Fig. 1
Representative slices of ifc-MRI networks averaged from 22 healthy controls
In patients with brain tumors, ifcMRI has been used to investigate the connections and strength of the connections between cortical areas (Manglore et al. 2013). In a study of the capacity of ifcMRI to identify the seizure focus in a group of patients with non-lesional epilepsy, the authors concluded that ifc-MRI may serve as an adjunct presurgical tool facilitating the identification of the seizure focus in patients with focal epilepsy (Weaver et al. 2013).
2.1.3 Computer-Based Analysis of Structural MRI
The so-called computational neuroanatomy is an established methodology to characterize the neuroanatomical configuration of different brains, encompassing mainly the following techniques: (1) voxel-based morphometry (VBM) which compares neuroanatomical differences on a voxel by voxel basis; (2) deformation-based morphometry (DBM) which provides information about global differences in brain shape; (3) and tensor-based morphometry (TBM) which provides information about local shape differences (cf. Ashburner et al. 2003 for review). These 3-D MRI analysis techniques which make use of local registration algorithms, however, are only validated for analyses at group level: it could be demonstrated that VBM could not satisfactorily delineate the spatial extent of focal lesions in individuals, even in simulations that avoided preprocessing artifacts (Mehta et al. 2003). To be applicable in the clinical practice and, in particular, in the presurgical setting, the techniques have to be sensitive to quantitative or qualitative alterations of normal brain structures in a given individual patient. In this respect, it is not useful to apply this computerized approach in lesions which are clearly assessable by conventional high-resolution MRI such as in neoplasms. Nevertheless, there are applications of computerized analysis of structural 3-D MRI for presurgical diagnostics, e.g., in the field of epilepsy surgery and here, e.g., in malformations of cortical development (MCD). First, there have been voxel-based 3-D MRI analysis tools developed for an automated observer-independent MCD lesion detection (Huppertz et al. 2005). These algorithms serve mainly as image screening tools in comparison to a healthy control sample in order to facilitate MCD detection and localization. Second, as a consequent continuation of the multimodality approach in the sense of a combined voxel-based mapping (Kassubek et al. 2000), a combination of ligand imaging using PET (which is able to provide relevant information on MCD; Cross 2003) and SPM-based MRI analysis has been proposed for the investigation of MCD (Richardson et al. 1997). Although at the expense of the measurement of absolute quantitative values (the voxel-based comparison of MRI and PET enables an automated objective whole-brain analysis), issues concerning partial volume effects and mixed tissue sampling in ligand images have to be resolved, and disproportionate changes in function and structure to be identified. In a study using the approach of voxel-based MRI analysis and subtraction of ictal SPECT co-registered to MRI (SISCOM), it could be demonstrated that multimodal assessment of MCD may contribute to an advanced observer-independent preoperative assessment of the seizure origin and might thus improve presurgical diagnostics in symptomatic epilepsy (Wiest et al. 2005). Wehner and Lüders (2008) summarized the role of neuroimaging in presurgical mapping focusing on structural MRI, PET, and SPECT.
2.1.4 Diffusion Tensor Imaging (DTI)
Diffusion is a physical process involving the translational movement of molecules via thermally driven random motions, the so-called Brownian motion. The underlying microstructure of the cellular tissue influences the overall mobility of the diffusing molecules by providing numerous barriers and by creating various individual compartments within the tissue (e.g., intra-/extracellular space, neurons, glial cells, axons). Signal intensity of diffusion measured via diffusion-weighted imaging shows a greater signal enhancement and more rapid diffusion of water molecules along the direction parallel to the axis of the fiber bundles (so-called anisotropy) than in the perpendicular direction where signal intensity and diffusion velocity is lowest (so-called isotropy). As diffusion is characterized by hindrances of equal molecular movement caused by oriented barriers, it requires a general tensor which fully describes the mobility of molecules along each direction and the correlation between these directions. A common way to summarize diffusion measurements with DTI is the calculation of a parameter that reflects the overall anisotropy, such as the fractional anisotropy (FA) as a measure of the proportion of the diffusion tensor due to anisotropy. The FA can either be presented as a gray-scale image or the principal diffusion direction of the brain structure to be examined can be encoded in color resulting in color-coded parameter maps (Basser and Jones 2002; Le Bihan and van Zijl 2002).
Concretely, DTI measures the mean longitudinal direction of axons in white matter tracts. For this purpose, the application of fiber-tracking algorithms allows a delineation of the major white matter pathways (Mori and van Zijl 2002). With respect to presurgical mapping, the DTI technique provides information about the deformation, the displacement, or even the interruption of the white matter tracts around a lesion (Sinha et al. (2002). For instance, DTI is used to differentiate normal white matter from edematous brain and contrast-enhancing tumor margins in patients with high-grade gliomas. Witwer et al. (2002) reported that the ¬ isualization of white matter tracts by DTI was beneficial in the surgical planning for patients with intrinsic brain tumors showing that anatomically intact fibers may be present in abnormal-appearing areas of the brain. The knowledge of the course of major white matter tracts in relationship to the exact location of the lesion is of utmost importance to the neurosurgeon for the planning of the most appropriate surgical approach and may thus help to prevent the risk of new postoperative neurological deficits (Clark et al. 2003). For topographical identification, DTI atlas systems have been developed (Oishi et al. 2011). Figure 2 shows an example of a patient with a glioma showing DTI-based structural connectivity changes adjacent to the left cortex.
