18 Since the initial development of intraoperative magnetic resonance imaging (iMRI) technology in the mid-1990s, a variety of MRI systems have evolved for use during neurosurgical procedures. The systems have ranged from low-field (0.12 tesla) to high-field (3.0 tesla) imaging magnets and have involved either operating within the magnetic field or outside the magnetic field.1–8 In general, the field strength of the iMRI system determines image quality and imaging sequences or software applications available to the neurosurgeon. A broad spectrum of neurosurgical disease has been monitored with iMRI, in particular neoplastic pathology; however, there is a distinct paucity of literature regarding the use of iMRI in the surgical treatment of cerebrovascular disease. The treatment of cerebrovascular disease has changed tremendously, relying heavily on advances in surgical technique, technology, and neuroimaging. Rigid cranial fixation without limiting head position is essential to allow maximal brain relaxation and to minimize brain retraction. A surgical microscope is a necessity and a microscope-interfaced surgical chair with mouth and foot controls is utilized by many cerebrovascular surgeons. High-resolution imaging modalities such as digital subtraction angiography (DSA), MRI/MR angiography (MRA), and more recently, rapid computed tomographic angiography (CTA) have tremendously improved the evaluation and treatment of cerebrovascular disease. Convergence of imaging modalities in the operating room (OR) is the natural next step toward refining and optimizing surgical treatment. Intraoperative technologies such as angiography, CT, ultrasound Doppler flow probes, neuronavigation, and near-infrared indocyanine green videoangiography (ICG) are among the techniques now available to the cerebrovascular surgeon. With the advent of MR systems in the OR, the utility of iMRI in treating vascular disease has been examined. Specific MR capabilities, such as MRA, diffusion weighted imaging (DWI), and diffusion tensor imaging (DTI), represent potentially powerful tools to aid intraoperative management of cerebrovascular disease. The cerebrovascular operations discussed in this chapter were performed at Barrow Neurologic Institute (BNI) in Phoenix, Arizona, or at the University of Calgary (UC), in Calgary, Alberta, Canada. The iMRI system at BNI utilizes a General Electric (General Electric Medical Systems, Waukesha, WI) Signa HD 3.0 tesla (T) imaging magnet, based on the principle of moving the patient to a stationary magnet for imaging. The system is located in a separate radiofrequency- (RF-) shielded room within the main neurosurgical operating theater area, which houses 11 neurosurgical ORs. It is directly accessible from two adjacent ORs and indirectly from the remaining nine operating theaters. The operating tables in the adjacent two rooms are equipped with a translating table top, which slides onto the mobile MR gantry. A Mayfield-type MRI-compatible skull fixation system is incorporated into the table top. For intraoperative imaging, the intubated patient is transferred, with or without the surgical site open, onto the mobile MRI gantry by sliding the table top onto the docked gantry. The gantry is then transferred to the MRI suite and docks to the MRI system for imaging. An MRI-compatible ventilator is utilized in the imaging suite. Following completion of imaging, the patient is returned to the operating table in a similar fashion. The GE HD 3.0 T system is equipped with high-power 23 to 50 mT/m gradients. Slew rate is 80 to 150 mT/m per millisecond. The magnet is 2.4 m tall, 2.2 m wide, and 2 m in length. It possesses a working bore of 60 cm and weighs 12,000 kg. The 5 gauss (G) fringe field is 2.8 × 5.0 m. The electronic OR table is custom made by Maquet (Rastatt, Germany), and possesses all of the positional capabilities of a standard OR table as well as standard head fixation attachments. The iMRI system at The University of Calgary was initially based on a movable prototype 1.5 T magnet4,9 and now a movable IMRIS 3.0 T magnet. The magnet (Siemens Verio 3.0 T, Erlangen, Germany) measures 1.73 m in length, with an internal diameter of 90 cm and a weight of 8,000 kg, compared with the prototype 5,100 kg 1.5 T system. The working aperture is 70 cm with shielded gradients in place. The 5 G fringe field measures only 4 m×3.5 m. The system provides a large homogeneous field of view measuring 50 × 50×50 cm at the isocenter, maximum gradient strength of 45 mT/m, and a slew rate of 200 mT/m per millisecond. When not needed for surgery, the magnet is housed