© Springer Science+Business Media New York 2014
Sudhakar K. Venkatesh and Richard L. Ehman (eds.)Magnetic Resonance Elastography10.1007/978-1-4939-1575-0_66. Impact of Magnetic Resonance Elastography on Liver Diseases: Clinical Perspectives
(1)
Baylor University Medical Center, 3410 Worth Street, Suite 860, Dallas, TX 75246, USA
(2)
Division of Gastroenterology and Hepatology, Mayo Clinic, 200 First Street SW, Rochester, MN 55905, USA
Introduction
Burden of Chronic Liver Disease
Chronic liver disease and cirrhosis remains a major public health problem worldwide. In the United States alone, an estimated 150,000 persons annually are diagnosed with chronic liver disease with nearly 30,000 (20 %) individuals having cirrhosis at initial presentation. Furthermore, over 75 % of individuals are likely to be asymptomatic from their liver disease at diagnosis [1]. These trends are expected to increase based on an aging population and the growing epidemic of obesity [2, 3].
Current diagnostic tests used within clinical practice are not sensitive or specific enough to function as screening tests for more invasive, definitive procedures such as liver biopsy. Although liver biopsy has been considered the gold standard for detecting hepatic fibrosis, its use in clinical practice appears to be declining over time. The availability of laboratory assays to identify liver diseases such as chronic hepatitis C is a major reason for this phenomenon. Additionally, the ability to perform liver biopsy in large populations are limited by the risk for procedure-related complications, patient acceptance, cost, and inaccuracies associated with sampling error [4, 5]. In turn, there remains a need to introduce novel, effective methods to diagnose and risk stratify individuals with chronic liver disease.
Contemporary Issues with Liver Biopsy
Liver histology classification systems recognize five stages of fibrosis, defined as F0 (no fibrosis), F1 (portal fibrosis), F2 (periportal fibrosis), F3 (bridging fibrosis), and F4 (cirrhosis). Clinically significant hepatic fibrosis is defined by the presence of periportal fibrosis (stage F2) or greater on liver histology. While measurement error with liver biopsy has been an acknowledged limitation for decades, recent studies have systematically demonstrated that a minimum liver tissue size (≥20 mm) and portal tract number (≥10) are needed to maximize the accuracy of biopsy [4, 5]. In clinical practice, however, the average liver tissue size and portal tract number are often less than this threshold [6]. Hence, the ability to make reliable and valid fibrosis staging assessments remains inexact for a significant number of patients.
Elastography Imaging for Detecting Hepatic Fibrosis
Given the practical limitations of using liver biopsy among the growing number of individuals with chronic liver disease, a number of noninvasive methods to detect liver fibrosis have been developed over the past decade. Furthermore, the ability to characterize fibrosis extent by categories as opposed to discrete stages has gained importance as more effective therapies for chronic liver disease become available. In general, these methods have been characterized as (1) serum tests containing panels of markers reflecting the biology of hepatic fibrogenesis and (2) functional imaging tests which measure unique physical characteristics associated with the development of fibrosis. Among the imaging studies to date, the most widely studied and used are elastography imaging techniques with ultrasound or magnetic resonance (MR) platforms [7].
Ultrasound-based transient elastography (TE) utilizes a transducer probe which emits low-frequency (50 Hz) vibrations into the liver when applied between the rib interspaces where biopsy would be performed. The propagating shear wave induced by these vibrations is then detected by a pulse-echo acquisition, and the velocity of the wave is then calculated. Shear wave velocity is measured in kiloPascals (kPa), and is proportional to liver stiffness represented by the equation for Young’s elastic modulus E (expressed as E = 3ρv 2, where v is the shear velocity and ρ is the density of tissue, assumed to be constant). The accurate measurement of liver stiffness by TE is represented by (1) the ratio of successful measurements to the total number of acquisitions being ≥60 % and (2) the interquartile range for measurements being within 30 % of the median value [8].
Magnetic resonance elastography (MRE) uses a modified phase-contrast imaging sequence to detect propagating shear waves within the tissue of interest. Acoustic shear waves are generated by a pneumatic driver placed directly over the upper abdomen for propagation into liver tissue. Subsequently, the liver stiffness values are calculated from wave displacement patterns and displayed as color-encoded images (elastograms). Region of interest analysis throughout four cross-sectional slices of liver (avoiding vascular structures) is then performed to calculate mean liver stiffness [9, 10]. Elasticity quantification by MRE is based on the formula representing shear modulus, which is equivalent to one third of the Young’s modulus which is used with TE [11].