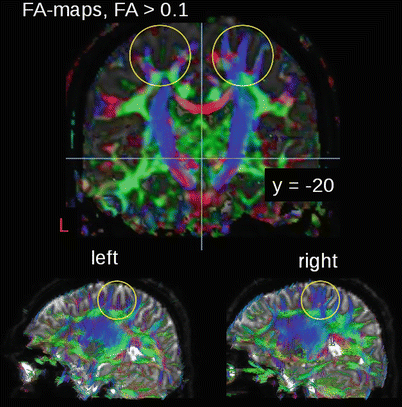
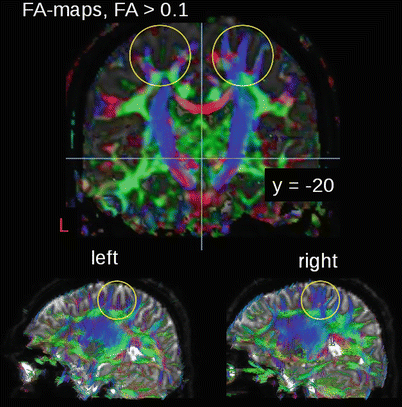
Fig. 2
FA-mapping of a patient with a glioma showing FA reductions in the cortex of the left hemisphere, with locally altered fiber tracts
The role of DTI for the assessment of important pathways in the vicinity of tumor borders has already been described in 2004 by Ulmer and coworkers (2004) who concluded that DTI can significantly enhance the estimation of the proximity of functional brain structures/areas to brain tumor borders suggesting DTI to be a valuable tool for presurgical planning. This work was followed by a pre- and postoperative DTI-based analysis (white matter tractography) of brain tumors (Lazar et al. 2006) showing the preservation of white matter tracts during surgery. Ferda and coworkers (2010) reported that DTI offered significant additional information in 24 patients with glial tumors which might help for the differentiation of tumors with infiltrative growth character from more circumscribed tumors, and it has been possible to advance the accuracy of estimation of tumor grading prior to final determination of the morphological diagnosis. The finding of a reduced FA in the white matter around a tumor increases the probability of peritumorous white matter infiltration by tumor invasion. Figure 3 shows an example of the application of a DTI analysis in a patient with a biopsy-proven anaplastic oligoastrocytoma WHO III° by comparing the FA maps acquired before combined radio-/chemotherapy with those obtained 2, 5, and 9 months after radio-/chemotherapy (for the methodological approach, please cf. Müller and Kassubek 2013). After surgery, the patient received combined radio-/chemotherapy and 6 cycles of adjuvant chemotherapy.
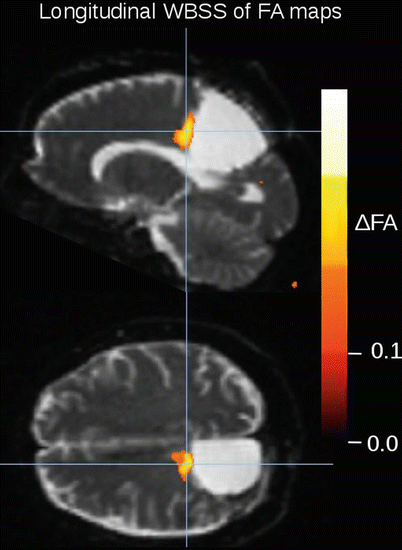
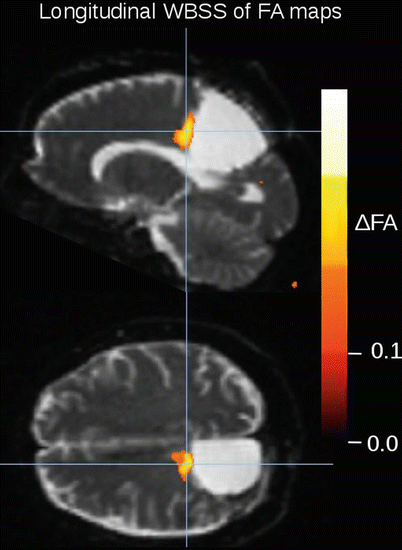
Fig. 3
FA-map differences between the DTI scan after radio-/chemotherapy and the DTI scan 7 months later, i.e., scans of time points 2 and 4. Longitudinal single-subject whole-brain-based spatial statistics (WBSS) was performed with a FDR corrected FA threshold of 0.15
Consecutively, DTI measurements are increasingly used in advanced neuronavigation protocols (Rohde et al. 2005). That way, the course of the pyramidal tract can be displayed with DTI for the intraoperative visualization in functional neuronavigation systems (Nimsky et al. 2005b). Moreover, DTI is helpful for the visualization of the optic radiation to preserve the patients’ visual field during the surgery of lesions adjacent to the course of the optic radiation. For instance, Coenen et al. (2005) could reproduce the course of the optic radiation by using neuronavigation with optic radiation DTI and preoperative and postoperative neuro-ophthalmological testing including perimetry. Also in epilepsy surgery, visual field defects are a well-recognized complication of anterior temporal lobe resection and may occur secondary to disruption of the Meyer loop. The use of DTI tractography for the visualization of the optic radiation before and after surgery has been concluded to be useful for the prediction and perhaps prevention of visual field defects following temporal lobe resection (Powell et al. 2005).
However, there are also limitations for DTI which is not able to correctly identify fiber crossings due to its inability to resolve more than one single axon direction within each voxel. Advanced approaches, such as the so-called q-ball imaging technique, can resolve intra-voxel white matter fiber crossings as well as white matter insertions into the cortex and might hence be able to uncover the complex intra-voxel fiber architecture and thus eliminate a key obstacle for the noninvasively mapping of neural connectivity in the human brain (Tuch et al. 2003). An approach which addresses the limits of diffusion resolution of “crossing” or “kissing” fibers and in the vicinity of tumor or edema is tractography method based on high-angular-resolution diffusion imaging (HARDI). With compressed sensing techniques, HARDI acquisitions with a smaller number of directional measurements can be used, thus enabling the use of HARDI-based fiber tractography in the clinical practice (Kuhnt et al. 2013; Bucci et al. 2013). More recent investigations applied Q-ball imaging as high-definition fiber tractography (HDFT) to patients with intracerebral lesions (Fernandez-Miranda et al. 2012) in order to understand the lesional patterns of structural injury and facilitate neurosurgical interventions. A further application of HFTD was reported in a patient with traumatic brain injury (Shin et al. 2012). An additional technical challenge, especially in presurgical mapping, i.e., the intraoperative displacement of major white matter tracts during mass resection due to brain shift, has been addressed by a comparison of preoperative with intraoperative DTI-based fiber tracking (Nimsky et al. 2005c). The authors described a marked shift and deformation of major white matter tracts due to tumor removal, herewith emphasizing the need for an intraoperative update – in navigation systems – during the resection of deep-seated tumor portions near eloquent brain areas. Consequently fiber tracking is not only useful for preoperative neurosurgical visualization but also for intraoperative planning and updating. Another emerging challenge for the clinical routine use is the lack of standardization which affects DTI as well as fMRI. There are many different software packages available for tractography, including traditional DTI-based methods (such as the fiber assignment by a continuous tracking algorithm), as well as more advanced methods that take into account each eigenvector within an individual voxel (Pillai 2010).
