Fig. 1
53-year-old male with small bowel adenocarcinoma metastatic to the liver undergoing radioembolization preparatory angiogram. a Aortogram showed a replaced left hepatic artery (white arrow) arising off the left gastric. Note the lack of any arteries feeding the left hepatic lobe arising from the proper hepatic artery (black arrow), b Selective angiogram of the gastrohepatic trunk confirmed the replaced left hepatic artery (white arrow) sharing a common origin as the left gastric artery (black arrow). This represents a Michels Type II configuration of hepatic arterial anatomy and is the most common variant
Table 1
Michels classification of variant hepatic arterial anatomy
Michels type | Description | Incidencea (%) |
---|---|---|
I | Standard anatomy with right hepatic, middle hepatic, and left hepatic arising from the celiac axis | 55 |
II | Replaced left hepatic from left gastric artery | 11 |
III | Replaced right hepatic from superior mesenteric artery | 10 |
IV | Replaced right hepatic from superior mesenteric artery; replaced left hepatic from the left gastric artery; middle hepatic from celiac artery | 1 |
V | Accessory left hepatic from left gastric artery | 8 |
VI | Accessory right hepatic from superior mesenteric artery | 7 |
VII | Accessory right hepatic from superior mesenteric artery; accessory left hepatic from left gastric artery | 1 |
VIII | Combination of replaced right hepatic with accessory left hepatic OR accessory right hepatic with replaced left hepatic | 2 |
IX | Common hepatic from superior mesenteric artery | 2.5 |
X | Common hepatic from the left gastric artery | <1 |
A study evaluating 600 patients that had undergone angiography showed a similar distribution of patients with variant hepatic arterial anatomy (Covey et al. 2002). Standard anatomy was seen in 61.3 % of patients. The most common variant was an aLHA arising from the LGA in 10.7 % of patients, and an rRHA from the SMA in 8.7 % of patients. In addition, several other variants were present that were not mentioned in Michels’ study, including an origin of the CHA directly from the aorta, and a “double hepatic” artery where one or both of the left and right hepatic arteries arose directly from the aorta or from the celiac artery. These two studies demonstrate the wide variability that can occur with variant hepatic arterial anatomy.
The perihilar plexus includes arteries that provide a communicating arcade between the right and left hepatic arteries (Tohma et al. 2005). This arcade connects the segment IV branch or the main LHA with the main or anterior trunk of the RHA. Intrahepatic communications between segments also exist, and provide collateral flow when branch hepatic arteries are occluded or compromised. These interlobar and intersegmental communicating branches were described several decades ago during studies of patients after hepatic arterial ligation for trauma or tumor treatment. Proximal interruption of any major hepatic artery, such as the RHA or LHA, results in near immediate filling via cross collaterals of the occluded branch (Charnsangavej et al. 1982; Mays and Wheeler 1974). This property was successfully exploited over 30 years ago in consolidation of flow for intra-arterial chemotherapy (Chuang and Wallace 1980). Familiarity with and evaluation of these arcades are important in expanding options concerning catheter placement for radioembolization.
Michels also recognized the importance of extrahepatic blood supply to the liver. He categorized 16 different routes, apart from the hepatic arterial variants, from which blood could supply parts of the liver (Michels 1966). The extrahepatic branches described included inferior phrenic, internal mammary, and intercostal arteries (Fig. 2). Other studies have shown that tumors near the surface of the liver are more likely to recruit extrahepatic blood supply, which become particularly evident when there has been compromise of normal intrahepatic arteries, for instance, from intra-arterial therapies (Seki et al. 1998). Parasitized extrahepatic arteries frequently supply tumors at the bare area of the liver, even prior to any treatment, and are a cause of recurrence after chemoembolization of intrahepatic supplying branches (Miyayama et al. 2010). Therefore, these potential routes require close attention and appropriate recognition in the evaluation of radioembolization patients, since unmanaged they can lead to incomplete treatment and recurrence after treatment.
