The role of magnetic resonance (MR) imaging in breast imaging and evaluation has increased rapidly. MR imaging now encompasses diagnostic evaluation as well as screening for breast cancer in high-risk groups, monitoring the extent of disease and the response to chemotherapy. It is expected that the utility of breast MR imaging will continue to increase, requiring additional facilities and expertise. Establishing a breast MR imaging program requires familiarity with several unique issues pertaining to the nature of this imaging modality. This article attempts to address some of these issues, including selection of a magnet based on needs of the particular practice and magnet field strength, selection of a dedicated breast coil, magnet location and siting, advantages and challenges of higher strength magnets such as 3 Tesla, establishing a referral base, scheduling of breast MR examinations, patient safety concerns, and examination interpretation and reporting.
Contrast-enhanced breast magnetic resonance (MR) imaging has been used as an imaging tool for detection of breast cancer for well over 15 years, with reported sensitivities in the range of 83% to 100% and specificities ranging from 29% to 100%. MR imaging provides not only anatomic and morphologic information but also indirectly reflects functional characteristics by imaging the dynamics of blood flow within lesions. Both features are important in the analysis of enhancing lesions. A 1998 study showed that based on qualitative morphologic analysis alone the sensitivity and specificity of breast MR imaging were 83% and 54%, respectively, whereas quantitative analysis alone showed a sensitivity of 83% and specificity of 66%. Combining morphologic assessment with quantitative analysis improved sensitivity to 93% and specificity to 74%, with considerably higher overall accuracy. Therefore, a major challenge in breast imaging has been to achieve high-resolution anatomic imaging while performing the necessary sequences rapidly enough to document the dynamic enhancement kinetics of masses and nonmass-like lesions.
This has been made possible by technical developments that allow high enough spatial resolution for detailed morphologic assessment and more accurate plotting of the time-intensity curves within the short period of time when breast tumors usually reach peak contrast enhancement and undergo washout. Breast MR imaging is now a well-established and widely accepted imaging modality for breast cancer detection and monitoring, with increasing demand for the examination. A 2008 survey of radiology practices in the United States showed that contrast-enhanced breast MR imaging was offered at 74% of 754 surveyed practices, the majority performing less than 20 examinations per week. Mammography Quality Standards Act–accredited radiologists supervised and interpreted breast MR imaging examinations in most facilities. The majority of practices offered screening breast MR imaging and performed MR-guided interventional procedures, and half reported that interpretation was performed with computer-aided detection. It is highly likely that more and more radiology practices will add breast MR imaging and will offer screening and diagnostic or problem-solving MR imaging.
American College of Radiology BI-RADS lexicon
Widespread breast MR imaging has been greatly aided by the standardization of imaging protocols, interpretation, and reporting, which has been possible largely because of the American College of Radiology (ACR) Breast Imaging Reporting And Data System (BI-RADS) lexicon, developed specifically for breast MR imaging and published in the fourth edition of the BI-RADS manual in 2003. The BI-RADS standards provide guidelines for imaging and interpretation criteria and a common language for clear and concise communication.
Indications for breast MR imaging
Further validation for breast MR imaging was provided in 2007, when the American Cancer Society (ACS) published newly expanded guidelines specifying indications for breast MR imaging, including screening for women at high risk (20%–25% lifetime risk), tumor staging, and treatment monitoring. These revised guidelines are based on newer evidence that became available since 2003, which was collected and reviewed by a panel of experts who developed recommendations for women at different defined levels of risk to help identify patients for whom breast MR imaging should be recommended. The ACR supports these guidelines ( www.acr.org ), and these are detailed elsewhere.
It is likely that the group of women at high risk for breast cancer will benefit from screening with MR imaging, as cancers may be found at an earlier stage, and this will lead to reduction in mortality. Moreover, some experts believe that because of its almost 10 times higher sensitivity, MR imaging will likely replace mammography as the screening modality of choice in high- and intermediate-risk women in the not too distant future.