The integration of DTI into presurgical mapping protocols is also useful with respect to MRI multimodality, since the bi-parametric combination of DTI with fMRI provides valuable information that cannot be obtained by the use of either technique alone. The use of fMRI and DTI in a multimodality approach enables the connection of the advantages of both techniques. While fMRI is able to highlight the localization of function within the cortex, DTI visualizes white matter structures in vivo (Dimou et al. 2013; Kleiser et al. 2010; Wu et al. 2007). The validity and sensitivity of noninvasive functional mapping for surgical guidance can be improved by considering the results obtained with both methods, and the use of 3-D visualization seems crucial and unique for the representation and understanding of the complicated spatial relationship among the lesion, gray matter activation, and white matter fiber bundles (Hendler et al. 2003). In neoplastic brain lesions, the combined use of fMRI with DTI can provide a better estimation of the proximity of the tumor borders to eloquent brain systems, such as language, speech, vision, motor, and premotor functions (Ulmer et al. 2004). DTI and fMRI can thus be used as complementary techniques for the delineation of eloquent subcortical and cortical areas (Fig. 4).
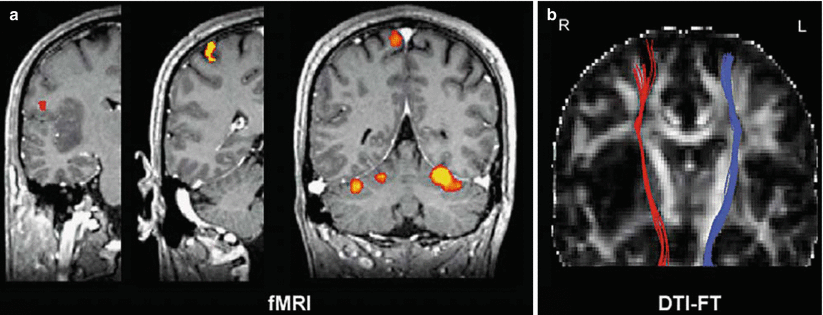
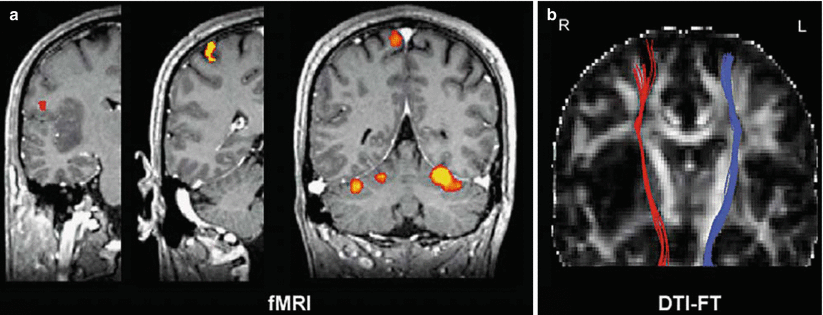
Fig. 4
(a, b) DTI-based fiber tracking of the pyramidal tract for the presurgical delineation of eloquent white matter areas. (a) Somatotopic mapping of primary motor cortex shows a normal activation patterns. From left to right: tongue, hand, and foot movements. (b) DTI fiber tracking visualizing the spatial relationship of the pyramidal tract to an astrocytoma in the right insula (Images courtesy of C. Stippich, MD)
The use of fMRI together with or without DTI currently seems to be a promising application of the available neuroimaging techniques in the clinical context. These multiparametric MRI data can be implemented in computer systems for functional neuronavigation or radiation treatment (Stippich 2010). There has been significant interest in using this bi-parametric MRI approach for the localization of potential brain regions which may be at risk during neurosurgical procedures. There is an increasing number of groups worldwide that have integrated both modalities in stereotaxic guidance systems, providing clinical insight in the mapping of eloquent gray matter and white matter regions to the neurosurgeon (Dimou et al. 2013). It has been reported that as the distance between tumor and eloquent cortex (“lesion to eloquent area distance” or “LEAD”) decreases, so does the likelihood of a complete surgical resection. Consequently, DTI was proposed as a tool for the identification of those patients who would benefit most from total surgical resection and, thus, having longer survival time (Castellano et al. 2011). Used in conjunction, both techniques provide important information both prior to surgery for a thorough preoperative planning, as well as during surgery for the intraoperative update of the information via integration into frameless stereotactic neuronavigational systems.
The use of fMRI landmarks for ROI selection has been shown to enhance the fiber-tracking performance compared to a strictly anatomically based ROI selection and is usually considered reliable in controls. Nevertheless, this approach may be inaccurate in patients with brain lesions (Schonberg et al. 2006). Kleiser and coworkers (2010) found that DTI-related results were more accurate than results using anatomical landmarks alone. Four patterns of regional anisotropy have been observed in relation to WM tracts in the vicinity of tumors (Jellison et al. 2004), i.e., (1) normal signal with altered position or direction, observed in cases of tract displacement; (2) decreased but present signal with normal direction and location, supposedly corresponding to vasogenic edema; (3) decreased signal with disrupted maps, believed to be related to tumor infiltration; and (4) loss of anisotropic signal corresponding to fiber tract destruction. In two trials addressing a potential modification of the surgical approach to corticotomy, the presurgical use of combined DTI/fMRI led to a change in the surgical approach in 16–21 % of cases (Dimou et al. 2013). Together, these techniques show great promise for improved neurosurgical outcomes. Clinical reports have suggested that the additional information provided by the combination of the two imaging modalities, via a co-registered map delivered to the neurosurgeon on a heads-up display, is valuable and facilitates a safe resection (Berntsen et al. 2010). While further research is required for a more widespread clinical validity and acceptance, results from the literature (e.g., Schonberg et al. 2006; Bello et al. 2008; Kleiser et al. 2010; Kuhnt et al. 2012, 2013) are very promising to cement these techniques into the clinical setup in the very new future. For instance, more recent works have applied DTI-based fiber tracking in intraoperative glioma surgery (Kuhnt et al. 2012).
2.1.5 MR Spectroscopy
In vivo proton (1H)-MR spectroscopy (MRS) visualizes signals from carbon-bound, non-exchangeable protons, showing the highest information density in the spectral region from 1 to 5 ppm (Trabesinger et al. 2003). Principal metabolites detected by MRS include the neuronal marker N-acetylaspartate as an indirect expression of the integrity of neurons, choline-containing compounds (such as metabolites involved in phospholipid membrane synthesis) as markers for glial activity, creatine (including phosphocreatine) as a marker for energy metabolism, and lactate as an indicator for anaerobic glycolysis detected under pathologic conditions. For data acquisition, there are single-voxel and multiple-voxel techniques, depending on the information required. If applied in a larger area of expected signal alteration, the latter would be preferable as a screening for abnormalities in different locations within a bigger region (Barker 2005).
