Fig. 1 a–d
Normal magnetic resonance (MR) appearance following meniscal repair. Sagittal fast spin echo intermediate weighted (a) and T2-weighted fat-suppressed (b) images through the medial meniscus illustrate a clearly defined vertical tear (arrow, a, b) extending through the posterior horn of the medial meniscus. c Corresponding position matched MR imaging performed 5 years following meniscal repair shows persistent increased intrasubstance signal changes related to the healed meniscal tear site (arrow). d Importantly, no increased fluid signal intensity is seen on the corresponding T2- weighted fat-suppressed image correlating to the normal appearance of a successfully healed meniscal repair
The accuracy of MRI in diagnosing meniscal tears following meniscal surgery appears to be related to the amount of meniscal tissue that has been resected. In menisci where there has been resection of <25% of the meniscus, MRI demonstrates accuracy rates of 89–100% utilizing the traditional criteria of grade III signal on short TE sequences [9]. In menisci where >25% of the meniscus has been resected, the presence of grade III signal on short TE sequences is of limited diagnostic utility. Accuracy rates of 50–65% have been recorded for conventional MRI depending on the degree/extent of prior meniscal resection, the lower figure representing the accuracy in those with >75% of the meniscus resected [9]. Similarly, contour abnormality is of limited accuracy in the diagnosis of a meniscal tear in patients with extensive partial meniscectomy. In one study, all patients who demonstrated marked contour irregularity after resection of more than a third of the meniscus had a negative repeat arthroscopy, while in another study using alteration of meniscal morphology beyond what would be expected following meniscal surgery as the only criteria for a recurrent tear, an accuracy rate of only 67–68% was achieved [10]. Traditional diagnostic criteria of a meniscal tear can still be applied for diagnosis of tears in portions of the meniscus where surgery has not been performed, but this is dependent on access to prior surgical reports and preoperative imaging.
Therefore, it is clear that diagnostic criteria for accurate assessment of meniscal tearing need to be modified following meniscal surgery. The suggested modified criteria for diagnosis of a meniscal tear in a postoperative meniscus include an area of fluid signal intensity extending into the substance of the meniscus on T2-weighted images, and identification of a displaced meniscal fragment or meniscal fragmentation [7]. As identification of fluid signal intensity is critical to diagnosing a recurrent tear, it is worthwhile to obtain T2-weighted images in both the sagittal and the coronal planes. The addition of fat saturation increases the conspicuity of the fluid signal and can be helpful. Indeed, in the group of patients with resection of >75% of the meniscus, the meniscal remnant may be hard to visualize on proton density images and is best seen on T2-weighted images, particularly in the presence of adjacent fluid. Radial tears in particular appear to be more common in the postoperative setting in comparison with virgin menisci, and this is likely related to altered biomechanical properties of a partially resected meniscus [11]. Another potentially useful sign of recurrent or residual meniscal tearing following meniscal repair is widening of the tear repair cleft on serial examinations [8]. In patients with prior meniscal repair, identification of surfacing high T2 signal within a meniscus has a specificity of 88–92% and a sensitivity of 41–69% [7, 9]. However, in applying these diagnostic criteria in the diagnosis of a recurrent or residual meniscal tear, it must be remembered that fluid signal may be seen within a healing cleft without signifying a residual tear within the first 12 weeks following meniscal repair [7].
Identification of fluid signal within a tear is likely to be related to imbibition of joint fluid into a tear gap. In an attempt to utilize this mechanism, direct MR arthro — graphy has been used for evaluation of recurrent or residual meniscal tears. Direct MR arthrography has potential advantages in imaging of a postoperative meniscus through bathing the meniscus completely in a diluted gadolinium mixture and increasing intra-articular pressure by distending the joint, and then the tear cleft is theoretically more likely to be outlined. In addition, T1- weighted imaging, which is typically performed at MR arthrography, has a higher signal-to-noise ratio than T2- weighted acquisitions and may be advantageous for detection of tear clefts. Direct MR arthrography is most commonly performed under fluoroscopic guidance whereby intra-articular position of the needle is confirmed by injection of a small quantity of iodinated contrast, followed by injection of a 20–30 mL gadolinium mixture with a 1:100 to 1:250 dilution. Gentle exercise following the injection can further assist in imbibition of the gadolinium mixture into a potential tear.
