4Image-Guided Stereotactic Radiosurgery
Jasvinder Nangiana, John R. Adler Jr., and Steven D. Chang
Stereotactic radiosurgery combines stereotactic localization with multiple cross-fired beams from a highly collimated high-energy radiation source. This method of noninvasive ablation has proven to be an effective alternative to conventional neurosurgery, cranial irradiation, and brachytherapy for selected small cranial tumors and arteriovenous malformations (AVMs). Most current and historical stereotactic techniques rely on a rigid frame fixed to a patient’s skull for head immobilization and target localization. Despite being an effective alternative to invasive neurosurgery and irradiation, frame-based radiosurgery is not ideal for all patient populations. Because they require immobilization, invasive and noninvasive frames are particularly challenging for pediatric patients. Children often require general anesthesia during application of the stereotactic frame and subsequent treatment. Additionally, infants cannot be suitably treated with radiosurgery due to the malleable nature of their skull.
Furthermore, the usefulness of frame-based radiosurgery is largely limited to intracranial tumors. Extracranial lesions beyond the highest cervical level typically cannot be adequately targeted. Although body stereotactic frames have been previously used in a small number of cases, frame-based technologies offer little practicality for spinal lesions, as patients were subjected to lengthy procedures involving placement of metal clamps on spinous processes, exposing them to general anesthesia and the possibility of wound infections.1 Finally, a fixed frame limits the treatment degrees of freedom, and the metal components of current frames produce imaging artifacts on computed tomography (CT) and magnetic resonance imaging (MRI) scans.
The standard radiosurgery instruments such as the Gamma Knife and conventional linear accelerators are designed to deliver radiation based on a fixed isocenter. This works well with spherical targets but is not ideal for complex or irregular shapes. To treat complex shaped tumors, radiosurgery relies on overlapping spherical dose volumes, a method that results in intralesional dose heterogeneity. A system that achieves shape matching without significantly compromising dose homogeneity could be particularly advantageous for treating many intracranial lesions.
Image-Guided Radiosurgery
Image-guided radiosurgery in the form of the CyberKnife (Accuray Inc., Sunnyvale, CA; Fig. 4.1) was developed as a frameless alternative to conventional intracranial radiosurgery.2 Similar to other stereotactic methods, the system presumes a fixed relationship between the target and the skull. However, the CyberKnife uses real-time image guidance to direct therapy, eliminating the need for skeletal immobilization. Along with noninvasive image-guided localization, a lightweight, high-energy radiation source and a robotic delivery system enable the CyberKnife to overcome the limitations of previous modalities. This noninvasive method has proven to be effective for the treatment of intracranial tumors and AVMs, as well as extracranial targets within the spine.
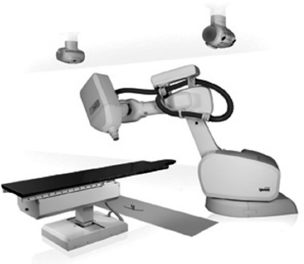
Fig. 4.1 The CyberKnife. From Accuray, Inc. Reprinted with permission.
The CyberKnife consists of several distinct parts. The first component is a 130 kg, 6 MV x-ray linear accelerator (linac). This linac is sufficiently light enough to be mounted to a commercially available robotic arm (the second component) that allows it to be pointed with six degrees of freedom and achieve an average treatment precision of 0.3 mm.3 Compared with a gantry-mounted linac that moves in a planar arc and points to a fixed isocenter, the CyberKnife’s unique maneuverability enables it to be positioned to any point in space, thus providing for much greater flexibility and allowing nonisocentric treatment delivery. Furthermore, over the last several years, the dose output of the CyberKnife linac has increased, resulting in reduction of overall patient treatment times.
High-speed computers represent the third component of the CyberKnife. By calculating the location of the treatment site before administering each dose of radiation, the CyberKnife is able to adjust and compensate for the movements of the patient. The fourth distinct feature of the CyberKnife is an x-ray imaging device (amorphous silicon detectors) placed in the floor of the treatment room on both sides of the patient.4 Initial alignment radiographs are taken to determine the location of the target within the robotic coordinate system prior to treatment. These real-time digital radiographs are registered to digitally reconstructed radiographs (DRRs) from the treatment planning CT study, enabling the skull or spine (and thus treatment) position to be translated to the coordinate frame of the linac. The robotic arm receives information about the target position and compensates for any patient movement by aligning the linac with the treatment site. After the beam is adjusted and realigned with the target, a preplanned radiation dose is administered. Real-time digital radiographs are captured at repeated intervals as the robotic arm moves the linac to ~100 preset points, called nodes, surrounding the patient. Anatomical position can be evaluated at each node, enabling recalibration of the target position before administering each dose of radiation.