In the presurgical diagnostics of epilepsy surgery, MRS has shown to have additional potential to be used in imaging protocols for the presurgical evaluation of non-lesional frontal lobe epilepsy (Guye et al. 2005) and for the assessment of the surgical outcome in patients with temporal lobe epilepsy (Antel et al. 2002). In this context, the combination of different approaches such as MRS and 3-D MRI-based measurements of regions of interests (ROIs) such as the amygdala-hippocampal volume has been found to provide complementary results in presurgical neuroimaging protocols (Cendes et al. 1997). MRS has been established as part of presurgical multimodality neuroimaging studies using various MRI-based techniques and PET in patients with non-lesional unilateral temporal lobe epilepsy (Knowlton et al. 1997) and also in the in vivo analysis of metabolic disturbances associated with partial epilepsy (Knowlton 2004).
MRS may be used in the diagnostics of brain tumors for the identification of regions of viable tumor, investigation of the tumor extent, and monitoring of the effectiveness of the applied therapy (Vigneron et al. 2001). Consecutively, MRS has now been integrated into presurgical mapping protocols for glioma surgery to indicate the degree of tumor invasion by the co-registration of the metabolic maps derived from MRS postprocessing onto the anatomical 3-D MRI data set for its use in neuronavigation/frameless stereotaxy systems (Ganslandt et al. 2005). In this study, the stereotactic biopsies were acquired by the use of the spectral data, and the tumor areas as defined by the metabolic maps and as histopathologically confirmed by biopsy exceeded the T2-weighted signal change on conventional MRI in all seven cases investigated. The authors concluded that MRS may be useful for a more accurate definition of the tumor infiltration zone in glioma surgery than conventional anatomical MRI alone. Furthermore, a combination of MRS with functional landmarks obtained from fMRI is possible to increase the sensitivity for the assessment of tumor borders and the localization of functionally eloquent areas. Furthermore, intraoperative high-field MRI with integrated microscope-based neuronavigation enables the acquisition of MRS also during the surgical procedure for immediate intraoperative update and control (Nimsky et al. 2005a).
2.2 Combination of MRI and Nuclear Medicine
2.2.1 Principles of PET/CT, PET/MRI, and SPECT/CT
The integration of PET/CT, PET/MRI, and SPECT/CT in combination into presurgical multimodal functional neuroimaging allows the augmentation of a quantitative information of regionally disturbed brain function or regional tumor metabolism into the common framework of image analysis and display, depending on the radiopharmaceutical used for the imaging study. In general, PET and SPECT generate images of the regional and temporal distribution of a radiolabeled pharmaceutical after IV injection. Integrated scanner designs allow for sequential or simultaneous acquisition of PET and SPECT data, respectively, with CT scanning and, more recently, with simultaneous MRI as well. For SPECT, pharmaceuticals are usually labeled with the photon emitters 99mTc or 123I, which, due to their radiochemical properties and half-life of 6 and 13 h, respectively, require either a daily on-site preparation of the radiopharmaceuticals or a delivery from a regional provider. The positron emitters needed for PET, however, have half-lives ranging from 2 h (18F) to 2 min (15O) which implies that for all preparations of radiopharmaceuticals apart from the 18F-labeled compounds, a local cyclotron facility is mandatory. Image resolution depends on the technique and instrumentation used, ranging from a physical resolution of 10–15 mm full width at half maximum (FWHM) for SPECT to 3 mm FWHM for PET/CT in high-resolution techniques. For SPECT, image resolution directly correlates with the distance between the detector and the patient’s head. Further, the number of detectors used (single-headed cameras versus triple-headed cameras) markedly improves image quality as well. From a technical point of view, there is no doubt that, whenever possible, PET should be preferred to SPECT and that when not available, SPECT using triple-headed cameras should be preferred to SPECT using a double-headed camera.
2.2.2 Radiopharmaceuticals, Their Applications, and Clinical Indications for Combined MRI and Nuclear Medicine Imaging
99mTc-d,l-Hexamethylene-propylenamin-oxime (99mTc-HMPAO) and 99mTc-ethyl-cysteinate-dimer (99mTc-ECD) are lipophilic molecules with a high first-pass extraction and near-total intracranial retention, used for the depiction of regional blood flow. As these tracers are trapped within the perfused cortex within seconds, the time of injection and not the time of brain scanning determines the measured perfusion state which makes these two tracers ideal for ictal studies in epilepsy, where the imaging itself can be postponed to a time after seizure cessation. The sensitivity and specificity of the detection of the ictally hyperperfused epileptogenic zones is increased when using the SISCOM procedure, where an interictal SPECT perfusion scan using HMPAO-SPECT or ECD-SPECT is subtracted from a strictly ictally injected perfusion scan and consequently co-registered to a 3-D MRI image set (O’Brien et al. 2004). The combination of SISCOM with voxel-based analysis from MRI has been shown to further improve the detection of temporal and extratemporal epileptogenic lesions (Wiest et al. 2005; Matsuda et al. 2009; Kimura et al. 2012) (Fig. 5).
