukemic cells as well as esophageal squamous cell carcinomas [76, 77].
Numerous other HDAC inhibitors have shown radiosensitizing properties in various different tumor cell cultures , including SAHA in squamous cell carcinoma, prostate cancer cells, and pancreatic cancer cells as well as CI-994 and depsipeptide in glioblastoma [50, 78, 79]; phenylbutyrate in glioblastoma and hepatocellular carcinoma cells [80, 81]; tributyrin in melanoma cells [82]; PCI-24781 in cervical and colon carcinoma [83]; AR-42 in hepatocellular carcinoma cells [81]; and LBH589 in colon, breast, and lung cancer cells [84, 85].
In Vivo Radiosensitization
The in vitro data clearly demonstrates that HDAC inhibitors have the ability to enhance the radiosensitivity of a variety of tumor cell types by inhibiting HDAC function. Furthermore, these studies suggest that histone hyperacetylation may be used as a biomarker for radiosensitization. To further evaluate the antitumor potential of HDAC inhibitor and radiation combination therapy, in vivo xenograft model experiments have been performed.
The first xenograft experiments with MS-275 were performed by injection of MS-275 at 6 mg/kg every 12 h for up to three days. Mice were euthanized 6 h after 2, 4, or 6 injections in order to evaluate levels of acetylated histones in each tumor. Peak histone acetylation in DU145 xenografts was observed after 4 injections, with a rapid decrease in acetylation within 24 h of the last injection, consistent with the in vitro data [68]. A follow-up experiment was then designed in which radiation was delivered between the fourth and fifth of six MS-275 doses. Comparison of tumor growth rates revealed that MS-275 clearly enhanced radiation-induced tumor growth delay with a DEF of 2.8, signifying that MS-275 treatment increases the radiosensitivity of DU145 xenografts. In a similar study using U251 glioma xenografts , 150 mg/kg of VPA (a serum VPA concentration corresponding to that necessary to prevent seizures in humans) [72] was administered with a single dose of 4 Gy irradiation delivered 6 h after the third dose. Tumors showed a significant growth delay with a DEF of 2.6 for the combination treatments [75].
Other studies explored the actions of HDAC inhibitors FK228 and CBHA on human gastric and colorectal adenocarcinoma cells [86] and the effect of combination therapy of the HDAC inhibitor LBH589 on non-small cell lung cancers [87]. Collectively, these in vitro and in vivo analyses (summarized in Table 3.1) have afforded a rational basis for combining HDAC inhibitors with standard radiotherapy in both preclinical and clinical trials, a number of which are currently ongoing.
Table 3.1
HDAC inhibitors in preclinical/clinical trials (partial list)
Agent | Class | In vitro studies | Select tumor lines studied | Shown radiation modifying effects | In vivo studies | Clinical trials | References |
---|---|---|---|---|---|---|---|
Vorinostat (SAHA) | Hydroxamic acid | Yes | Squamous cell carcinoma | Yes | Yes | Yes | |
Prostate cancer | |||||||
Pancreatic cancer | |||||||
Leukemia | |||||||