This imaging system also allows spinal lesions to be treated with the CyberKnife.5 The spine is located in the imaging system’s coordinating frame in a manner analogous to that used to locate intracranial targets; by comparing the DRRs from the treatment planning study to the current spine location acquired via real-time images, the CyberKnife is able to determine the current position of the patient’s spine. The robotic arm adjusts the linac, and treatment doses are administered.
Precision of CyberKnife Treatment Planning
The principal innovation of the CyberKnife is the use of radiographic images of internal anatomical features to align the treatment beam with the target volume.
To achieve this, the position of a patient’s anatomy within the camera field of view must be communicated to the robot. By establishing a fixed reference point in both the robot and the camera coordinate frames, the two systems can be aligned with a precision of approximately ± 0.5 mm along each axis.6
However, eliminating the need for a stereotactic frame inevitably raises issues about the spatial accuracy of dose placement when compared with existing frame-based systems. The requirement of CT or CT/MR coregistration for the generation of DRRs introduces a level of uncertainty in the treatment planning of the CyberKnife. Standard CyberKnife treatment planning CT slice thickness is 1.25 mm, resulting in the uncertainty in the inferior/superior coordinate of the treatment volume of ~0.63 mm. Furthermore, radiographic technical limitations—edge softening from attenuation of the diagnostic x-rays, blurring from the reconstruction technique, and other ambiguities in the delineation of structure within an image—introduce an uncertainty of ~0.5 to 1.0 mm in the other two planning coordinates. These errors introduced by imaging are comparable to the imaging errors in frame-based systems.7
A second component of total clinical error is that introduced by the robot itself (mechanical accuracy of the robot). The linac beam is pointed by the robotic arm at an isocenter from ~75 to 150 different beam positions. The individual beams miss the theoretical targets with errors that are randomly distributed around a zero mean for each coordinate axis, with a net root mean square (rms) radial error of 0.7 mm.8 For a treatment that uses all beams, the effect of this source of error is to blur rather than offset the dose distribution. The rms radial pointing error of the CyberKnife is comparable to the deviation in the arc motion of a linac moving along a gantry path, which has been reported in one instance to be ≤ 0.6 mm.9
Another variable affecting dose precision is patient movement during frameless radiosurgery. In the present CyberKnife system, patient position is measured at select intervals prior to delivering each dose. Any movement during radiation delivery itself will lead to misdirection of that portion of the dose. The patient’s changed position is detected and compensated for at the beginning of the next cycle to preserve the pattern in which the beams traverse patient anatomy and intersect within the target. Given that there are typically over 100 nodes, a single patient movement affects no more than about 1% of the total administered dose in a single-fraction treatment. Furthermore, rotational changes in the patient’s position can be minimized by using head restraints, such as a molded AquaPlast mask (WFR/Aquaplast Corp., Wyckoff, NJ), during cranial treatments or a custom alpha cradle mold (Smithers Medical Products, Inc., Akron, OH) during spinal treatments. The noninvasive restraints also serve to confine the treatment site to a small region (± 10 mm) within which the imaging system is calibrated to measure translations. More recent advancements in image registration allow for measurement of all six degrees of freedom in the position and orientation of patient anatomy, thus compensating for rotational changes by the patient during the procedure.
CyberKnife Treatment Protocol
The initial steps of patient treatment consist of treatment imaging. Thin-slice CT and/or MR imaging is obtained and loaded onto the treatment planning computer. The treatment planning system (TPS) of the CyberKnife is designed to allow planning based on the geometry of the lesion and surrounding structures, the maximum number of allowable beams, and the dose distribution. Geometry is outlined on CT or MR images, and the amount of radiation each structure can tolerate is specified. A three-dimensional map of the lesion is created using contour data. The system then organizes beams in a manner that allows equal coverage of the tumor, with beams originating from different orientations and nodes. To accommodate dose constraints due to critical structures near the lesion, dose weighting is calculated for different beams. If initial beam configuration does not satisfy dose constraints, information from optimization will aid selection of a new beam; this process continues until a favorable set of beams and doses is obtained. This flexibility enables critical structures adjacent to the lesion to be spared and optimally directs radiation for irregularly shaped tumors. This is accomplished by exploiting the robot’s six-degree-of-freedom maneuverability to deliver a homogeneous dose that closely conforms to the irregular volumes.10,11 Once the inverse treatment planning is complete, the final plan is then reviewed by the treating team prior to delivery. During the actual treatment, the CyberKnife treatment algorithm places the linac at a determined position, uses real-time imaging to locate the target and adjust for movements, delivers radiation, and repeats the process at various nodes surrounding the patient.