Meniscal tears at MR arthrography are diagnosed on the basis of a cleft of similar signal intensity as intraarticular gadolinium. Several studies directly comparing conventional MRI and MR arthrography in each patient have demonstrated a higher accuracy rate with MR arthrography in patients with previous meniscal surgery [9, 12, 13]. Applegate et al. demonstrated an overall accuracy of 66% for conventional MRI and 88% for MR arthrography [9]. Sciulli et al. obtained similar results, with accuracy rates of 77% and 92%, respectively, for conventional MRI and MR arthrography [12]. MR arthrography does not appear to have an advantage over conventional MRI for detection of recurrent tears in the subset of patients where <25% of the meniscus has been resected, with an accuracy of 89% for both techniques [9, 13]. In another study, whereby patients were randomized to either conventional MRI or MR arthrography, there were no statistically significant differences between the two techniques, with conventional MRI demonstrating a sensitivity of 86% and a specificity of 67%, and MR arthrography 89% and 78%, respectively, in the diagnosis of a recurrent or residual meniscal tear [14]. Diagnostic difficulties with direct MR arthrography can still be encountered because of residual increased intrasubstance signal changes seen within a healing or healed meniscal tear on short TE acquisitions, such as T1- weighted sequences traditionally employed at MR arthrography. Volume averaging of signal can occur across residual tear clefts utilizing traditional 2D MR acquisitions with image slice thicknesses of 3–4 mm. Such acquisitions may average voxel signal related to intrameniscal contrast or fluid with adjacent meniscal tissues, resulting in intermediate signal characteristics and diagnostic difficulties at evaluation.
CT arthrography has been utilized for detection of meniscal tears in virgin menisci with a reported sensitivity and specificity of over 90% [15]. CT arthrography has a sensitivity of 100% but a specificity of only 78% in detection of recurrent or residual meniscal tears [15]. The lower specificity is likely to be related to the high spatial resolution of CT arthrography identifying small foci of intrameniscal contrast that may represent stable partialthickness meniscal tears. Using the criteria of contrast extending throughout the height or depth of the meniscus for a full thickness tear or at least a third of the height or depth of the meniscus for diagnosis of an unstable partial- thickness tear, the specificity of CT arthrography improves to 89%, but with a lower sensitivity of 93% [16].
In the setting of a young patient without pre-existent cartilage loss and an irrepairable extensive meniscal tear resulting in meniscal deficiency post meniscal resection, meniscal transplantation may be performed. MRI plays an important role in the preoperative assessment of potential transplant candidates, providing important information regarding the status of articular cartilage and ligamentous integrity of the joint, and serving as an accurate measure of meniscal transplant size matching requirements [17]. MRI can be used for evaluation of possible complications following meniscal transplantation. Few long-term followup MR imaging studies of meniscal transplants have been performed to date. The presence of meniscal transplant tears, healing of meniscal bone plugs, status of the menisco — capsular junction, and extrusion of the meniscus and chondral changes can be evaluated, but there appears to be poor correlation between MRI findings and clinical symptoms [17]. A degree of peripheral displacement of the body of a meniscal transplant can be seen in up to 46–72% of patients post meniscal transplantation [17]. However, frank meniscal transplant extrusion is less commonly seen and is more likely to be associated with symptoms. Additional MRI findings described suggestive of transplant failure include meniscal fragmentation, and progressive adjacent articular cartilage loss [17].
Imaging of Postoperative Ligaments
Anterior Cruciate Ligament
The anterior cruciate ligament (ACL) is by far the most frequently completely torn ligament of the knee. Treatment and reconstruction techniques of ligamentous injuries of the knee have improved significantly over the past two decades. Treatment of ACL injury is typically tailored to the patient’s lifestyle and age, and the presence or absence of other associated injuries of the joint. In older patients, conservative treatment may be an option, with physiotherapy and muscle training used in attempts to minimize the degree of joint instability and thus avoid or sufficiently delay the early onset of arthritis. In people used to sporting activities or in professional athletes, operative treatment is advocated in order to prevent ongoing joint instability and complications of ACL deficiency, including further ligamentous damage, cartilage loss and meniscal tearing. Primary healing capacity of the ACL after tearing is limited, and primary repair of complete ACL tears without using augmentation grafts has generally been unsuccessful to date. Therefore, primary ACL reconstruction utilizing autologous graft material, allowing for early restoration of joint function, has become the most widely employed method of surgical management of the ACL deficient knee.