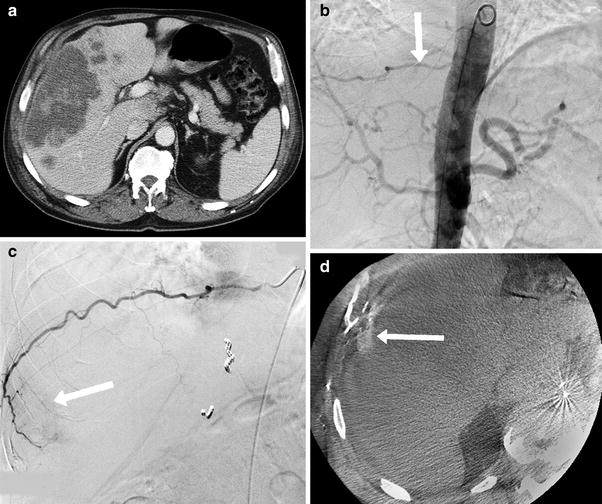
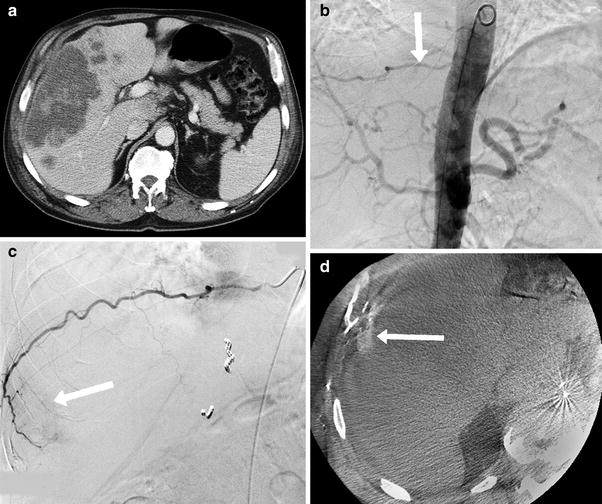
Fig. 2
63-year-old male with cholangiocarcinoma undergoing radioembolization preparatory angiogram. a Pre-procedure CT scan showed a large tumor along the anterolateral margin of the right lobe of the liver, b Aortogram showed a hypertrophied right T9 intercostal artery (white arrow), c Selective angiography of T9 showed tumor blush (white arrow), d CACT on selective injection of the right T9 intercostal artery confirmed tumor enhancement (white arrow). To deliver radioactive microspheres to this territory, the parasitized intercostal artery was pre-emptively bland embolized with large particles to re-establish intrahepatic perfusion from the hepatic artery
Special attention should be paid to several suspect vessels that are the most common parasitized extrahepatic arterial sources of tumor supply, which can be found in about 18 % of untreated patients. The most common is the right inferior phrenic artery, which one study found to be the supply in almost half of all patients where an extrahepatic source was found (Chung et al. 2006). The same study found that greater omental arteries were the extrahepatic source in 15.6 % of cases, with cystic, adrenal, and intercostal arteries accounting for 5.4–8.8 % each. Much less frequent were left and right gastric, right and left internal mammary, renal or renal capsular, superior mesenteric, left inferior phrenic, and pancreaticoduodenal arteries.
For optimum treatment–complete treatment of all intrahepatic tumors and avoidance of intra and extrahepatic non-target embolization-hepatic arterial variants, and parasitized extrahepatic vessels need to be addressed during planning of radioembolization.
2 Redistribution and Consolidation of Hepatic Arterial Flow
2.1 Redistribution
Intrahepatic collateral vessels can supply arterial flow to tumors across segments or lobes. Selective embolization of intrahepatic branches to redistribute intrahepatic flow patterns to the tumors has been shown to be effective and safe (Karunanithy et al. 2011; Bilbao et al. 2010). During evaluation of intrahepatic arterial tumor supply, multiple feeding vessels may be identified, often in close proximity to hepatico-enteric or hepatico-splanchnic vessels. Although, pre-emptive coil embolization of the hepatico-enteric vessels imparts a high degree of safety, some vessels may be too small or angulated to allow this skeletonization. Embolizing one or more intrahepatic feeding branches can reduce the number of sites of administration of radioactive microspheres, and can facilitate administration distal to recognized hepatico-enteric vessels. For instance, a disadvantageous segment IV artery with small ductal artery branches can be coil embolized so that arterial supply to that segment is taken over by branches of the LHA or RHA or both (Fig. 3). Alternatively, coil embolization of a segment VIII artery supplying the lateral edge of a left lobe tumor can reduce treatment to only the LHA, sparing the remainder of the right lobe.