The role of breast MR imaging for surgical planning in patients with recently diagnosed breast cancer continues to be debated, as the cost/benefit ratio is not entirely clear. Those opposing this role of breast MR imaging believe that because of very high sensitivity, the false-positive rate in breast MR imaging raises the “cost” to an unacceptable level, leading to too many unnecessary mastectomies. In a 2007 review Kuhl and colleagues acknowledge that “arguments against the use of breast MR imaging include costs, frequency of false positive diagnoses, lack of availability of minimally invasive biopsy capabilities, lack of evidence by randomized controlled clinical trials, and, last, fear of overtreatment.” However, because MR imaging is significantly superior to mammography and ultrasound (US) in detecting intraductal extension of invasive carcinoma, the benefits of preoperative staging MR imaging cannot be ignored. Kuhl and colleagues suggest that rather than letting incidental and “false positive” findings automatically lead to mastectomy, the guidelines for when mastectomy is necessary could be modified as “some small MR imaging detected additional multicentric breast cancer foci will be sufficiently treated by radiation therapy.”
Despite controversy and debate, the role of MR imaging in diagnostic and screening breast imaging continues to grow, and there is demand for additional facilities with the best possible equipment, with expert performance and interpretations. Keeping up with fast-changing technical developments while in the process of establishing a new breast MR imaging facility or expanding an existing one can be challenging.
This article attempts to address some of the issues that should be considered in the planning stages of such a facility, and draws attention to some important details of setting up an efficient and organized process for examination performance, interpretation, and reporting.
Indications for breast MR imaging
Further validation for breast MR imaging was provided in 2007, when the American Cancer Society (ACS) published newly expanded guidelines specifying indications for breast MR imaging, including screening for women at high risk (20%–25% lifetime risk), tumor staging, and treatment monitoring. These revised guidelines are based on newer evidence that became available since 2003, which was collected and reviewed by a panel of experts who developed recommendations for women at different defined levels of risk to help identify patients for whom breast MR imaging should be recommended. The ACR supports these guidelines ( www.acr.org ), and these are detailed elsewhere.
It is likely that the group of women at high risk for breast cancer will benefit from screening with MR imaging, as cancers may be found at an earlier stage, and this will lead to reduction in mortality. Moreover, some experts believe that because of its almost 10 times higher sensitivity, MR imaging will likely replace mammography as the screening modality of choice in high- and intermediate-risk women in the not too distant future.
The role of breast MR imaging for surgical planning in patients with recently diagnosed breast cancer continues to be debated, as the cost/benefit ratio is not entirely clear. Those opposing this role of breast MR imaging believe that because of very high sensitivity, the false-positive rate in breast MR imaging raises the “cost” to an unacceptable level, leading to too many unnecessary mastectomies. In a 2007 review Kuhl and colleagues acknowledge that “arguments against the use of breast MR imaging include costs, frequency of false positive diagnoses, lack of availability of minimally invasive biopsy capabilities, lack of evidence by randomized controlled clinical trials, and, last, fear of overtreatment.” However, because MR imaging is significantly superior to mammography and ultrasound (US) in detecting intraductal extension of invasive carcinoma, the benefits of preoperative staging MR imaging cannot be ignored. Kuhl and colleagues suggest that rather than letting incidental and “false positive” findings automatically lead to mastectomy, the guidelines for when mastectomy is necessary could be modified as “some small MR imaging detected additional multicentric breast cancer foci will be sufficiently treated by radiation therapy.”
Despite controversy and debate, the role of MR imaging in diagnostic and screening breast imaging continues to grow, and there is demand for additional facilities with the best possible equipment, with expert performance and interpretations. Keeping up with fast-changing technical developments while in the process of establishing a new breast MR imaging facility or expanding an existing one can be challenging.
This article attempts to address some of the issues that should be considered in the planning stages of such a facility, and draws attention to some important details of setting up an efficient and organized process for examination performance, interpretation, and reporting.
Magnet selection
Almost all of the reported literature in breast MR imaging is based on imaging performed on field strength magnets of 1.5 Tesla (T) or greater. More recently, clinical 3-T magnets have become available and are being used at academic institutions as well as in the private practice setting. The specific needs of a particular practice will help determine the optimal magnet. In a multispecialty radiology group, the needs of high-volume users such as neuroradiology, musculoskeletal radiology, and cardiac imaging must be taken into account, and input from colleagues in these specialties should be sought from the beginning. In such a group the advantages and disadvantages of 1.5- versus 3-T magnets in each subspecialty area of imaging would need to be determined.
