5 Imaging is a critical component for spine radiosurgery. It has a substantial impact on each step of the radiosurgical process. This chapter will describe the clinical roles of imaging in diagnosis, simulation, planning, localization, delivery, and assessment of spine radiosurgery. Vertebral body metastases occur in up to 40% of patients suffering from malignant cancer, and as many as one quarter of these will develop epidural spinal cord compression.1 Although the most common tumors metastasizing to the spine are lung, breast, prostate, and renal cell carcinomas, almost any tumor can present with spinal metastases.2,3 The thoracic spine is the most common site of epidural metastases, with 60 to 80% of disease occurring in this area. However, up to half of patients have disease in two or more regions. In the overwhelming majority of patients with vertebral body metastases and/or spinal cord compression, back pain is the first symptom.2–4 At diagnosis, other common symptoms of spinal cord compression (in decreasing frequency) are weakness, autonomic dysfunction, and sensory deficits.4 These symptoms are almost always accompanied by back pain.1–4 In general, magnetic resonance imaging (MRI) is the imaging modality of choice for diagnosing metastatic disease to the spine1,5–8 because it provides superior definition of the extent of disease radially and axially, can help distinguish malignant from benign disease,9,10 and is noninvasive. MRI has a specificity of 97 to 98%,6,10 a sensitivity of 93%,10 and a positive predictive value of 98%.6 In a prospective study of 280 patients with suspected malignant spinal cord compression, Husband et al6 found that 211 (75%) had epidural spinal cord or thecal sac compression. Of the patients with spinal cord compression, 25% had compression at two or more levels, and 69% of these involved more than one region of the spine. A paraspinal mass was found in 28% of patients, and only a third of these masses were detected on plain films. When radiotherapy fields were planned solely on the basis of plain films and neurological findings in 91 patients, the use of the subsequent MR images resulted in changes in 48 (53%) of these plans. A clinically significant change—primarily lengthening and/or widening of the field to include disease untreated on the initial “non-MRI” plan—was observed in 19 cases (21%). Although a staging bone scan may provide an initial indication of the sites of spinal metastases, it is far less sensitive than MRI in detecting small or intermedullary lesions.11 Computed tomography (CT) scans with bone and soft tissue windowing may suggest the presence of paraspinal, spinal, and epidural disease, but they are inferior to MRI in diagnosing the precise location of metastases. However, a CT scan remains essential to treatment planning and, in many cases, quality control. Although 18F-FDG (18F-2-deoxy-2-fluoro-D-glucose) positron emission tomography (PET)/CT may offer significantly better specificity than CT scanning alone in the diagnosis of malignant disease in the spine,12 it lacks the spatial resolution of MRI and is not (yet) an established tool for treatment planning. PET also lacks sensitivity in detecting blastic lesions arising from prostate and breast cancer.13,14 Whereas diagnosis is often, unfortunately, delayed, prompt treatment of malignant spinal cord compression is essential to optimizing outcome.15–21 In a prospective trial of patients with malignant spinal cord compression, Turner et al20 found that palliative radiation therapy, delivered via a posteroanterior (PA) field to the involved spine of ambulatory patients, allowed over 80% to remain ambulatory postradiation therapy. However, only 2 of 16 nonambulatory patients became ambulatory postradiation therapy. Pain was significantly reduced in 22 of 30 patients (73%). In a similar prospective trial, Maranzano and Latini17 found that “early diagnosis and treatment” (i.e., detection and radiation therapy of spinal cord compression prior to the onset of major neurologic deficits) was a significantly favorable predictor for the retention/recovery of the ability to walk. Complete or partial pain relief was observed in 54 (17%) of the patients undergoing radiation therapy. In a prospective trial of 139 male veterans with epidural compression who were treated with dexamethasone and radiotherapy, Zaidat and Ruff21 found that starting radiation within 12 hours of the loss of ambulation was 6.86 times more likely to restore ambulation than if treatment began after 12 hours (p <.001). Somewhat paradoxically, Rades et al19 noted that the functional outcome following radiotherapy for malignant spinal cord compression is significantly better in patients with a slow onset of motor deficits. CT, MR, and often PET imaging are used for treatment planning. CT is typically used as the gold standard for geometric target localization, along with soft tissue information provided by MRI. PET images are also used to provide supplemental functional information of the detected tumors. Subsequent to immobilization, simulation images are acquired to localize the target and critical organs/structures for radiation treatment planning. Three-dimensional (3D) CT imaging is the preferred modality for radiation treatment simulation, as it provides accurate patient geometric information and electron density information for heterogeneity correction during the monitor unit calculation. The drawback of using CT for simulation is its relatively poor soft tissue contrast compared with MRI. Iodinebased contrast agents are typically used to improve the image contrast. After a treatment plan is generated, a set of digitally reconstructed radiographs (DRRs) are typically calculated in two orthogonal directions. The DRRs are used as references to check the treatment isocenter against the treatment portal images. The ideal slice thickness should be no more than 3 mm because a thicker slice tends to degrade the quality of the DRR images and decreases the localization accuracy. Figure 5.1 T1-weighted magnetic resonance imaging (MRI) (a,c,e) and the corresponding computed tomography (CT) images (b,d,f). The fusion result can be checked with the MRI-CT hybrid images (g). Beam orientation of an intensity-modulated radiation therapy plan for spine radiosurgery with three-dimensional rendering is shown in h. MR images can usually provide good soft tissue contrast, and thus are useful for delineating the tumor target. T1-weighted MR images are commonly used for spine radiosurgery.22–25 Because MR images lack information about electron density and may suffer geometric distortion, they are usually registered to the simulation CT images for treatment planning. The soft tissue information in the MRI is fused to the CT to confirm target delineation. However, dose calculations are still performed based on the simulation CT images. Figure 5.1 shows T1-weighted MR images on the left and the corresponding CT images on the right. Both MR and CT images are shown in three orthogonal orientations—axial, coronal, and sagittal views. After the MR and CT images are registered, the fusion result can be checked with the MR-CT hybrid images (Fig. 5.1g). Because tumors being treated with radiosurgery are usually small, high spatial resolution is required for MR images. The resolution is usually limited by slice thickness. A slice thickness of 1 mm should be used for small tumors. Sometimes, molecular imaging using PET images is used to help confirm the malignancy of an identified lesion. Registration of PET images with either CT or MR images is rather challenging due to different imaging information presented in these images. The use of PET/CT modality would enhance this correlation between CT and PET images because both types of images are acquired when the imaging object is at the same position. As described in the previous section, the sensitivity and specificity are not always 100%, and biopsy remains the only definitive diagnostic tool. Figure 5.2 Isodose distributions in axial (a), coronal (b), and sagittal (c) views, and cone-beam computed tomography-guided target verification (d-f) for a spine radiosurgery case. After 3D images are acquired and imported into a treatment planning system and the image registration is completed (if multimodality images are used), the target and critical organ volumes are then delineated based on CT, MRI, and/or other imaging modalities, such as PET. Either 3D conformal (both static and arc) or intensity-modulated radiation therapy (IMRT) beams can be used to deliver a highly conformal dose to the target.26–33 For stationary conformal plans, a large number of beams should be used to conform the radiation to the target and achieve a rapid dose fall-off. Each beam can be conformed using a multileaf collimator (MLC) or other beam-shaping materials. For conformal arc beams, the radiation dose is delivered while the gantry rotates around the isocenter. When MLC is used on arc treatment, it can dynamically reshape the field to conform the beam to the target at each gantry angle. This technique is effective in conforming the geometry to the target, but it is not optimal for critical organ sparing. Compared with other techniques, IMRT can be used to deliver the radiation dose more precisely to the target while sparing critical organs. IMRT beams are modulated according to fluence maps, which are usually obtained through an inverse-planning process. During treatment delivery, the modulated beams are delivered according to an intensity map by moving the MLC either in a sliding-window mode or in a step-and-shoot mode. Figure 5.1h shows an example of an IMRT plan for spine radiosurgery using an Eclipse (Varian Medical Systems Inc., Palo Alto, California) treatment planning system with 120-leaf MLC. Nine IMRT beams are used to deliver the dose to the target volume. The dose to the spinal cord is minimized with IMRT. The corresponding isodose distributions are shown in Fig. 5.2. Target localization is a critical process in radiosurgery for spinal tumors. It is essential to precisely deliver the planned radiation dose to the target. Any deviation in localization accuracy could lead to an unexpected deviation from the treatment intent. Radiographic imaging has proven to be one of the most effective methods for localization of spinal tumors. Recent developments in onboard imaging technology have substantially improved this verification technique. Conventional film imaging has been largely replaced by more efficient two-dimensional (2D) digital imaging, as well as more accurate 3D cone-beam computed tomography (CBCT). There are two approaches to acquire 2D radiographic images for target localization: megavoltage (MV) imaging using treatment beams and kilovoltage (kV) imaging using localization beams. Both are typically used for isocenter localization. Electronic portal imagers are commercially available that use MV beam in real time for target localization. This verification method has become the norm in many radiation therapy centers. However, some concerns with this technology remain to be addressed, including poor soft tissue contrast, high radiation dose from using MV beams, and the fact that MV portal images are not exactly congruent to simulation images because of the different physical principles of image formation. The desire to use a kV x-ray beam for patient positioning and target localization is becoming stronger as daily imaging is increasingly used for patient setup correction and target localization. Although several approaches were developed decades ago, clinical implementation of kV imaging has become commercially available only within the last 5 years. Whereas MV portal imaging uses the treatment beam, kV imaging requires an additional x-ray source for imaging. Various system configurations have been developed for in-room kV imaging for treatment target localization.31
Imaging and Target Localization
Imaging for Diagnosis
Imaging for Planning
CT Imaging
MR Imaging
Molecular Imaging
Planning Techniques
Imaging for Localization
2D Radiography
Stay updated, free articles. Join our Telegram channel

Full access? Get Clinical Tree
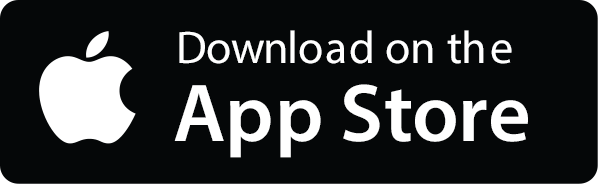
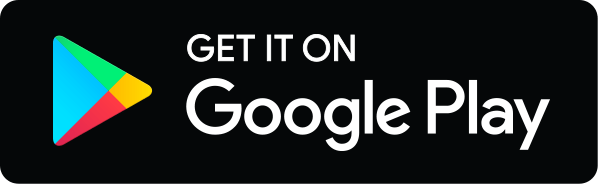