TSA | Hydroxamic acid | Yes | Colon carcinoma | Yes | No | No | |
Melanoma | |||||||
NaB | Carboxylic acid | Yes | Melanoma | Yes | No | No | |
VPA | Carboxylic acid | Yes | Erythroleukemia | Yes | Yes | Yes | |
Esophageal squamous cell carcinoma | |||||||
Glioma | |||||||
Depsipeptide (romidepsin, FK228 ) | Cyclic peptide | Yes | Gastric adenocarcinoma | Yes | Yes | Yes | Miller et al. [102], Tan et al. (2010) |
Colorectal adenocarcinoma | |||||||
Apicidin | Cyclic peptide | Yes | Pancreatic carcinoma | No | No | ||
Entinostat (MS-275) | Aminobenzamide | Yes | Glioma | Yes | Yes | Yes | |
Tacedinaline (CI-944) | Aminobenzamide | Yes | Colon carcinoma | Yes | No | No | Beckers et al. [84] |
AML | |||||||
NSCLC | |||||||
Mocetinostat (MGCD103) | Aminobenzamide | Yes | Lung carcinoma | No | Yes | Yes | Saito et al. [49], Fournel et al. (2008) |
Colon carcinoma | |||||||
Trapoxin B | Epoxyketone | Yes | Colon carcinoma | No | Yes | No | Cerna et al. [50] |
Tubacin | Hydroxamic acid | Yes | Burkitt lymphoma | No | No | No | Haggarty et al. [52] |
ALL | |||||||
PCI-34051 | Hydroxamic acid | Yes | T-cell lymphoma | Yes | No | No | |
Phenylbutyrate | Carboxylic acid | Yes | Glioma | Yes | Yes | Yes | |
Hepatocellular carcinoma | |||||||
Melanoma | |||||||
AR-42 | Carboxylic acid | Yes | Hepatocellular carcinoma | Yes | Yes | Yes | Lu et al. [81] |
Abexinostat (PCI-24781) | Cyclic peptide | Yes | Cervical carcinoma | Yes | Yes | Yes | Gressette et al. (2014), Banuelos et al. [83] |
Colon carcinoma | |||||||
Neuroblastoma | |||||||
Panobinostat (LBH589) | Hydroxamic acid | Yes | Colon carcinoma | Yes | Yes | Yes | |
Breast carcinoma | |||||||
Non-small cell lung carcinoma | |||||||
CBHA | Hydroxamic acid | Yes | Gastric adenocarcinoma | Yes | Yes | No | Coffey et al. [51] |
Colorectal adenocarcinoma | |||||||
Belinostat (PXD-101) | Hydroxamic acid | Yes | Cervical carcinoma | No | Yes | Yes | |
Ovarian carcinoma | |||||||
Colon carcinoma |
Tumor Versus Normal Cells
It is thought that tumor cells alone would be susceptible to the cytotoxic and cytostatic effects of HDAC inhibitors due to aberrant histone deacetylase activity in tumor cells as compared to normal cells. For the most part, experimental evidence has backed up this theory, as shown by cell culture studies [102, 104] and animal models administered with clinically relevant HDAC inhibitors at antitumor doses [105–107]. One such study shows that following NaB exposure , melanoma cell lines were significantly radiosensitized, while normal cells remain unaffected [82]. The same group then found that a tumor radiosensitizing treatment of SAHA delivered to normal fibroblasts also had no effect on radiosensitivity [50]. In accordance with these studies, Kim et al. found that while HDAC inhibitors amplified the in vitro sensitivity of tumor cells to DNA-damaging drugs, they had no effect on the drug sensitivity of normal breast or intestinal cells [108]. Finally, the HDAC inhibitor Garcinol was found to radiosensitize human cervical adenocarcinoma line HeLa and large cell lung carcinoma line A549 to radiation, but not normal human fibroblasts [109].