Clinical Impact of Elastography Imaging on Liver Disease
A major goal within clinical practice is to identify patients who have developed clinically significant hepatic fibrosis or cirrhosis. Decisions regarding monitoring versus institution of disease-specific therapy are now dictated by the category of fibrosis involvement rather than its specific individual stage. For most noninvasive tests, the ability to categorize patients as having no to minimal fibrosis (F0–F1) or moderate to severe fibrosis (F2–F4) is now possible [7, 8]. Studies to date have shown that liver stiffness assessment by MRE and TE is highly effective at separating individual patients into these diagnostic categories. Liver stiffness as measured by MRE is highly reproducible with high interobserver agreement [12, 13]. Further it may also be feasible and highly accurate among the pediatric population, including those with obesity [14].
Detection of Clinically Significant Hepatic Fibrosis and Cirrhosis
The first clinical application of MRE has been the detection and characterization of hepatic fibrosis. In this diagnostic role, MRE offers a safer and potentially more accurate alternative to invasive liver biopsy. Pilot and initial prospective studies have demonstrated the feasibility and diagnostic accuracy in detecting hepatic fibrosis among patients with known chronic liver disease [15–19]. MRE has been shown to be highly effective for distinguishing normal from fibrotic livers with a very high negative predictive value exceeding 97 %. Receiver operating characteristic (ROC) analysis has demonstrated that a liver stiffness value ≥2.9 kPa separates any degree of liver fibrosis from normal liver with a sensitivity and specificity of 98 and 99 %, respectively.
Conversely, MRE is highly accurate for the detection of cirrhosis with sensitivity and specificity values exceeding 90 %, respectively [16, 17]. For example, among persons with chronic hepatitis B, MRE was significantly more accurate than serum fibrosis markers for the detection of significant fibrosis (0.99 vs. 0.55–0.73) and cirrhosis (0.98 vs. 0.53–0.77). Sensitivity, specificity, positive predictive, and negative predictive values for MRE for cirrhosis were 97.4, 100, 100, and 96, and 100, 95.2, 91.3, and 100 %, respectively [20].
This compares favorably with TE where average sensitivity and specificity rates nearing 90 %, respectively, are also observed [21, 22]. The importance of identifying cirrhosis at its earliest stages is for detecting complications such as esophageal varices and hepatocellular carcinoma, both of which can benefit from early interventions.
Furthermore, MRE can discriminate between patients with clinically significant hepatic fibrosis (F2–F4) as compared to individuals with no to mild fibrosis (F0–F1) with sensitivity and specificity values in the 80–85 % range, respectively [10, 17]. Notably, these results are higher when compared to TE where sensitivity and specificity values between 70 and 80 %, respectively, have been reported in single and combined analyses [21, 22]
Recently, a large prospective study comparing MRE, TE, and the serum AST-to-platelet ratio index (APRI) in a series of 141 patients with chronic liver disease was performed [17]. In addition to a higher technical success rate for MRE vs. TE (94 vs. 84 %), the diagnostic accuracy of MRE was also superior to both TE and APRI. While comparable accuracy rates for detecting cirrhosis were observed for MRE and TE, the accuracy of MRE was significantly higher than TE for detecting clinically significant hepatic fibrosis (F2–F4).
The comparative study between MRE and TE highlights known inherent differences between these techniques in assessing hepatic fibrosis. While the reproducibility of TE is excellent in experienced centers, its accuracy is diminished by the presence of inexperienced operators and patient factors including obesity and narrow rib interspaces [23, 24]. This is based, in part, on the inability of TE to provide wave penetration beyond a distance of 6–7 cm which can represent the width of subcutaneous tissues in some obese patients [23, 24]. Thus, a major issue for TE is the prevalence and severity of obesity in developed countries, where the frequency of incomplete exam and complete technical failure rates may exceed 30 % [23, 24]. The reproducibility of MRE is also excellent [25]. In a recent study comparing MRE and shear wave elastography in the same individual, the rates of unreliable exam were higher with shear wave as compared to MRE (19 vs. 0 %) [26]. Furthermore, MRE is not significantly affected by obesity, rib interspace width, or ascites. While TE is designed to measure liver stiffness in a cylindrical-shaped area 1 cm wide and 4 cm long confined exclusively to the peripheral right liver, MRE calculates liver stiffness over the entire cross-sectional areas of hepatic parenchyma from multiple slices. Individuals with typical contraindications to MRI, however, are unable to undergo MRE [11, 24].
