© Springer-Verlag London 2015
Andrew Gaya and Anand Mahadevan (eds.)Stereotactic Body Radiotherapy10.1007/978-0-85729-597-2_22. History and the Technological Evolution of Stereotactic Body Radiotherapy
(1)
Department of Radiation Oncology, Baylor Scott & White Health, Temple, TX, USA
(2)
Purdue University School of Health Sciences, 550 Stadium Mall Drive, West Lafayette, IN 47907, USA
Abstract
After the discovery of use of therapeutic radiation, tremendous advances have been made towards targeted radiation. 3D conformal and intensity modulation have led to conformal therapy minimizing normal tissue toxicity. Improvements in diagnostic technology in delineating tumors, complex planning algorithms, robotic tracking devices, and megavoltage and particle beams have led to use of ablative radiation in the body with minimal side effects.
Keywords
Stereotactic radiosurgeryExtracranialHypofractionation2.1 Introduction
Traditional radical radiation therapy delivery requires multiple fractions of 1.5–3 Gy administered daily over a period of 3–7 weeks. These regimens have been derived and calculated from widely accepted models of the radiobiological effect of X rays on human tissue. However, hypo-fractionated, or even single large dose fraction treatments, were practiced in early days of application of X-rays in the treatment of cancer. It has been observed that large doses per fraction were tumorcidal, especially for epithelial tumors, but early clinical experience provided also lessons regarding the balance between tumor control and normal tissue toxicities. Early evaluations of radiation therapy showed that the delivery of large dose per fraction treatments was leading to unacceptably high acute and consequential late normal tissue toxicities. Therefore, quite early in the development of radiation therapy these schedules were abandoned because of complications such as fibrosis, stenosis, and vascular injury. Data collected at the initial stages of the development of radiation therapy supported the understanding that large dose single fractions lead to unacceptable treatment complications.
The ability to manage the parameters underlying the unfavorable results for large dose per fraction therapy was severely limited in the early days of radiation therapy by the immature technologies of dose delivery. Particularly unfavorable were the relatively low energies of external beams used in the therapy. They were responsible for delivering large doses to normal tissues that were situated between skin and the target. These early experiences and their impact on the acceptance of particular fractionation schemes have been largely forgotten by later practitioners of radiation therapy, even when the megavoltage energies started to become available in radiation therapy. The paradigm of delivering a dose in small daily fractions has been taken for granted, and disconnected from the reflection that early treatments suffered multiple dosimetric limitations resulting from inadequate physical parameters of beams and unsophisticated therapy delivery technology. The exception to this was the treatment proposed by neurosurgeons in the Karolinska Institute; Dr. Leksell, inspired by the availability of high energy, megavoltage Cobalt beams, designed the radiosurgical treatment technique for brain known as Gamma Knife. The technique was not only utilizing the ability to move highly energetic photons to the targeted tissue in brain without depositing excessive energy to cells located between beam entrance at the skull surface and the target, but also relying on the relatively small separation distance between target tissue and the skull surface, and on limiting dose to healthy tissue by moving photons concentrically on the target from many directions (using 201 Cobalt sources).
Characteristic of this technique was that volumes exposed to high dose were relatively small in comparison to the total mass of brain tissue and delivered with high geometrical precision to targets (brain structures have fixed position relative to the skull). These properties of permanent localization of brain structures relative to the skull were helping to achieve the high accuracy of dose delivery when assisted by precise fixation of the skull relative to the Gamma Knife focus through a frame attached surgically to the patient’s skull. The precision achieved by the attachment of the frame to the skull made it very inconvenient to irradiate brain in multiple fractions; therefore the regime of single fraction treatment was established for this radiosurgical procedure. The single fraction treatment made radiation oncologists who were practicing multi-fraction radiotherapy delivery skeptical about the radiosurgical treatment mode. Nevertheless, positive outcomes of these treatments accumulating over many years of clinical use of the technique (high success in target ablation and relatively small treatment toxicity with limited and manageable complications) gave enough evidence to reconsider the paradigm of multi-fraction treatments in radiotherapy.