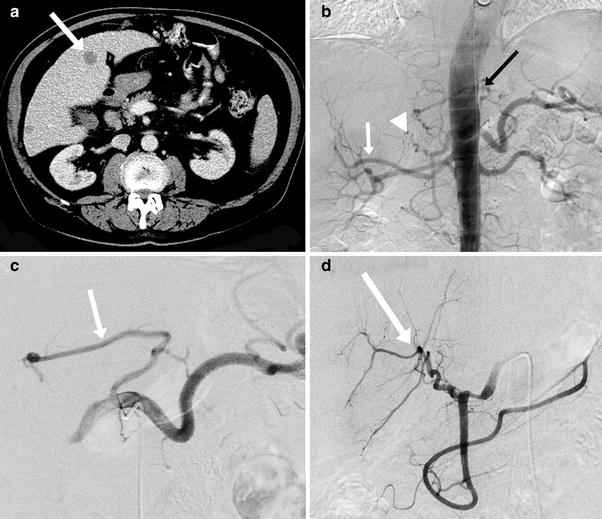
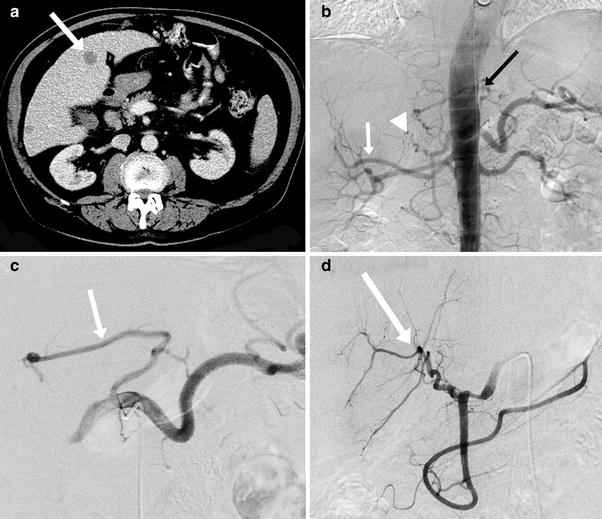
Fig. 3
74-year-old male with rectal cancer metastatic to the liver undergoing radioembolization preparatory angiogram. a Pre-procedure CT scan showed bilobar metastases including in segment 4 (white arrow), b Aortogram showed a replaced right hepatic artery (white arrow), accessory left hepatic artery (black arrow), and a segment 4 artery arising off the proper hepatic artery (white arrowhead), c Selective angiography of the celiac artery confirmed the accessory left hepatic artery (white arrow), d Common hepatic arteriogram better demonstrated the segment 4 artery arising off the proper hepatic artery (white arrow). In this instance, embolization of the segment 4 artery could be performed to consolidate flow to the replaced right and left hepatic arteries in order to reduce the number of treatment sites
In a study of 24 patients, 11 of whom had Michels Type I anatomy, single photon emission computed tomography (SPECT) combined with computed tomography (CT) performed after administration of technetium macroaggregated albumin (99mTC-MAA) showed uptake in the redistributed areas in all 11 Michels Type I patients (Bilbao et al. 2010). Branches embolized include segment IV, segment VIII, and LHA. In a separate study, 11 patients underwent embolization of the anterior division RHA, RHA, segment IV, or LHA to redistribute flow for administration of radioactive microspheres (Karunanithy et al. 2011). Post-treatment PET showed a statistically significant decrease in standardized uptake values (SUV). These studies demonstrate that embolization of intrahepatic branches from either the left lobe or right lobe can successfully redistribute flow to simplify and increase the safety of treatment. It is important to note that both studies performed embolization with coils only. More distal embolization using particles would lodge in the tumor at the arteriolar level (Lee et al. 2008). This could theoretically prevent radioactive microspheres from reaching the tumor.
2.2 Consolidation
A similar technique for management of variant hepatic arteries can be used. The goal of consolidation is to create a simpler and safer arterial anatomy for the administration of radioactive microspheres. Since many variant hepatic arteries arise off of branches that also supply the gastrointestinal tract, non-target radioembolization is an increased risk (Riaz et al. 2009). For instance, administration in the rLHA or aLHA originating from the LGA can result in reflux into esophageal and gastric branches just proximal to the course of the variant artery in the fissure of the ligamentum venosum. Likewise, the rRHA or aRHA frequently gives off small branches to the duodenum, and arises from the main SMA, which supplies nearly the entire bowel (Fig. 4). One early study on radioembolization safety consolidated variant hepatic arteries with resultant reconstitution of flow by intrahepatic collaterals and is important because it demonstrated that consolidation is able to limit toxicity, although treatment efficacy was not fully assessed (Andrews et al. 1994). Consolidation by coil embolization of variant or redundant arteries provides a way to achieve distribution of microspheres to the targeted tumors while minimizing non-target deposition complication risk to the patient.