On the other hand, for an exclusively breast imaging group, a dedicated breast imaging system could be considered. A dedicated system is designed to provide maximal patient comfort in the prone bilateral breast imaging coil, and some systems have an open design to address the not uncommon issue of claustrophobia. There is currently no dedicated breast magnet available at 3 T.
If the magnet is to be used for breast imaging only, financial feasibility to justify such an installation needs to be determined. Factors influencing the cost/benefit ratio of a system used for breast imaging only include the following: is the magnet in an inpatient or outpatient facility; is it to be housed in a newly constructed free-standing facility or in a preexisting space which is to be renovated; is the magnet being added to an existing imaging suite, thereby sharing the operating expenses such as personnel, supplies, utilities, overhead; and will the MR imaging facility be used for diagnostic breast imaging only or also for image-guided interventional procedures. These factors need to be balanced by projected breast imaging volumes. If MR imaging-directed interventional procedures will be performed, not only will the appropriate biopsy-capable breast coils be essential, but the facility will need to be designed to accommodate all other necessary equipment. Whether only diagnostic imaging or interventional procedures also are performed at a free-standing outpatient facility, patient safety mechanisms for emergency situations such as contrast reactions or complications of interventional procedures should be worked out in advance and put in place.
Selecting magnet strength
Current Status of Breast Imaging at 1.5 T
Breast imaging is inherently challenging because of the need to image both breasts repeatedly over a short time period. As mentioned earlier, high spatial resolution is necessary to assess morphologic features of benign and malignant tumors, whether these are seen as masses or areas of nonmass enhancement. In the case of masses, margins are the most reliable predictors of benign versus malignant pathology, and detailed and reproducible margin assessment is best performed on high-resolution images with in-plane spatial resolution less than 1 mm. Because tumor neoangiogenesis is the basis of breast cancer detection on MR imaging, dynamic scanning is essential to evaluate the arterial and venous phases of tumors. To achieve this, it is necessary to image both breasts before contrast administration and then repeatedly and rapidly after contrast is given. In this way images are obtained during the arterial phase to demonstrate the rate of contrast uptake by the tumor, and repeat scans over the next 7 to 8 minutes allow demonstration of the delayed phase or washout characteristics. Most breast cancers have rapid uptake of contrast and, due to the presence of abnormal tumor vessels, also show rapid washout, which can be reflected by the dynamic sequences.
Fat is seen in MR imaging as very high signal, which can mask enhancement of fibroglandular tissue and of abnormal masses. Historically, this problem has been addressed in the United States by applying fat suppression pulses that render the fat signal null. In Europe, subtraction of the precontrast series from identical postcontrast series has been used to cancel out the signal from fat. Although the latter technique has the advantage that there is no loss of signal, it can be problematic if the patient moves during the dynamic scans causing misregistration, which can lead to the appearance of falsely “enhancing” lesions or nonvisualization of truly enhancing areas. Chemically selective fat suppression is best achieved in a homogeneous magnetic field. At intermediate and low field strength field homogeneity is suboptimal, resulting in uneven fat suppression and image degradation ( Fig. 1 ).