Attempts to transfer the Gamma Knife experience to extracranial treatments were tried in Karolinska. However, initial attempts to transfer Gamma Knife experience to extracranial sites exposed differences in both therapies that pose technical difficulties [1].
First of all most extracranial structures are not fixed in position relative to the skin and so having information about location of the body surface relative to accelerator focus (isocenter) does not guarantee that the target is precisely positioned relative to irradiating beams. Moreover, extracranial organs are moving both interfraction and intrafraction, and so their location at treatment may be different from their location at simulation and planning, even if we were able to reproduce the position of the target relative to machine focus with perfect precision before treatment was initiated. Therefore therapy practitioners who wanted to transfer cranial radiosurgery experience to extra cranial targets faced considerable technical challenges. The precise positioning of the target in the isocenter of the machine required setting a target by coordinate system derived from imaging of the body, together with verification that body geometry during treatment was close to identical to body geometry at the time of simulation and planning. Finally, there was a clear indication of the need for elimination, or significant suppression at least, of the body structures motion during treatment.
These conditions were to a large degree achieved by the body frame designed for this treatment in Karolinska by Drs. Henrik Blomgren and Ingmar Lax [1]. A treatment technique for extracranial radiosurgery was thus proposed by these researchers. The body frame allowed comfortable repositioning of the patient’s body relative to the frame, equipped with metallic fiducial markers determining the system of coordinates relative to the frame geometry that was easily and accurately localizable relative to the room coordinate system of the simulator and the treatment accelerator.
The abdominal compression attached to the body frame allowed for minimization of respiratory motion of the organs within the chest and abdomen. Reproducible positioning of the patient’s body with respect to frame assured, after accurate placement of the frame within the room system of coordinates, close correlation between treatment room system of coordinates of the target and organs at risk relative to accelerator isocenter and relative to spatial geometry of treatment beams.
Rescanning patients before each treatment fraction assured moreover the geometry of the body at treatment conformed to the body geometry at simulation and planning as referred to the body frame. Application of abdominal compression made the breathing motion small enough to keep margins around the target from 5 to 10 mm guaranteeing the volume of high dose exposure to be small. To keep the volume of normal tissues exposed to high dose per fraction in extracranial radiosurgery small, the original recommendation for hypofractionated treatment was to not treat targets exceeding 7 cm diameter. The final recommendation of Karolinska clinicians was that multiple beams converging on the target are used to concentrate high dose volume only in the target and its close vicinity. Following these recommendations assured that the dose distribution in extracranial radiosurgery was similar with standards of dose distribution utilized by Gamma Knife intracranial radiosurgery. These properties of extracranial dose characteristics gave them the confidence of recommending hypofractionated treatment, following the experience of Gamma Knife cranial radiosurgery. Generally three to five fractions (ranging between 8 and 20 Gy. per fraction) were used for extracranial radiosurgery with these characteristics. To obviate radiobiological uncertainties, these treatments were recommended initially for targets situated in parallel organs such as lung and liver.