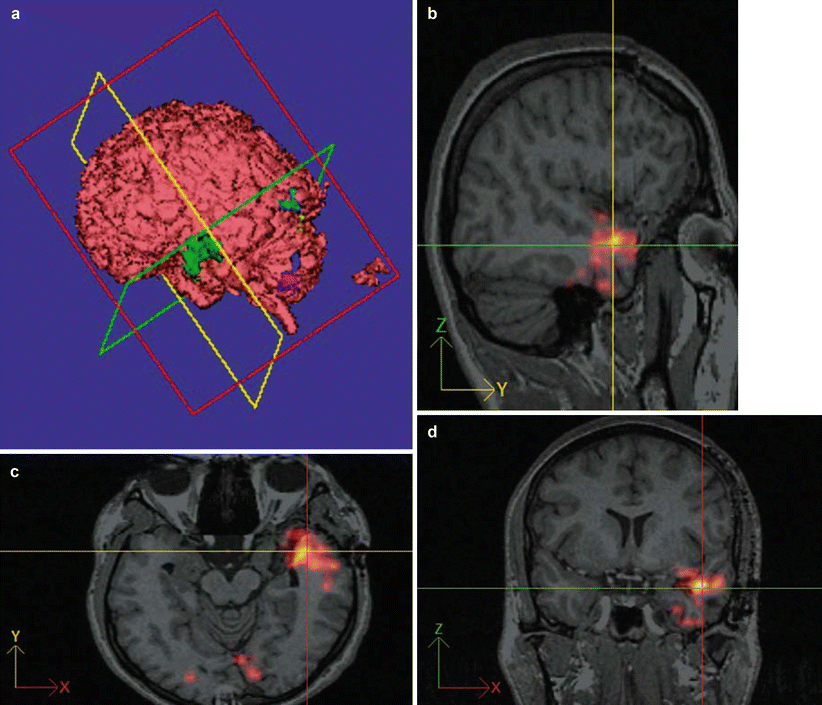
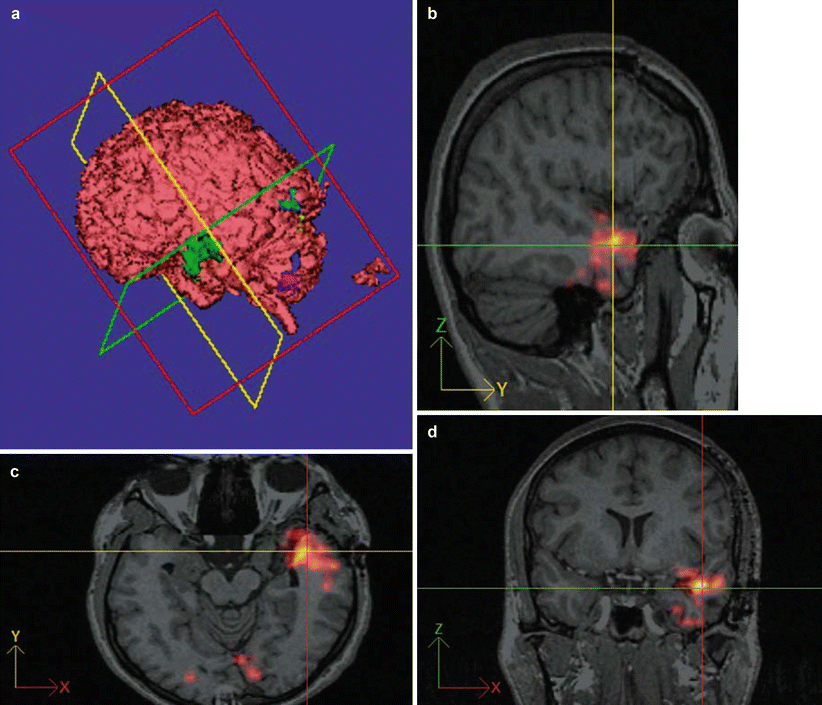
Fig. 5
(a–d) Presurgical activation map of ictally hyperperfused brain areas (using the SISCOM procedure and 99mTc ECD for the SPECT scan) in a patient with left temporal seizures scheduled for epilepsy surgery. Activated brain areas (a) (i.e., perfusion differs more than two standard deviations from the mean difference) are projected onto the co-registered 3-D MRI data set in the sagittal (c), coronal (d), and transverse (b) planes as well as on a surface reconstruction (Images by F.D. Juengling, MD)
In addition, 123I-iomazenil, a selective antagonist for the central GABA-A-benzodiazepine-receptor complex is used for presurgical epilepsy diagnostics (Kaneko et al. 2006) and 123I-tyrosine, an amino acid tracer, is used for the depiction of the amino acid metabolism of tumors (cf. Juengling and Kassubek 2002; Kassubek and Juengling 2002 for reviews).
18F-Fluorodeoxyglucose (FDG), the most commonly used PET radiopharmaceutical, is a glucose analog actively transported into the cell compartment by the glucose transporter GT-1 and then metabolically trapped within the cell. Tumor cells express more glucose transporters on their cell membrane than normal cells and are usually characterized by an increased demand in glucose. Furthermore, brain tumors show an increased uptake of FDG in contrast to normal tissue. As normal cortical FDG uptake is already high, co-registration with a 3-D MRI data set is mandatory to differentiate tumor uptake from cortical uptake, whenever integrated PET/MRI scanning is not available. An increased FDG uptake may also occur due to locally increased cell density or increased neuronal activity during the uptake period (usually 60–90 min). Decreased FDG uptake is associated with regional neuronal dysfunction. Consequently, FDG-PET may also delineate peritumoral neuronal dysfunction as the interictal area of regional functional deficit in epilepsy. FDG-PET has been demonstrated to indicate the metabolically most demanding tumor part and thus the most informative location for taking a biopsy which therefore may increase the probability of a proper biopsy sample (Levivier et al. 1995; Goldman et al. 1997; Pirotte et al. 2003). In epilepsy surgery, changes in focal FDG-PET uptake (regional decreases) allude to the localization of cortical abnormalities (Muzik et al. 2005).
18F-Ethyl-tyrosine and 18F-tyrosine (FET/F-TYR) are radiolabeled amino acids with the same physical half-life as FDG, developed as substitutes for 11C-methionine (MET), the most commonly used amino acid tracer with a physical half-life of 20 min. FET, F-TYR, and MET show a very low uptake in the normal brain, while they are avidly taken up by gliomas of any grade. Their uptake is mainly mediated by the l-type amino acid transporter system at the blood-brain barrier (BBB). Therefore, increased uptake is typical even in low-grade gliomas in the absence of a BBB disruption, which is a substantial advantage over contrast-enhanced CT or MRI. In contrast-enhancing gliomas, the spatial extent of the increased MET uptake is larger than that of the contrast enhancement (Ericson et al. 1985; Ogawa et al. 1993) and may include not only solid tumor portions but also their surrounding, probably reflecting tumor infiltration. There is also a close correlation with tumor vessel density (Kracht et al. 2003) and prognosis (Ribom et al. 2001). In a series of 89 low-grade gliomas, MET uptake was a significant survival factor among patients with astrocytomas and oligodendrogliomas (Ribom et al. 2001). These results suggest that MET uptake (or FET/F-TYR-Uptake) should be evaluated with respect to therapeutic response as tumor resection seems to be a favorable prognostic factor in patients with a high MET uptake but not in patients with a low uptake.