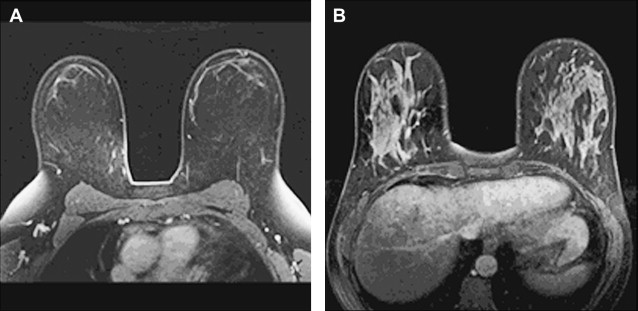
At 1.5 T there are well-established and carefully optimized breast imaging protocols using 3-dimensional gradient-echo sequences, with improved signal to noise ratio (SNR), decreased slice thickness (<1 mm), high in-plane resolution of less than 1 mm × 1 mm, shorter acquisition times caused by decreased repetition time (TR)/echo time (TE) (1–2 minutes), and bilateral full coverage, using parallel imaging sequences. All these factors ultimately provide breast MR imaging scans with high spatial and temporal resolution, so that detailed morphologic analysis can be performed without sacrificing the ability to characterize enhancement kinetics. Because there is a linear relationship between magnet strength and SNR, higher field strength such as at 3 T would theoretically provide images of very high spatial resolution. However, in the typical clinical setting the gain in SNR is offset by several technical modifications necessary to obtain quality images in the higher magnetic field. These factors include adjustment of the TR and flip angles, and modification of protocols to keep tissue heating (specific absorption ratio), dielectric effects, and B 1 field inhomogeneities within reasonable limits.
Although there is a paucity of literature comparing diagnostic clarity and accuracy of breast MR imaging using 1.5-T and 3-T magnets, and no definitive evidence has yet been reported that imaging on the higher field strength is more sensitive or accurate, there is increasing evidence that imaging at 3 T is at least as good as at 1.5 T. Specific applications such as MR spectroscopy and diffusion-weighted imaging may prove to be better performed on the higher strength magnet. In 2006 Kuhl and colleagues reported that “differential diagnosis of enhancing lesions is possible with higher diagnostic confidence at 3 T MR imaging.” However, the same group then published concerns about limited contrast enhancement at 3 T, saying that “due to spatial B 1 inhomogeneities across the field of view, enhancement of lesions may be reduced to a variable degree.” In a response to these concerns, Mountford and colleagues emphasized the importance of protocol design and technique in optimizing the performance of breast MR imaging at 3.0 T, and suggested that the issue of field inhomogeneity can be compensated for by adjusting other parameters in the system.
Advantages at 3 T
The most significant inherent advantage of the higher strength magnet is improved signal strength which, at 3 T is about twice that of a 1.5-T magnet. This higher signal strength provides higher SNR and allows performance of examinations with thinner slices, providing higher in-plane resolution. In addition, chemical shift effect scales in proportion to magnet field strength, doubling from 1.5 to 3 T, and providing improved fat suppression sequences as well as allowing better separation of metabolite peaks for spectroscopy. This finer analysis of the choline peak may provide the ability to distinguish invasive carcinoma from benign lesions and possibly from ductal carcinoma in situ, in vivo. With higher signal strength there is also an associated higher susceptibility effect. This effect has clinical applicability in the improved fast spin echo sensitivity to hemorrhage in certain modified protocols. Higher signal intensity may allow more detailed diffusion-weighted (DW) imaging. The potential role of DW imaging in characterization of breast masses has been studied in recent years. It is currently reported that apparent diffusion coefficient (ADC) values may be a valuable parameter in distinguishing benign from malignant masses. A recently published meta-analysis of 12 published studies evaluating ADC of breast tumors at 1.5 T revealed the pooled sensitivity and specificity to be 89% and 77%, respectively. In contrast, a single-institution report of DW imaging of breast lesions at 3 T showed the sensitivity and specificity to be 95% and 91%, respectively.
Challenges at 3 T
Image quality
The advantages of distinctly better SNR of a 3-T magnet for clinical imaging are balanced by several obstacles, namely higher radiofrequency deposition, increased tissue T1 relaxation times, dielectric resonances, stronger susceptibility effects, and larger chemical shift ( Fig. 2 ). To overcome these obstacles, attention to imaging protocol parameters is required. Radiofrequency deposition in tissues scales exponentially with increasing magnet strength, and causes tissue heating. This deposition of energy as heat is quantified as specific absorption ratio (SAR). The International Electrotechnical Commission (IEC) has established SAR limits for clinical examinations at 8 W/kg over 5 minutes or 4 W/kg for the whole body over 15 minutes. Imaging protocols at 3 T can be modified by adjusting the TR and TE to keep the SAR within acceptable limits.