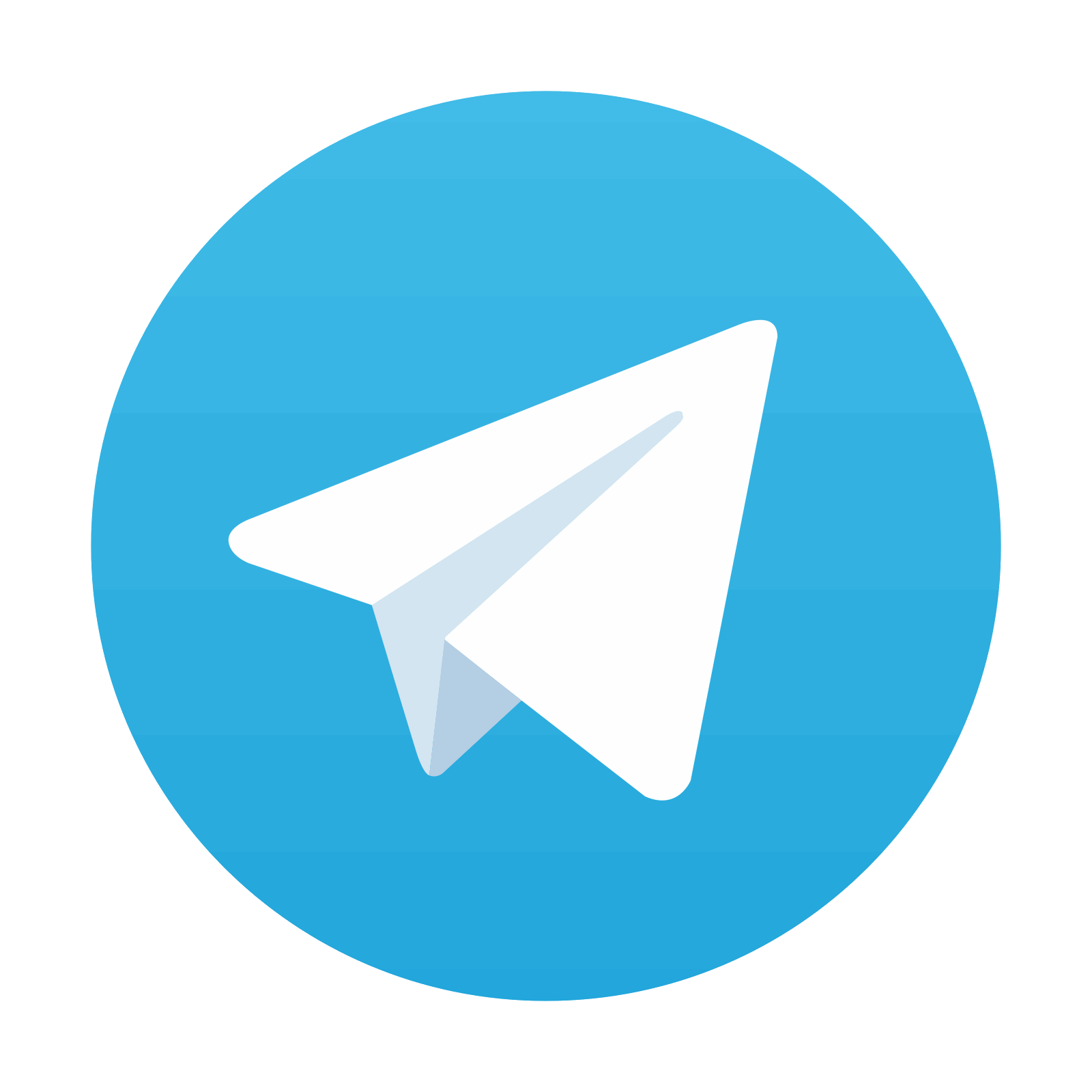
Stay updated, free articles. Join our Telegram channel

Full access? Get Clinical Tree
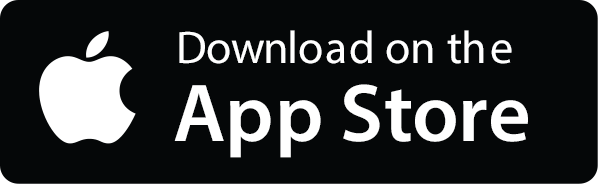
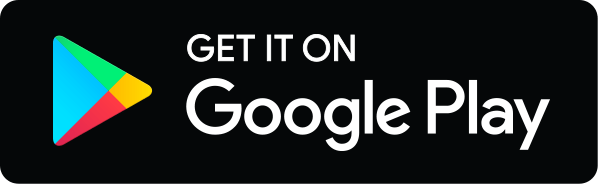