Herholz et al. showed the sensitivity and specificity of MET-PET in the differentiation between non-tumoral tissue and low-grade gliomas to be 76 and 87 %, respectively (Herholz et al. 1998). Comparative analyses between CT, MRI, MET-PET, and stereotactic biopsies have suggested that MET-PET has a greater accuracy in defining the extent of gliomas than CT or MRI alone (Bergstrom et al. 1983; Braun et al. 2002; Mosskin et al. 1989; Ogawa et al. 1993). To improve intraoperative tumor delineation, fused images of FET/FMT/MET-PET and contrast-enhanced MRI have been integrated into neuronavigation systems such as the Zeiss MKM system (Braun et al. 2002) or the BrainLab Vector Vision system (Grosu et al. 2003; Cizek et al. 2004; Vollmar 2006). In a matched comparison of presurgical MET-PET with sterotactic histopathology, it has been shown that MET-PET can delineate solid parts of brain tumors as well as the surrounding infiltration area with a high sensitivity and specificity, if global maxima are normalized to contralateral normal brain tissue and thresholded at a value of 1.3-fold uptake of contralateral normal brain tissue (Kracht et al. 2004). Suitable tumor normalization and thresholding methods have been implemented since then, e.g. via a dedicated visualization tool called “VINCI” (Vollmar et al. 2004) (Fig. 6).
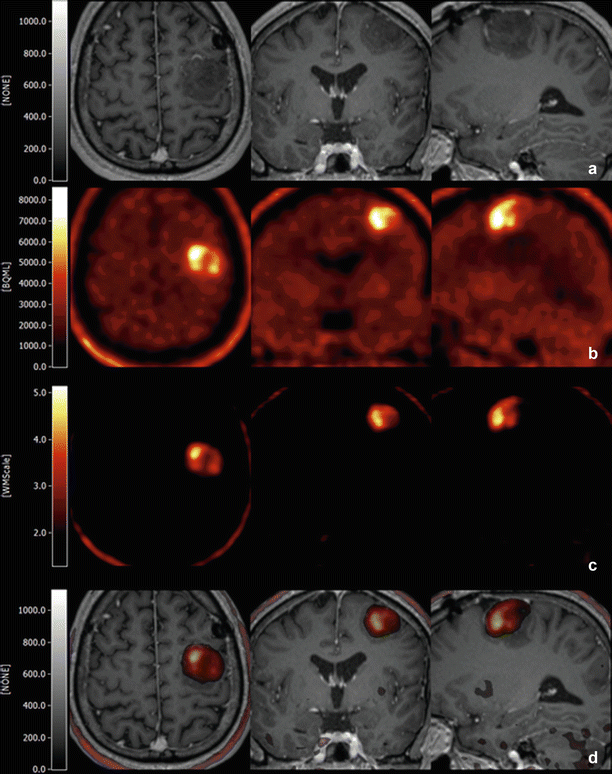
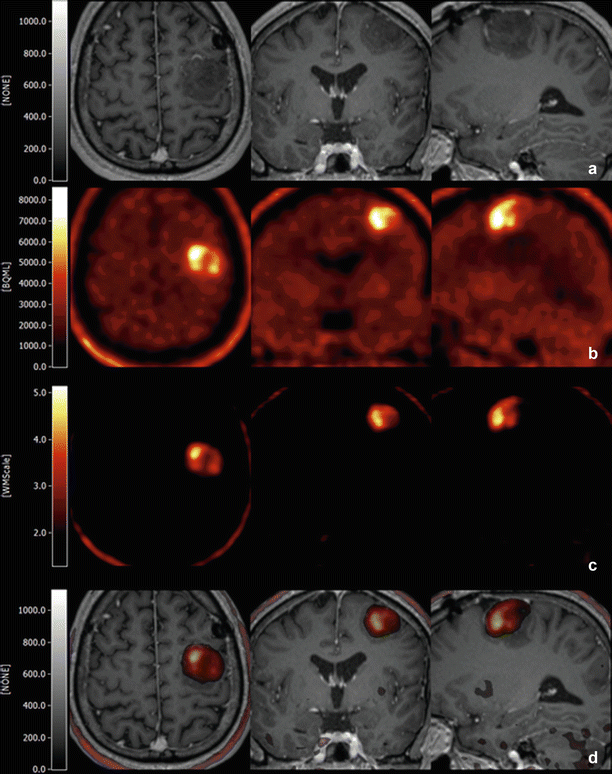
Fig. 6
(a–d) Example of presurgical multimodality imaging in a patient with a glioblastoma multiforme. Tl-weighted MRI (a), fused with the tumor-normalized MET-PET/CT (d) within VINCI (Vollmar et al. 2004). The full range MET-PET is shown in b, and the MET-PET normalized to white matter in (c) (Images by F.D. Juengling, MD)
In Cushing disease, where determination of the exact localization of an ACTH-secreting macro- or microadenoma of the pituitary gland is essential for the cure of this otherwise potentially fatal disease, MR imaging is often unsatisfactory missing up to 60 % of surgically removed microadenomas. Even in combination with superselective cavernous sinus sampling, localization of a microadenoma may fail. Presumably due to a higher cellularity and secretory activity, corticotroph adenoma presents with a higher MET uptake than the normal pituitary tissue, resulting in higher sensitivity for the localization and delineation of a Cushing adenoma as compared to high-resolution 3 T-MRI (including dynamic techniques) or CT scanning alone (Ikeda et al. 2010). This has induced to use MET-PET for surgical planning in specialized centers (Fig. 7).