Several techniques of ACL reconstruction have been described. Currently, the mainstay of modern surgical re Several techniques of ACL reconstruction have been described. Currently, the mainstay of modern surgical reconstruction is a single bundle reconstruction to recapitulate the anatomy and function of the anteromedial band of the native ACL. Recommended graft choices for such ACL reconstruction consist of either biologic autograft or allograft material [18]. Reconstruction of the ACL with prosthetic material has met with only limited degrees of success. The patellar tendon autograft is the method used in most orthopedic centers. The hamstring tendon graft has increased in popularity over the past several years, primarily as a result of improved fixation techniques [18]. No significant difference in long-term success between these two different methods of graft reconstruction has been found. However, advantages of hamstring graft ACL reconstruction include a small incision at the harvest site, as well as a decreased incidence of anterior knee symptoms following surgery. Bone-patellar tendon-bone grafts are commonly utilized in young active athletes because high-quality fixation of the graft allows for the earliest possible return to activity [18]. With this method, the middle third of the patellar tendon with bone plugs from the inferior pole of the patella and the tibial tuberosity are harvested. The major disadvantage of bone-patellar tendon- bone graft ACL reconstruction is a comparatively high incidence of symptoms at the harvest site, including anterior knee pain, postoperative arthrofibrosis, patellar tendon rupture, and even patellar fracture in rare cases. On MRI, following graft harvest the patellar tendon is initially thickened, generally illustrating intrasubstance increased T1- and intermediate T2- weighted signal. By about 18 months to 2 years after surgery, the gap in the patellar tendon becomes filled with granulation and scar tissue, demonstrating MRI signal almost identical to that of the original patellar tendon.
During the first couple of months after surgery, it has been have shown that the inserted graft is avascular, illustrating low MR signal intensity on all pulse sequences similar to that of the normal patellar tendon [19]. Within the first 3 months after ACL reconstruction, proliferating synovial tissue envelops the graft and provides its vascular supply. At approximately 6 months following surgery, however, the graft undergoes a remodeling process, during which time the graft experiences revascularization and resynovialization. The process of gradual transformation of the patellar or hamstring tendon graft into tissue very similar to the native ACL is referred to as graft „ligamentization”. The strength of the graft is decreased during the period of revascularization and this results in its vulnerability to reinjury during this time period. Normally by 12–24 months the resynovialization process is finished [19]. Persistent intrasubstance graft signal following this was previously hypothesized to be reflective of complications such as possible graft impingement or partial tearing. However, more recently published literature has shown that variable degrees of mild increased intrasubstance graft signal is in fact seen in the majority of patients post ACL reconstruction, with stable joints at biomechanical testing, and excellent
Hamstring tendon grafts are derived from resection of the distal segments of the semimembranosus and gracilis tendons. The tendons are harvested from the level of their tibial attachment to the level of the musculotendinous junction. The two tendons are sutured together, doubled back onto one another, and then sutured together again, resulting in a graft composed of four separate bundles. MR signal intensities of the hamstring graft are almost identical to the patellar tendon graft. However, because the hamstring graft is composed of four separate bundles sutured together, MR imaging often demonstrates linear areas of intermediate signal interposed between the separate bundles of the graft. Important clinical considerations for achieving optimal results of ACL reconstruction include isometric graft positioning, avoidance of graft impingement, proper tensioning and fixation of the graft, and appropriate postoperative rehabilitation. The position of the femoral tunnel is critical in obtaining graft isometry. Graft tunnel location is chosen to achieve graft isometry by restoring normal anatomic alignment, and thus limit potential stretching or impingement of the graft, as well as overconstraint of the knee. The position of the femoral tunnel is typically placed at the intersection of the posterior femoral cortex and the posterior physeal scar corresponding to the midpoint in the anatomic origin of the anteromedial and posterolateral bands of the native ACL. The position of the tibial tunnel is typically chosen to correspond to the tibial insertion of the anteromedial band of the native ACL, such that the graft is oriented parallel but posterior to the slope of the intercondylar roof (Blumensaat’s line), with the knee in a fully extended position, such as is well depicted on sagittal MR images. An anteriorly located femoral bone tunnel will cause elongation of the graft and result in instability of the knee. Posterior positioning of the tibial tunnel results in too steep a course of the ACL reconstruction, and potential residual ACL instability post reconstruction. In contrast, roof impingement occurs when the tibial tunnel is placed too far anteriorly.