CyberKnife Results
Benign Brain Lesions
Acoustic Neuromas
Acoustic neuroma control rates with CyberKnife radiosurgery are comparable to frame-based radiosurgery series, with tumor control rates above 98% and facial and trigeminal nerve complication rates of 1% or less.12 Multisession radiosurgery can be performed for acoustic neuromas (Fig. 4.2
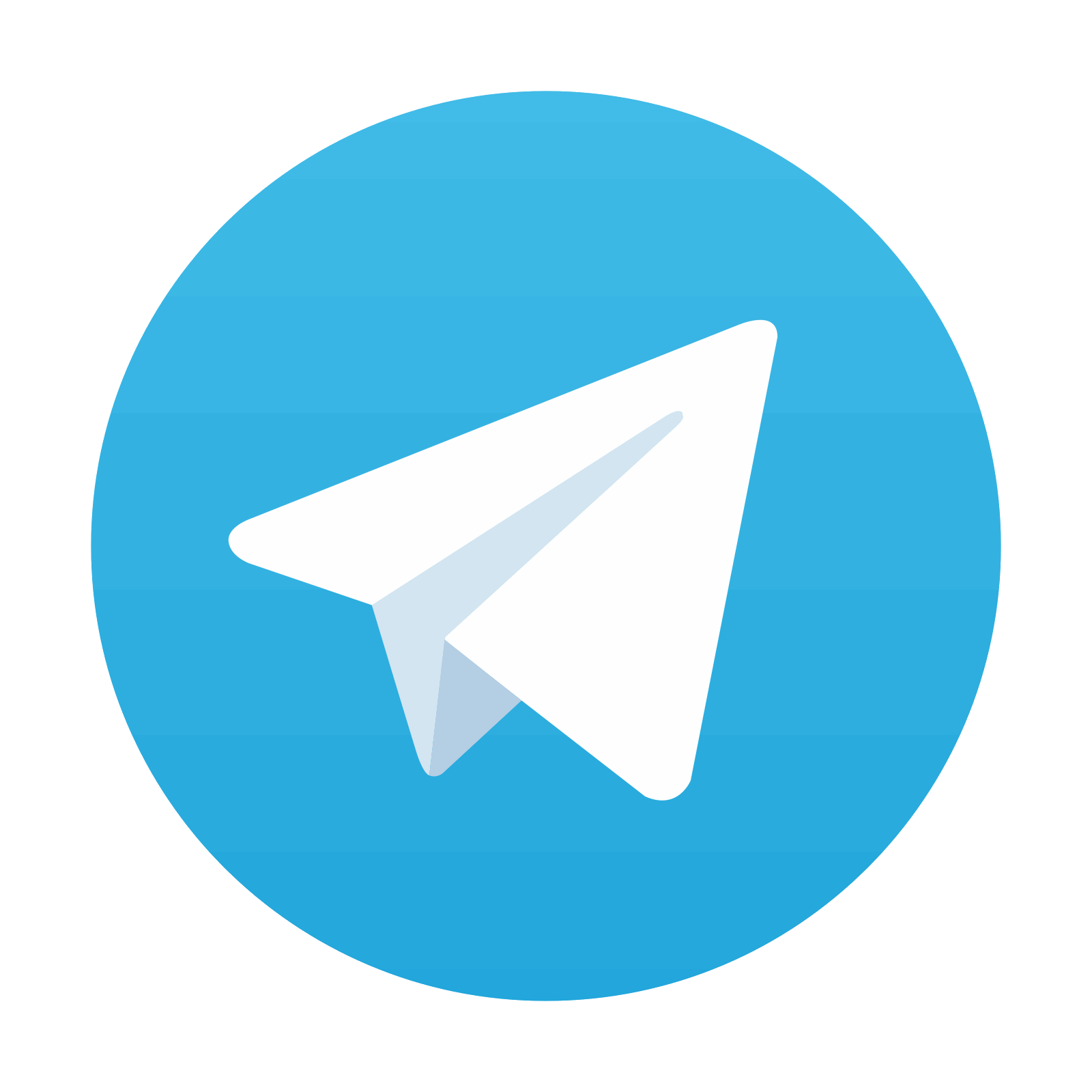
Stay updated, free articles. Join our Telegram channel

Full access? Get Clinical Tree
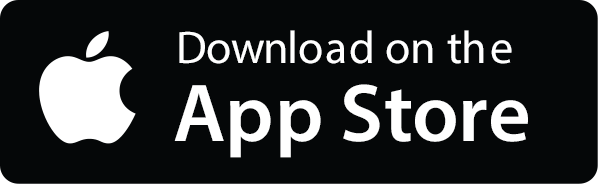
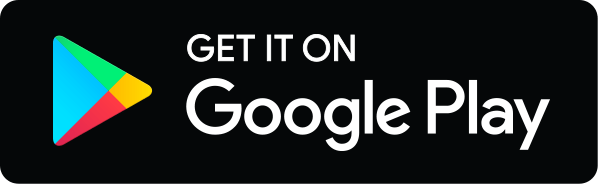