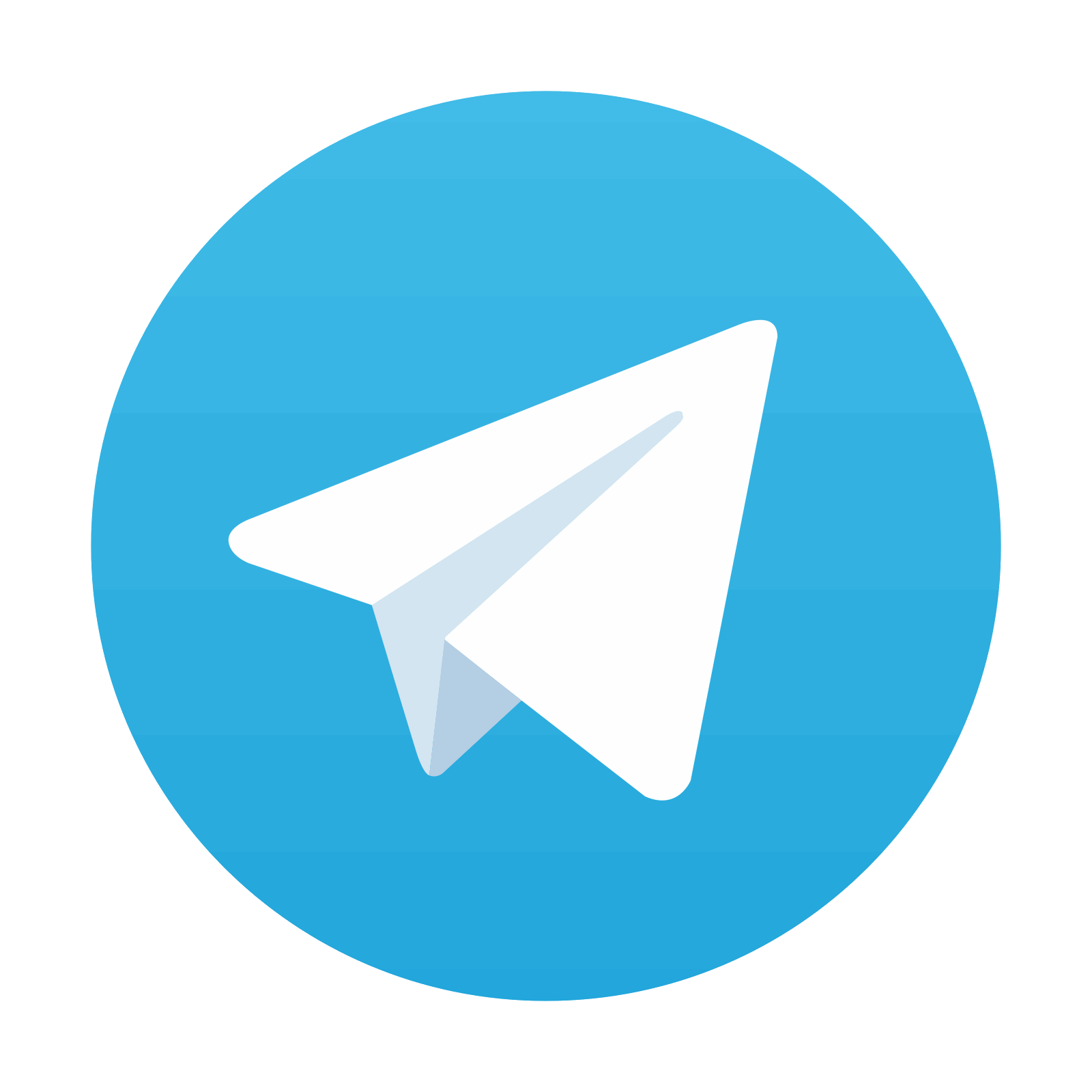
Stay updated, free articles. Join our Telegram channel

Full access? Get Clinical Tree
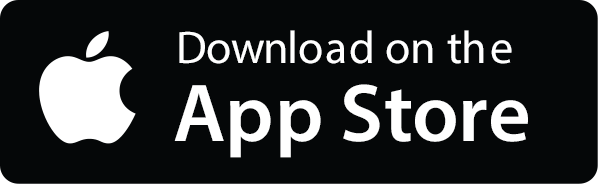
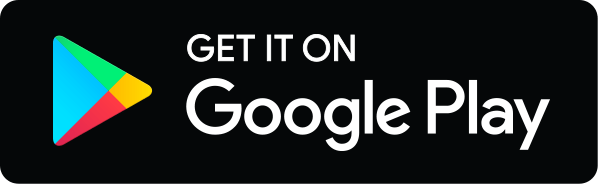