Recent modifications in single bundle ACL surgical technique to address possible issues of posterolateral rotational instability following ACL reconstruction have included lower positioning of the femoral graft tunnel, such that the graft is oriented in a less vertical fashion on coronal images. In general, an orientation of a single bundle ACL graft in the coronal plane should be such that the femoral tunnel and origin of the graft is at the approximate 2:30 position of a clock face, with the graft oriented in a plane less than 75 degrees relative to the tibial plateaus. An additional trend in ACL reconstructive surgery, performed in attempts to recapitulate the full biomechanical function of an ACL reconstruction, is the double bundle anatomic ACL reconstruction. With double bundle reconstructions, anatomic surgical reconstruction of the two native ACL functional (anteromedial, and posterolateral) bundles are performed.
Common clinical symptoms of complication following ACL reconstruction include recurrent ACL instability, limited knee joint extension postoperatively, or new symptoms of joint derangement. MRI plays an important role in the assessment of possible ACL repair complications. ACL grafts are most vulnerable to injury during the first several months following reconstruction. The remodeling process weakens the graft temporarily, but by the end of the first year the graft approaches the strength of the native ACL. Recurrent instability following ACL reconstruction may be seen in the setting of graft tearing, dislodgement or failure of graft fixation, or graft stretching in the setting of an intact graft at MRI, but findings or symptoms of instability at clinical examination. MRI is highly accurate for diagnosing complete ACL graft tears. Integrity of the graft fibers can be well evaluated on MRI, with complete discontinuity of graft fibers seen in the setting of complete graft tears (Fig. 2). However, partialthickness graft tears may also be seen with discontinuity of some, but not all, ACL graft fibers observed. Clinically, ACL graft tearing is classically associated with instability and history of re-injury of the knee. Another imaging feature that might accompany such clinical findings in the setting of intact graft fibers is dislodgement of a reconstruction graft secondary to fixation failure.
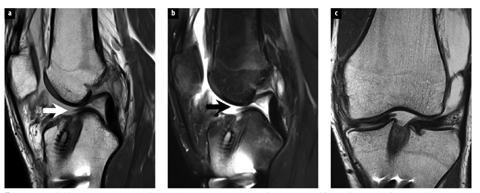
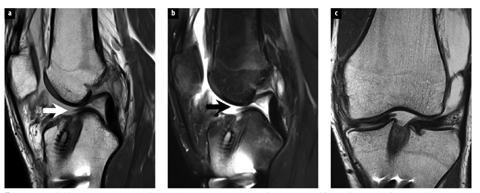
Fig. 2 a–c
Completely torn anterior cruciate ligament (ACL) reconstruction graft in a 30-year-old man who sustained an acute injury, 6 years following prior ACL reconstruction. Sagittal fast spin echo intermediate weighted (a), T2-weighted fat-suppressed (b), and coronal intermediate weighted (c) magnetic resonance images show complete discontinuity of ACL graft fibers (arrows) immediately adjacent to the tibial tunnel of the patients prior ACL reconstruction. Complete ACL graft tear was confirmed at the time of revision reconstruction surgery
Limited knee extension following ACL reconstruction is typically manifest by loss of the terminal 5–10 degrees of knee extension at clinical examination. One important cause of such complication, which is evaluated by MRI, is graft impingement. Graft impingement is typically observed with surgical tibial tunnel positioning partially or completely anterior to the projected slope of the intercondylar notch (Blumensaat’s line). In such instances, the distal half of the intercondylar roof mechanically impinges on the anterior surface of the graft during knee extension, leading to loss of terminal extension of the knee and the potential for progressive graft injury, fibrosis and possible rupture [19]. MRI allows direct visualization of the position of the tibial tunnel and its relationship to Blumensaat’s line, as well as the course of the ACL graft and its relationship to the intercondylar roof. The impinged graft demonstrates focal increased signal on T1- and T2-weighted images in the distal two-thirds of the graft fibers. Notchplasty (a surgical procedure that consists of resection of a few millimeters of bone from the anterior outlet of the roof and the lateral wall of the intercondylar notch) can be performed to treat cases of mild graft impingement. Signal intensity changes seen on MR images of impinged grafts usually resolve within several weeks following notchplasty.