in an alcove adjacent to the OR. For imaging, the magnet is moved into the OR and over the OR table with the aid of an electric motor and ceiling I-beam rails. The OR table and patient enter the magnet bore with the head of the table located in the center of the magnet. Once imaging is completed, the magnet is returned to the alcove. Frameless stereotaxy is utilized routinely at both sites. The Stealth Treon (Medtronic Navigation, Inc., Louisville, CO) stereotactic neuronavigation system is utilized in all BNI ORs and is integrated with the surgical microscope. In Calgary, a prototype ceiling-mounted BrainLab (BrainLab, Heimstetten, Germany) neuronavigation system was used. Intraoperative MR images at each site can be easily transferred to either the Stealth or BrainLab systems, and are utilized to update the navigation system to correct for brain and lesion deformation and shift as a result of surgery. All surgeries were performed with standard patient-positioning, skull fixation, microsurgical tools, techniques, and microscopes. Standard neuroanesthetic management was utilized. Aneurysm clipping was performed with commercially available standard titanium aneurysm clips (Spetzler, Yasargil, or Sugita). Controlled hypotension and temporary clipping was applied as required in the standard fashion. In this series, 20 patients harboring 23 aneurysms have had aneurysm clipping monitored with iMR imaging. All except two aneurysms were unruptured and were located throughout the cerebral circulation, 20 aneurysms involved the anterior circulation and three involved the posterior circulation (Table 18.1). In general, surgical planning iMRI showed the aneurysm and its relationship to conventional surgical corridors. Interdissection and quality-assurance MRA showed susceptibility artifact that prevented accurate assessment of the aneurysm neck. The desire to negate this observation has resulted in the development of MR invisible clips based on ceramic materials.10 In certain cases, aneurysm clipping was also immediately evaluated with ICG angiography, which demonstrated patency of daughter and parent vessels, and obliteration of the aneurysm. Quality-assurance iMRA obtained on these patients confirmed these findings. A superficial temporal artery to middle cerebral artery (STA-MCA) bypass was performed along with supraclinoid carotid ligation in a patient with a giant cavernous carotid aneurysm (Fig. 18.1). All vessels, including the bypass, were visualized, except the proximal internal carotid artery, which demonstrated the expected occlusion. All iMRA studies demonstrated significant susceptibility artifact at the clip site (Fig. 18.2). In another case, a ruptured anterior communicating artery aneurysm underwent clipping. At surgery a prominent perforator adherent to the dome and neck of the aneurysm could not be preserved. Diffusion weighted imaging did not demonstrate any significant ischemic deficit in this patient (Fig. 18.3). Hemorrhage was excluded in all patients prior to extubation. Seventy-one patients have had their cavernous malformation (CM) resection monitored with iMRI. Of these patients, 26 were located in the brainstem or diencephalon and the remainder were hemispheric, except for one orbital and one cervical cord lesion (Table 18.1). Surgical planning iMRI with or without surgical navigation was often utilized to accurately localize the CM, thereby optimizing craniotomy placement and surgical trajectory. Among the brainstem patients, inter-dissection MRI was used when needed to accurately localize the lesion in relationship to the planned brainstem entry site. Complete resection of the CM and absence of a postoperative hematoma was confirmed by the iMRI quality assurance imaging in all cases (Fig. 18.4). More recently, diffusion tensor tractography has been applied to patients with CM (Fig. 18.5). Fiber tracking demonstrates well the relationship of white matter tracts to both the surgical corridor and the cavernoma. This intraoperatively acquired knowledge makes the surgeon aware of the location of important structures to be avoided during the resection of these vascular malformations. Thirty-two patients with an arteriovenous malformation (AVM) of the brain were monitored with iMRI. These AVMs were distributed in a typical fashion throughout the cerebral hemispheres and cerebellum. In general, surgical planning iMRI with or without surgical navigation optimized craniotomy placement, whereas quality-assurance iMRI confirmed complete resection of the lesion and excluded acute surgical complications such as hemorrhage or cerebral edema.
