Image-Guided Robotic Radiosurgery
Radiosurgery combines principles of stereotactic localization with multiple cross-fired beams from a highly collimated high energy radiation source.1 This noninvasive technique has proven to be an effective alternative to conventional neurosurgery and irradiation for selected small cranial tumors and arteriovenous malformations. Because virtually all existing stereotactic techniques rely on rigid target fixation, almost all cases treated with radiosurgery to date have involved intracranial lesions. Recent improvements in high-speed computing, radiographic imaging, and lightweight linear accelerator design have led to the development of the Cyberknife: a frameless image-guided robotic stereotactic radiosurgical system. The Cyberknife has overcome many of the limitations of existing radiosurgical systems.
Constraints of Existing Radiosurgical Systems
Current stereotactic radiosurgery systems have several constraints. Existing cranial frame-based systems only allow access to intracranial or the highest cervical lesions. Previous attempts at extracranial stereotactic radiosurgery involved spinal tumors, and these have involved prototypes that require either open surgery2 or transcutaneous placement of clamps3 to fix bony processes. A more recent report reviewed 19 patients with spine metastases treated with modified linear accelerator radiosurgery using the surgical placement of metal clamps for rigid fixation.4 However, this surgery, along with the necessary prolonged anesthesia, subjects a patient to potential complications (two wound infections noted in the above 19 patients) and a lengthy procedure when the open procedure is combined with the radiosurgical treatment. Fixed frames also limit the treatment degrees of freedom, and the metal components of current frames produce imaging artifacts on computed tomographic (CT) and magnetic resonance imaging (MRI) scans. Finally, the discomfort associated with skeletal fixation makes fractionation impractical and the treatment of children difficult.
Standard radiosurgical instruments such as the Gamma Knife (Elekta, Atlanta, GA) and conventional linear accelerators utilize a fixed isocenter to which all radiation beams converge. This design works well with spherical targets but is not ideal for complex or irregular shapes. To treat nonspherical lesions, these radiosurgery methods rely on multiple overlapping spherical dose volumes. The disadvantage of this approach is dose heterogeneity; some portions of the target are overdosed whereas other regions are underdosed. A system that involves both shape matching and increased dose homogeneity would improve treatment. Furthermore, a frameless stereotactic radiosurgery system with greater degrees of freedom would allow treatment of extracranial and even nonneural tumors.
To address these limitations, the Cyberknife, a radically new technology that uses high-speed computers, noninvasive image-guided localization, a lightweight high-energy radiation source, and a robotic delivery system has been developed by Accuray, Inc. (Sunnyvale, CA, USA). The Cyberknife has been used to treat all types of intracranial lesions, but more importantly, has expanded the use of radiosurgery to treat extracranial lesions within the spine, thorax, and abdomen.
The Cyberknife: Technical Characteristics
The Cyberknife (Accuray, Palo Alto, CA) (Fig. 12–1) combines three advanced technologies to deliver frameless conformal radiosurgical doses. The first is a lightweight 6 MV X-band linear accelerator (LINAC), designed especially for radiosurgery and mounted to a highly maneuverable robotic manipulator (GMFanuc, Auborn Hills, MI, USA). Because the Cyberknife operates at an RF frequency of about 7.5 GHz, compared with 2.9 GHz for a typical medical S-band LINAC, the dimensions of the 6 MV accelerator cavity are decreased by a factor of 2.5. Less shielding is required, and the collimators for narrow radiosurgical fields are much smaller than those required for large therapeutic fields. This leads to an X-band accelerator head that measures 25 cm by 45 cm by 70 cm and weighs only 130 kg. A LINAC of this size can be carried by the robotic arm, whereas the heavier clinical S-band accelerators are far beyond the load limits of robotic arms and require a substantial gantry mechanism, which limits their positioning capability. The robot can position and point the LINAC with six degrees of freedom and has a pointing precision of 0.3 mm.