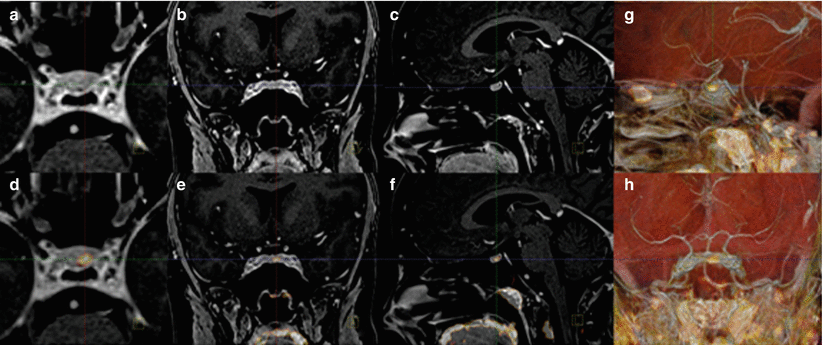
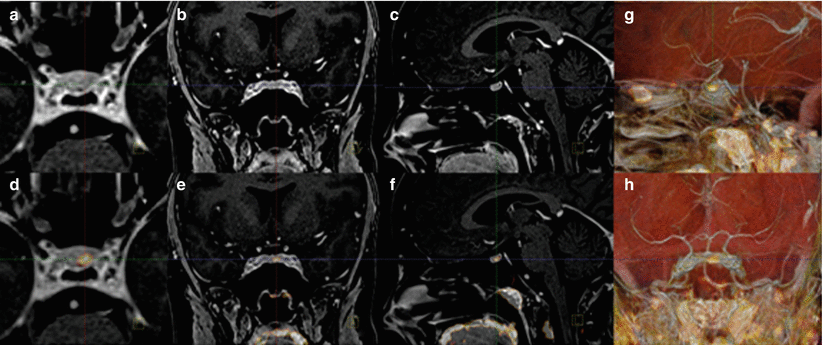
Fig. 7
(a–h) T1-weighted MRI of a patient with a Cushing microadenoma (a–c) fused with MET-PET/CT (d–h). Please note the excellent localization of the microadenoma as visualized by MET-PET/CT, co-registered to MRI, and confirmed by clinical remission after surgery (Images by F.D. Juengling)
18F-DOPA is a fluorinated form of l-DOPA and primarily used to measure the aromatic amino acid decarboxylase activity in monoaminergic neurons of the presynaptic pathways in movement disorders. It has gained attention for imaging newly diagnosed or recurrent brain tumors with similar physical properties as 11C-methionine (Becherer et al. 2003). It could be shown that the 18F-DOPA uptake in human astrocytomas is mediated by the l-type amino acid transporters 1 and 2 (LAT1/LAT2) (Youland et al. 2013) and can thus can be used as an alternative radiotracer for FET, F-TYR, and MET. In a direct comparison, 18F-DOPA has demonstrated higher contrast ratios over FET for lesions outside the striatum (Kratochwil et al. 2014), which was most pronounced in low-grade gliomas, and thus might be superior to FET for the preoperative delineation of tumor borders. Additionally, 18F-DOPA has proven capabilities for the differentiation between progressive brain metastases from late or delayed radiation injury (Lizarraga et al. 2014).
15O-H2O (physical half-life 2 min) is the most frequently used CBF tracer for PET, applied for functional activation (fPET) and allowing up to 12 quantitative measurements of CBF. Compared to fMRI, fPET provides a more physiologically specific and robust signal (Herholz et al. 1997; Kaplan et al. 1999; Krings et al. 2001; Vinas et al. 1997). An essential advantage of fPET over fMRI may be the clinical application in language activation studies, where active speaking during language production tasks does not induce technical artifacts (as is common with fMRI), and therefore a direct monitoring of task performance is possible even in functionally impaired subjects (Thiel et al. 1998). However, more recent advanced fMRI language mapping protocols might in the meantime overcome this problem, thus equalizing the described advantage of fPET.
18F-Flumazenil and 11C-flumazenil (FMZ) are selective antagonists for the GABA-A-benzodiazepine-receptor complex and are mainly used for the detection of epileptogenic areas. It has been demonstrated that FMZ binding is reduced in epileptogenic foci which is probably rather due to a decrease in the affinity of the tracer to the receptor than to a reduction in receptor density alone. Foci of reduced FMZ binding may be found with a high sensitivity of up to 94 % in patients with temporal lobe epilepsy (Ryvlin et al. 1998). As the area of focally decreased FMZ binding may probably indicate the epileptogenic zone that has to be resected to become seizure-free (Muzik et al. 2000; Juhasz et al. 2001), the need for the integration of FMZ into a multimodal approach for the facilitation of the resection of the epileptic zone becomes obvious. The co-registration of PET/CT and SPECT/CT findings to a volumetric MR image or the simultaneous acquisition of PET and MRI (PET/MRI) and their incorporation into an image-guided system has allowed surgeons to offer surgery to patients who may previously not have been considered eligible, with outcomes comparable to those obtained from patients with more straightforward epileptic lesions (Murphy et al. 2004).
11C-Alpha-methyl-tryptophane (AMT) is a marker of the serotonine synthesis capacity, and its uptake is elevated in tuberous sclerosis (Chugani et al. 1998). In the latter, the multiplicity of tubers precludes the resection of the whole potentially epileptogenic tissue, and no MRI technique has proven to be able to differentiate epileptogenic from non-epileptogenic tubers, yet. Recent work has shown that AMT-PET may specifically detect epileptogenic tubers in tuberous sclerosis (Asano et al. 2000), in particular within a multimodality imaging approach (including video-EEG monitoring, FDG-PET, and different MRI techniques), and may even be helpful in the detection of cortical dysplasia (Juhasz et al. 2003). AMT-PET may contribute to the presurgical planning with invasive electroencephalography by determining the localization and extent of the implantation of the grid electrodes and consecutively by defining the resection volume. There are data suggesting that AMT-PET is able to detect non-resected epileptic foci in patients with previously failed neocortical resection and to improve outcome after reoperation of AMT positive tissue (Juhasz et al. 2004). Based on surgical outcome data, resection of tubera with increased AMT uptake yielded an optimal accuracy of 83 % for the detection of tubers that needed to be resected to achieve a seizure-free outcome. These findings suggest that presurgical multimodal imaging for the resection of tubers with increased AMT uptake is highly desirable to achieve a seizure-free surgical outcome in children with tuberous sclerosis and intractable epilepsy (Kagawa et al. (2005). One major drawback, however, is that the radiosynthesis of AMT is complicated, so that its use is still restricted to specialized PET centers.
In summary, the application of PET/CT in the presurgical planning in epilepsy is well documented (Nooraine et al. 2013; Vivash et al. 2013; Kumar and Chugani 2013). Up-to-date data suggest that PET and MEG might be complementary and in combination yield increased sensitivity and specificity as compared to each technique applied on its own. For a review of the current knowledge concerning the usefulness of PET/CT and MEG for the presurgical assessment of MRI-negative epilepsy and the discussion of the impact of the combination of the results on the local seizure onset zone, see Rheims et al. (2013).
In presurgical planning of tumor resection, multimodal approaches using 18F-fluoroethyl-l-tyrosine PET/CT in combination with DTI for the detection of tumor invasion into the pyramidal tract in glioma patients with sensorimotor deficits (Stadlbauer et al. 2009) have been suggested.