For both MRE and TE, it should also be noted that other pathophysiological processes including acute inflammation, cholestasis, portal pressure, and hepatic congestion may independently contribute to liver stiffness [27–31]. A recent study evaluated the role of MRE in assessment of liver fibrosis among patients with congenital heart disease after the Fontan procedure. In this preliminary study, the mean liver stiffness was elevated at levels associated with advanced fibrosis. However, it is unclear whether in this population the elevated stiffness is marker of increased passive congestion or a reflection of true fibrosis due to longstanding cardiac dysfunction [32]. This emphasizes the point that elevated liver stiffness may have different implications depending upon the population.
Detection of Clinically Significant Portal Hypertension
Complications associated with portal hypertension represent a significant proportion of morbidity and mortality among individuals with cirrhosis. The gold standard test for detecting portal hypertension involves measurement of the hepatic venous pressure gradient (HVPG) by transjugular hepatic venography. Numerous studies to date have identified an HVPG ≥ 10 mmHg as an independent predictor of developing complications of liver disease. Furthermore, a reduction in HVPG below 12 mmHg (or HVPG reduction by ≥20 %) with treatment after variceal bleeding is associated with improved survival [35]. However, the measurement of HVPG in clinical practice has not become widespread based on the technical demands, invasiveness, and risk for complications associated with this procedure [35, 36].
Given the ability of elastography imaging to detect cirrhosis, a number of subsequent investigations have demonstrated that liver stiffness is strongly correlated with HVPG values below 10 mmHg among individuals with chronic hepatitis C [37–39]. The correlation is less precise, however, beyond an HVPG value of 12 mmHg based on complex pathophysiologic changes associated with advanced portal hypertension such as increasing resistance to portal blood flow, porto-systemic collaterals, and splanchnic vasodilatation [40, 41]. The potential use of liver stiffness for detecting esophageal varices in patients has also been studied. However, these have been studies using TE which show that liver stiffness is an insensitive predictor for esophageal varices among individuals with cirrhosis [40, 42]. Similar results have also been observed with MRE [43]. At this point, it cannot be recommended that elastography imaging be used as a screening method to determine which patients with compensated cirrhosis should undergo endoscopic screening for esophageal varices. On the other hand, a recent study did show promise in the prediction of presence of large varices (as compared to small varices). In this single center study, a cutoff of 5.8 kPA had good sensitivity (96 %) and negative predictive value (98 %) for prediction of large varices though the specificity (60 %) and positive predictive value (36 %) was poor [44].
Given the relationship between splenomegaly and esophageal varices with cirrhosis, spleen stiffness as a noninvasive predictor of portal hypertension was recently measured using MRE [43]. In addition to demonstrating that spleen stiffness was significantly higher among patients with liver fibrosis compared to healthy controls, the spleen stiffness was increased further among patients with cirrhosis and porto-systemic collaterals versus patients with cirrhosis alone. Notably, a mean spleen stiffness ≥10.5 kPa was 100 % sensitive in identifying all 17 patients with esophageal varices and cirrhosis in the study cohort. Therefore, it is possible that spleen stiffness provides additional information about the hemodynamic alterations within splenic and splanchnic circulations not captured by liver stiffness. Based on these preliminary results, future studies including the measurement of HVPG as well as spleen and liver stiffness are being pursued.