The second innovation incorporated in the Cyberknife is real-time image guidance, which eliminates the need to position and rigidly immobilize the target via skeletal fixation (e.g., with a frame). This imaging system acquires radiographs of skeletal features associated with the treatment site, uses image registration techniques to determine the treatment site’s coordinates with respect to the LINAC robot, and transmits the target coordinates to the robot, which then directs the beam to the treatment site. If the target moves, the process detects the change and corrects beam pointing. This process is rapid enough that the system reacts in near real time to changes in the patient’s position.
The third innovation has been the development of amorphous-silicon detectors, which has allowed improved radiographic imaging. The Cyberknife localization method can in principle be used wherever radiopaque features are associated with an anatomic target, a concept that allows the extension of radiosurgical technique to extracranial sites. Frameless radiosurgery has already been used to treat sites within the cervical spine5–8 prior to the use of amorphous silicon detectors. Earlier x-ray cameras were fluoroscopes consisting of a gadolinium oxysulfide screen viewed by a light-amplified video charge-couple device (CCD). Lens optics require that the CCD be 60 cm from the screen, which results in (1) poor signal-to-noise at low exposure levels, (2) low contrast, and (3) significant veiling glare. This design has made it difficult to obtain good-quality images of the skeletal anatomy within and around the thorax and abdomen. To overcome these limitations, the previous cameras in the Cyberknife have been replaced with flatpanel amorphous-silicon x-ray cameras (dpiX, Palo Alto, CA).9 These devices have a pixel pitch of 0.125 mm and acquire flat images that avoid distortions inherent to lensed or x-ray image intensifier techniques. When images from these sensors are processed by the new sixdimension registration software, a tenfold improvement in spatial resolution results. This imaging software and hardware have been specifically designed to provide variable fields of view and magnification ranges that can be adapted to multiple anatomic locations. For example, amorphous silicon x-ray sensors create a high-quality image of the lumbar spine using the typical Cyberknife imaging geometry (10 mAs, 75 kV x-ray exposure9). Such an exposure corresponds to a dose per image of approximately 25 mrads.
FIGURE 12–1. A schematic of the image-guided radiosurgery system, identifying the major system components. The 6 MV X-band linear accelerator (LINAC) (a) is mounted on the arm (b) of the robotic manipulator. The treatment couch (c) is positioned between the two x-ray cameras (d) and their respective diagnostic x-ray tubes (e) (Cyberknife, Accuray, Palo Alto, CA).
Target Localization
The components of the imaging system are fixed at known positions within the treatment room. This provides a stationary frame of reference for locating the patient’s anatomy, which in turn has a known relationship to the reference frame of the robotic arm and LINAC. As with conventional forms of brain stereotaxy, this approach assumes a fixed relationship between the target and the skeletal system. Once the skeletal system has been located within the imaging system’s coordinate frame, the position of the lesion is known. The Cyberknife determines the location of the skull or spine in the coordinate frame of the radiation delivery system by comparing digitally reconstructed radiographs (DRRs) derived from the treatment planning CT study with radiographs acquired by the real-time imaging system.