Analyses from PET-guided resection of supratentorial high-grade gliomas have suggested a significantly better survival in patients with PET-guided volumetric resection and no postoperative residual tracer uptake as compared to MRI-guided volumetric resection with no residual postoperative contrast enhancement (Pirotte et al. 2009).
Additionally, in patients with central Cushing disease, 11C-methionine or 18F-tyrosine PET/CT co-registered to MRI has shown to significantly increase the accuracy of the presurgical localization and delineation of pituitary microadenomas.
2.3 Combination of MRI and MEG (MSI)/ EEG
Magnetoencephalography (MEG) is an analysis technique which uses electromagnetic signals to localize the source of biomagnetic fields derived from intracellular electric current flows in the brain, thus providing direct information about neural activity (Hamalainen et al. 1993). One of the most important advantages of MEG is that its temporal resolution is very high (i.e., in the order of 1 ms) and is mainly restricted only by the sampling frequency of the recording system (Lounasmaa et al. 1996). Since magnetic fields are not distorted by conduction inhomogeneities in the head, MEG is a potentially powerful tool for the localization of brain function, providing a spatial discrimination below 5 mm. As a major limitation, MEG has a fundamental source identification problem (inverse problem), by determining the active site within the brain from the magnetic field pattern recorded outside the skull. Thus, some a priori assumptions must be made about the source. Usually, signal generators in the brain are described as current dipoles which are physiologically reasonable models for relatively small active cortical areas. MEG results can be directly co-registered to 3-D MRI. The merging of the data obtained from MEG with anatomical MRI images, i.e., magnetic source imaging (MSI), allows the integration of the functional information as obtained from MEG into the structural information of MR (Orrison 1999).
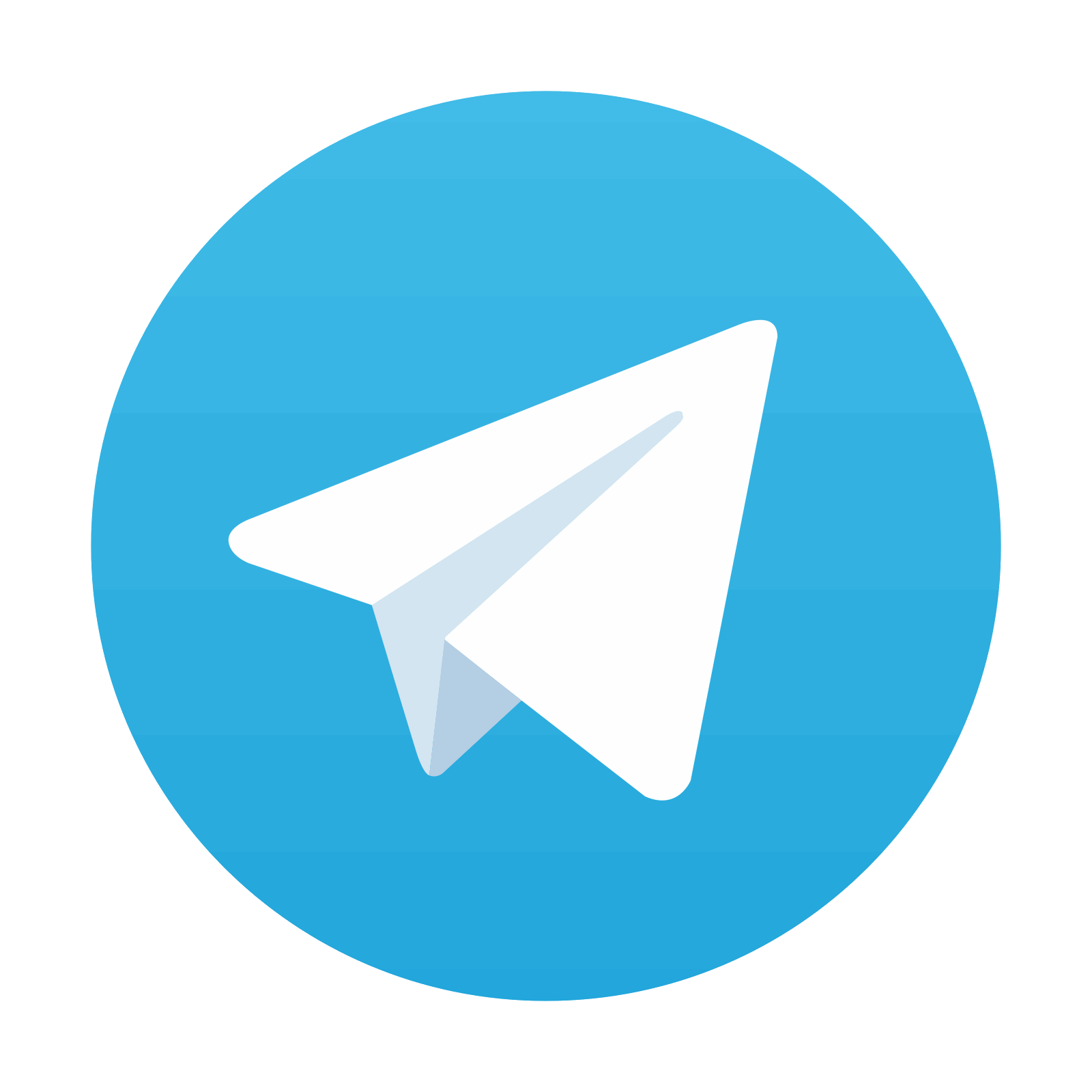
Stay updated, free articles. Join our Telegram channel

Full access? Get Clinical Tree
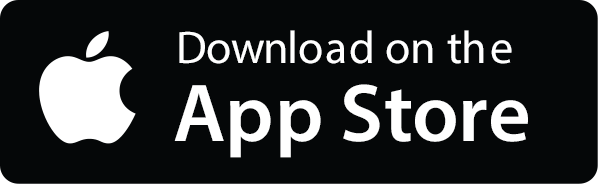
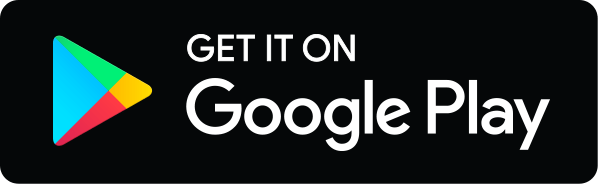