Compensated and Decompensated Cirrhosis
Patients affected by compensated and decompensated (presence of variceal bleeding, ascites or hepatic encephalopathy) cirrhosis are known to have disparate clinical outcomes. As compared to the general population, individuals with compensated cirrhosis have a fivefold increase, whereas patients with decompensated disease have a tenfold increase in mortality [45]. Given that a majority of deaths in patients with compensated cirrhosis are due to progression to a decompensated state and the development of its ensuing complications, the ability to predict decompensation is important. If patients with compensated liver disease at the highest risk of decompensation can be identified, it may be possible to institute enhanced surveillance and prophylactic measures for this patient subset. The role of elastography in identifying this risk of transition has been reported. In a recent meta-analysis, the degree of liver stiffness as quantified by either TE or MRE was associated with increased risk of decompensated cirrhosis, HCC, and death [46
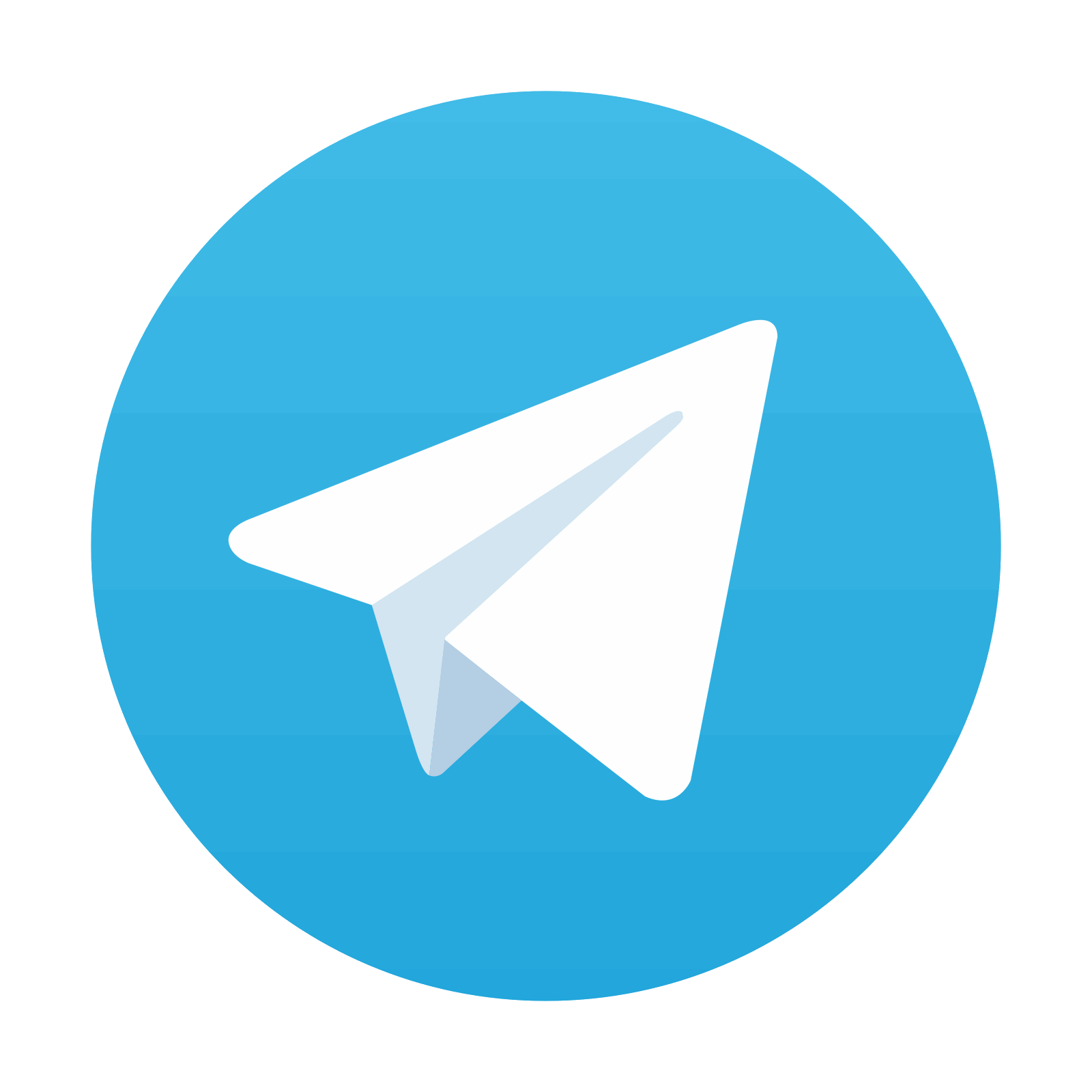
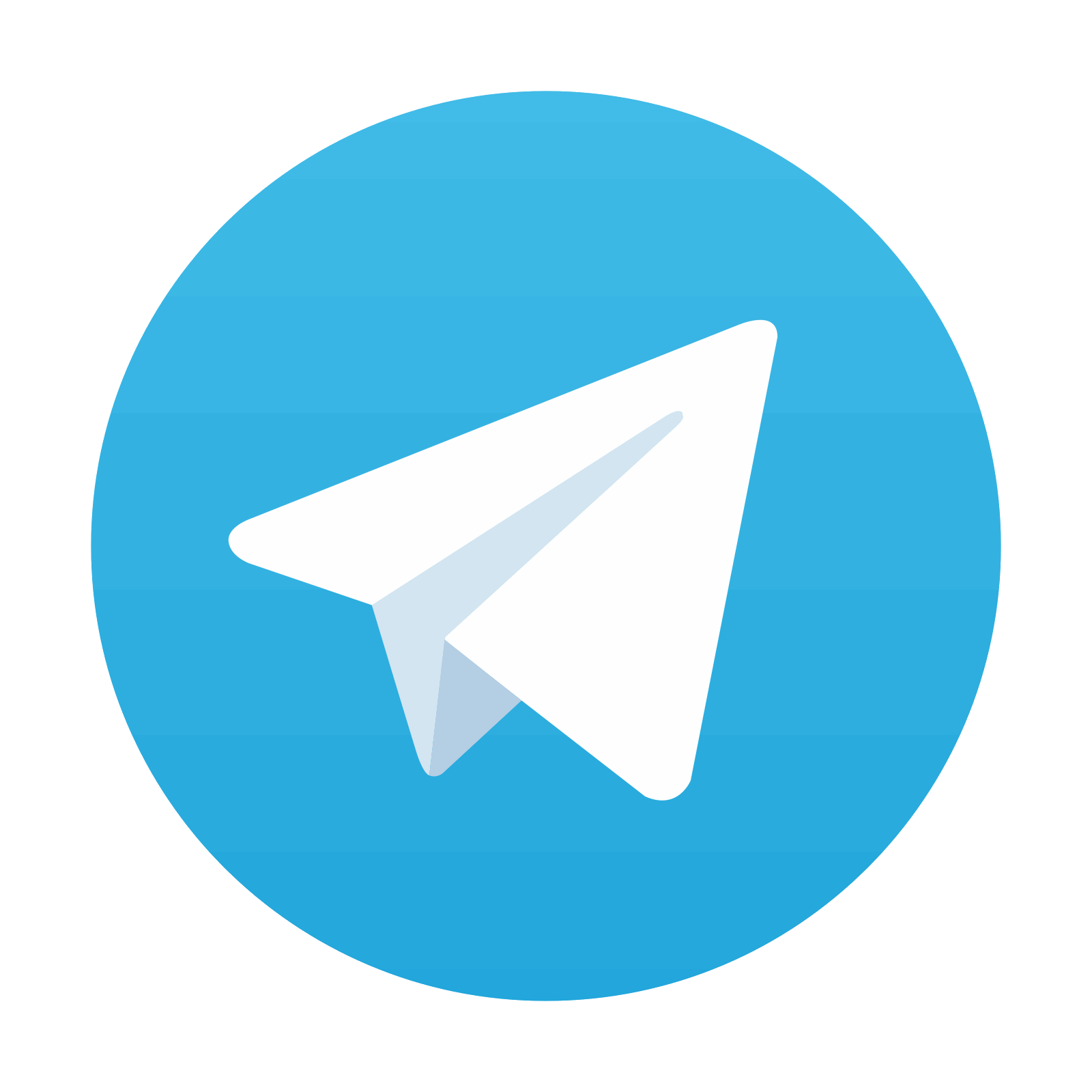
Stay updated, free articles. Join our Telegram channel

Full access? Get Clinical Tree
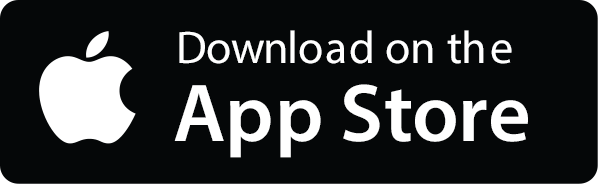
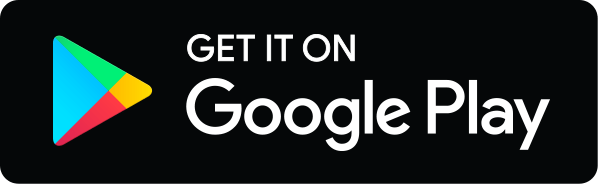