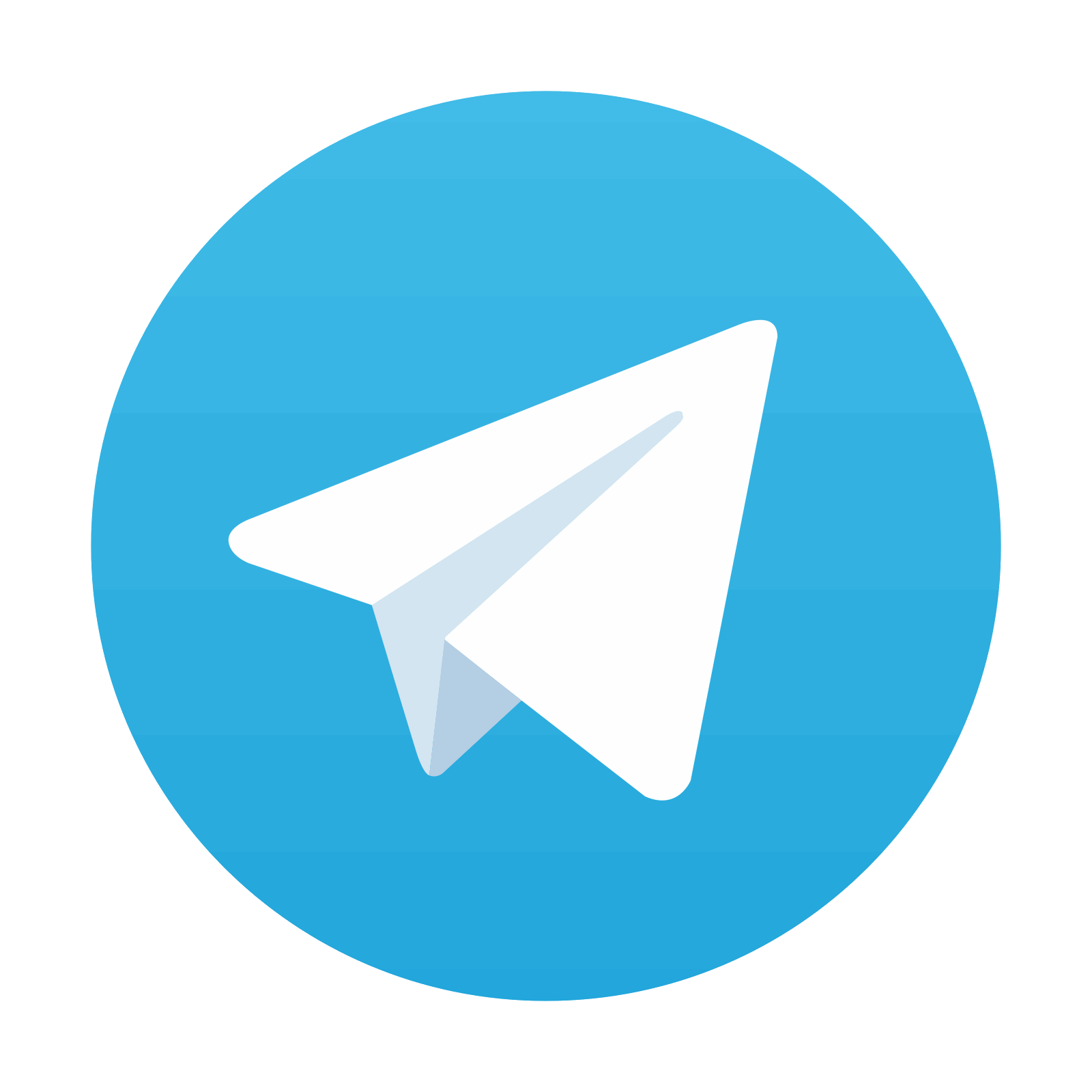
Stay updated, free articles. Join our Telegram channel

Full access? Get Clinical Tree
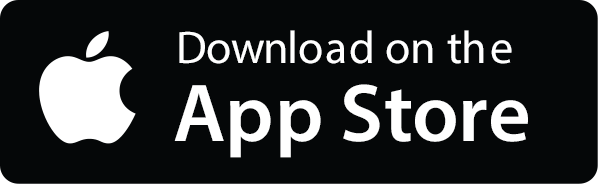
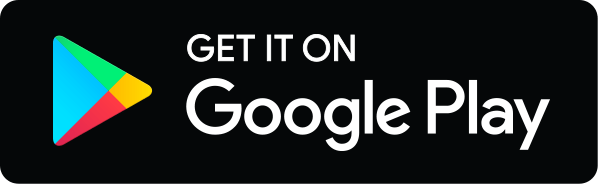