Intraoperative Magnetic Resonance Imaging and Cerebrovascular Surgery
Clinical Experience
Intraoperative MRI Systems
Vascular Lesions
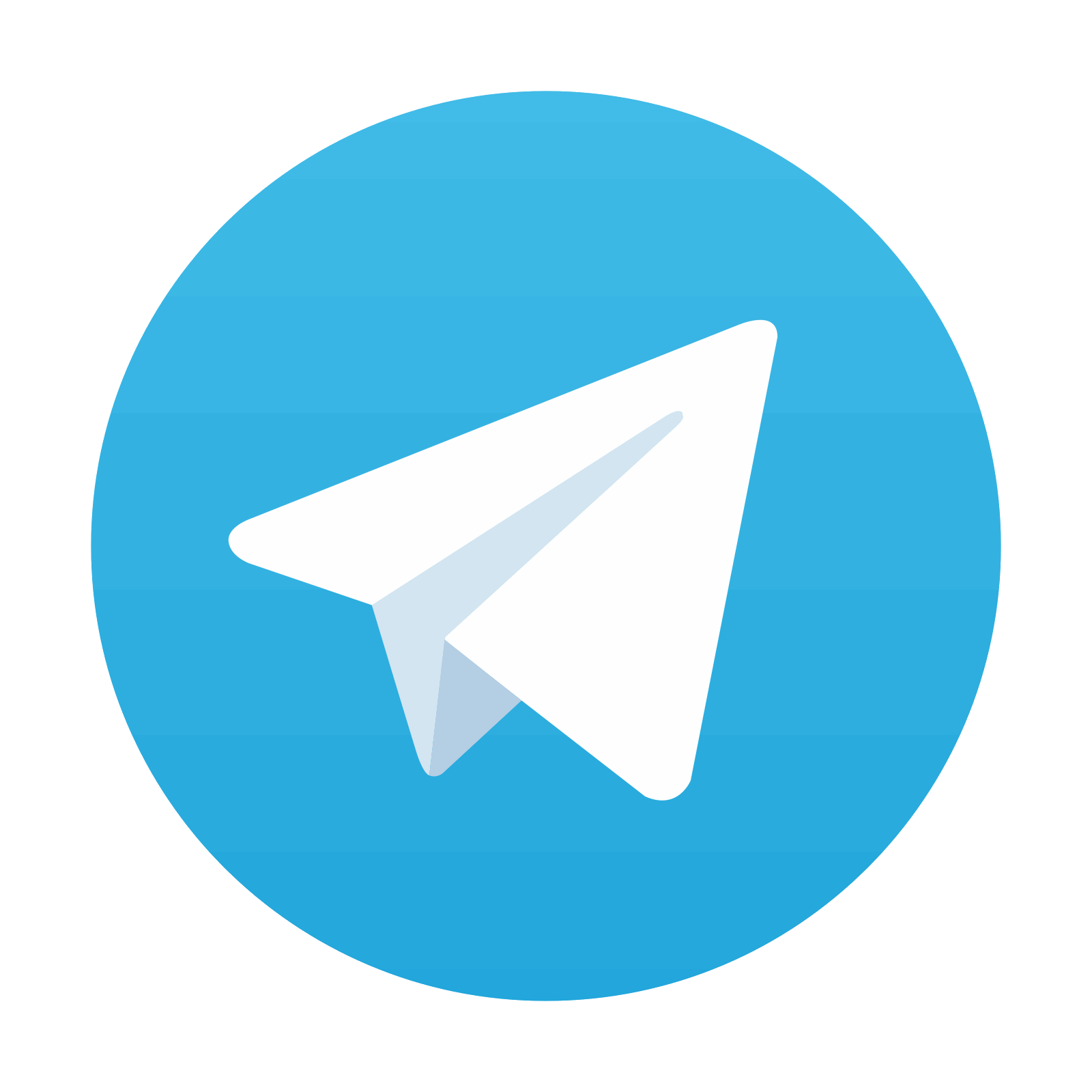
Stay updated, free articles. Join our Telegram channel

Full access? Get Clinical Tree
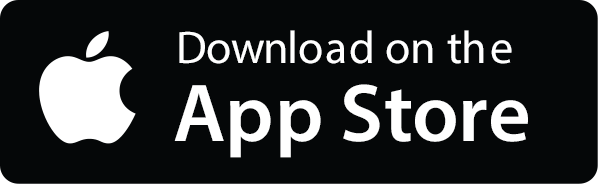
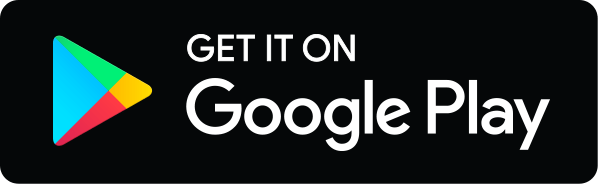