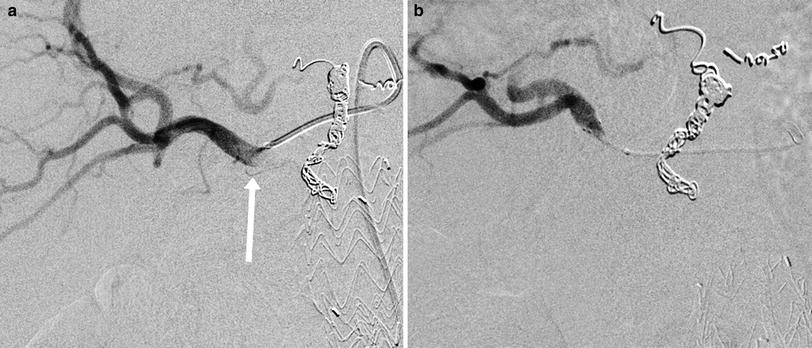
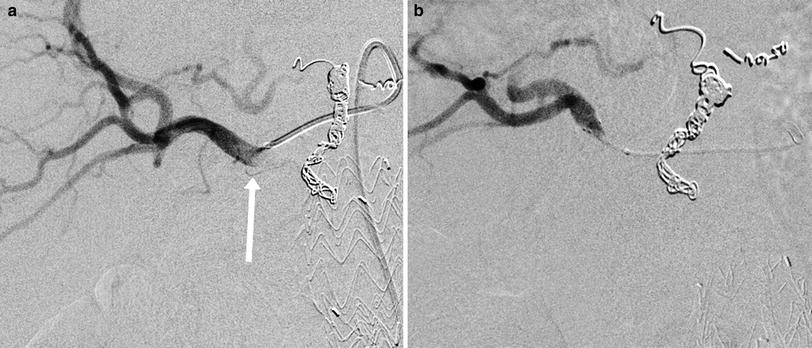
Fig. 4
69-year-old male with colorectal cancer metastatic to the liver undergoing radioembolization treatment. a Angiography of a replaced right hepatic artery arising from the SMA revealed small proximal branches supplying duodenum (white arrow), b Because of the multiplicity, small sizes, and acute angulations, the arteries could not be coil embolized and a reflux protection device (Surefire Medical Inc., Westminster, CO) was used to reduce the risk of radioembolic bead delivery to the bowel. A great deal of biological variability is found in the enteric branching patterns of replaced and accessory right hepatic arteries
In evaluating for any evidence of variant anatomy, all prior cross-sectional imaging, either contrast enhanced computed tomography (CT) or magnetic resonance (MR) imaging, should be closely studied. Thin-section arterial phase breath-held imaging is the most useful, if available, and coronal and sagittal reformatted images may help to confirm existence, origin, and course of variant vessels. Identified anatomic variants should be compared to the intrahepatic tumor distribution to predict dominant arterial supply to the targeted regions. Scrutiny of cross-sectional imaging will guide and possibly even expedite the preparatory angiography prior to radioembolization treatment.
During preparatory phase angiography, abdominal aortography is performed with injection of contrast medium at up to 15 cc/sec for 30 cc with the flush catheter at the level of mid to lower thorax (T7–T9) to identify variant hepatic and parasitized extrahepatic arteries, including those too small to be detected by CT or MRI, and to establish a baseline for future comparison. Next, all arteries of interest including normal and variant and parasitized vessels should undergo catheter selection and selective digital subtraction angiography (DSA). When available, C-arm cone beam CT (CACT) should also be performed for volumetric definition of subtended arterial territory. If no variant or parasitized arteries are identified, DSA and CACT should be performed while injecting contrast medium into the PHA or CHA. If any territories and especially if any tumors within the liver do not enhance, the search for additional arterial inflow should be renewed (Fig. 5). Selective catheterization and injection of the mesenteric vessels are performed as needed to confirm anatomy of the SMA, CHA, PHA, GDA, LHA, and RHA. Because of the high incidence of hepatofugal branches arising from the LHA, including the RGA, accessory LGA, left inferior phrenic artery, and falciform artery, some authors recommend power-injected angiography of each LHA (Lewandowski et al. 2007).