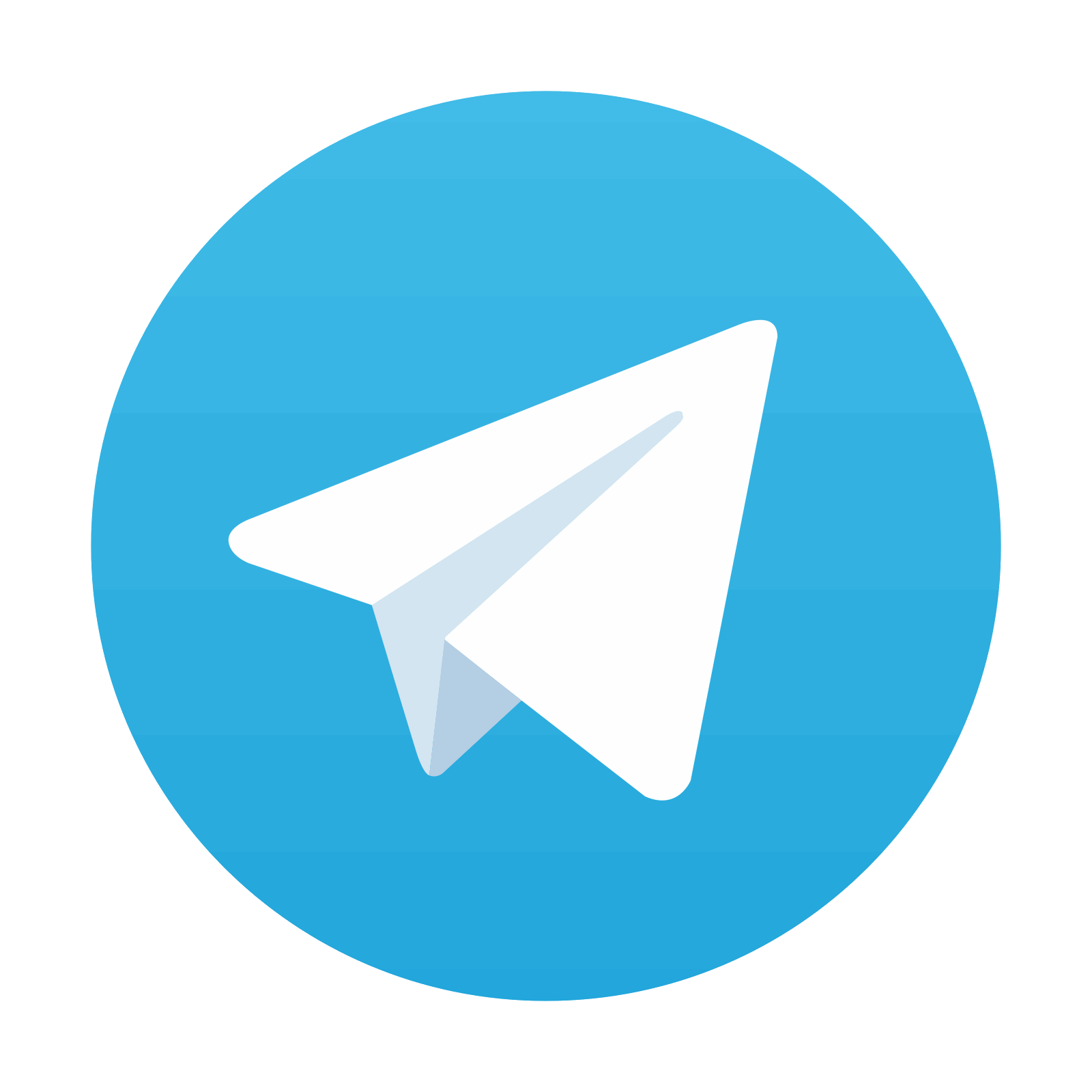
Stay updated, free articles. Join our Telegram channel

Full access? Get Clinical Tree
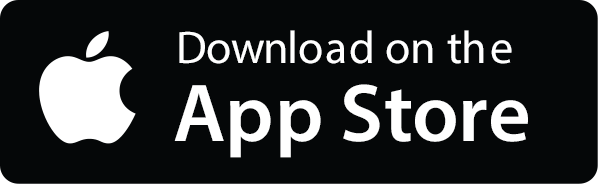
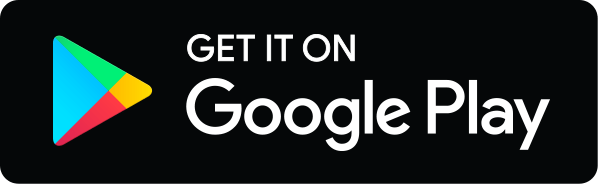