11 Intraoperative Ultrasound Intraoperative ultrasound is a dynamic and highly interactive imaging study and is one of the most rapidly developing areas within ultrasonography. Unfortunately, many radiologists have been reluctant to spend a significant portion of time out of the department during the workday to perform and interpret intraoperative ultrasound studies, fearing that they will lose 1 or 2 hours of work time while waiting in the operating suite until the surgeon is ready for the scans to be performed. However, the information obtained during intraoperative ultrasound is often crucial for accurate diagnostic assessment and planning of surgical approaches to resection of the disease processes. Studies have shown that the impact of intraoperative ultrasound imaging on surgical decision making justifies the time and effort involved in terms of both efficacy and costbenefit.1 Our opinion, as well as that of many others with experience in the field, is that the benefits gained by intraoperative ultrasound imaging justify the time spent by the radiologists performing the procedure. We have evolved several means to improve the radiologists’ efficiency when performing intraoperative ultrasound scans. These strategies typically allow a radiologist to perform the intraoperative ultrasound and return to the radiology department in ~30 minutes. The most effective strategies are as follows: 1. Intraoperative ultrasound studies are booked in advance with the ultrasound section whenever possible, which allows more planning and manipulation of the work schedule within the ultrasound section, and anticipation of the approximate time for performing the study. 2. Prepositioning of the intraoperative ultrasound scanner in the operating suite in advance of the examination may save a few minutes’ waiting time for elevators. 3. The radiologist who will perform the examination may choose to work in surgical scrubs, thereby eliminating the need to change into scrubs when the call from the operating room arrives. 4. Mutual cooperation and respect between the surgeons and radiologists has resulted in an agreement that the surgeons will call for the intraoperative ultrasound scan 10 to 15 minutes before they are actually ready for scanning, and the radiologists and technologists guarantee their readiness to perform the scan within 10 to 15 minutes of the telephone call. This arrangement is usually successful in avoiding any unnecessary waiting by either the surgical team or the radiological team and thus maximizes efficiency. In our institution, the radiologist scrubs in on the case and is gowned and gloved and performs the actual scanning. A typical intraoperative study can be completed within 5 to 10 minutes, assuming that the sonologist is experienced. Scanning performed by the surgeon with the radiologist observing at the bedside or by remote teleradiography is less optimal compared with the radiologist actually performing the scan. Scanning provides important hand-to-eye information, and the scanning technique of most surgeons cannot compare with that of a skilled and experienced sonologist. Even though intraoperative ultrasound scanning has removed many of the noise-generated barriers to excellent image quality, proper scanning technique, understanding of image artifacts, and recognition of subtle findings such as isoechoic tumor nodules are best achieved by someone with extensive experience in ultrasonography. In a busy hospital setting, with frequent utilization of intraoperative ultrasonography, a remote teleradiography linkage to the intraoperative ultrasound images may be an acceptable alternative, providing that the surgeon performing the intraoperative scans has sufficient experience at intraoperative scanning. If possible, it is optimal to sterilize the intraoperative ultrasound probes before the operation. We have had excellent success using gas sterilization with ethylene oxide for many of our intraoperative probes. However, many manufacturers are reluctant to allow gas sterilization, fearing that the transducers will be damaged by the high temperatures of aeration that are required by ethylene oxide gas sterilization, although we have not experienced any problems. Some operating suites will allow prolonged immersion in Cidex (glutaraldehyde; Johnson & Johnson, Arlington, Texas) as an adequate method of probe sterilization, but other institutions do not consider this sufficiently sterile for open intraoperative use, and there have been some adverse patient contrast reactions to the glutaraldehyde if it has not been sufficiently rinsed prior to patient exposure. In our institution, direct patient contact with glutaraldehyde-soaked equipment is not allowed, and therefore sterile probe covers are utilized. The application of probe covers may add 1 or 2 minutes to the time of the procedure as the cover is being applied, and there is some risk of compromise of sterile technique should the probe cover rip, which can occur occasionally. Therefore, when using equipment with sterile probe covers, we soak the probes in glutaraldehyde for 30 minutes before the procedure in case there is a break in sterile technique. The probes are thoroughly rinsed and, as an additional precaution, sterile gel is used inside the probe cover. It is preferable to use specifically designed transducer sheaths that fit snugly over the transducer head because loose-fitting covers may cause imaging artifacts due to trapped gas or folds in the sheath. Standard endoscopic sheathes can be used to cover the entire transducer cord. Our preferred method of sterilization currently utilizes the Sterrad system (Advanced Sterilization Products, Irvine, California), which is gas-plasma technology using low-temperature sterilization, thereby avoiding some of the problems with the high-temperature ethylene oxide systems. Sterilization is adequate to allow direct patient contact with the probes, thereby avoiding the necessity for probe covers. There are no hazardous emissions and, hence, sterilization time is shorter because prolonged aeration and ventilation are not required. Intraoperative ultrasound has many uses, and the applications are extensive and growing. In neurosurgery, intraoperative ultrasound is used effectively in surgery on the brain and spinal cord, and, in intraabdominal surgery, the uses are principally in the liver, biliary tract, and pancreas. Intraoperative assessment of vascular surgical disease and intraoperative ultrasound imaging post endarterectomy or reconstructive procedures is now on the increase. Other newly developing areas of use include intraoperative localization of breast tumors; applications in the genitourinary systems, such as evaluation of small renal cell carcinomas; as well as in gynecologic surgery; and, finally, to provide guidance for interventional procedures, such as prostate cryosurgery and tumor ablations in the liver. One of the most exciting and rapidly developing new areas is that of laparoscopic ultrasound (LUS) imaging, which is being applied to assessment of diseases of the liver, pancreas, and biliary tract within the abdomen and has also been used to help detect lung tumors during thoracoscopic resections. Another new and exciting application is the use of catheter-mounted, high-frequency ultrasound transducers for endoluminal intraoperative use in the bile ducts and ureters, for gynecologic procedures, and for vascular intraluminal assessment. Given the limitations of space, this discussion focuses on selected neurosurgical and intraabdominal applications. Intraoperative ultrasound scans of the brain can be obtained through a burr hole, using specially designed small burr hole probes or endoluminal probes, such as are used for prostate or transvaginal scanning. More commonly, intraoperative ultrasound scans are obtained through an open craniotomy flap. Excellent images can be obtained transdurally as well as directly on the brain surface after incision of the dura. The optimal frequency for brain imaging ranges from 5 to 7.5 MHz in frequency, and the best probe configuration is found in the endfire sector type probes, either mechanical or electronic convex array or phased array probes. The dura or brain surface is moistened with a small amount of sterile saline solution, which provides acoustic coupling for the transducer. Meticulous scanning technique is essential, with particular care to avoid applying significant pressure on the brain. A very light contact with the moistened dura or brain surface is sufficient for adequate acoustic coupling. The principal use of intraoperative ultrasound is to accurately locate and localize masses within the brain substance that cannot be visualized directly by the neurosurgeon, and of course the brain cannot be palpated. Even masses a few millimeters deep to the cortical surface are difficult or impossible to detect visually. The vast majority of primary and metastatic brain tumors are markedly hyperechoic in comparison with the surrounding normal brain structures.2 The sulcal convolutions on the brain surface are somewhat echogenic, but most of the brain substance is of relatively low and homogeneous echogenicity. Consequently, most tumors stand out dramatically as hyperechoic lesions against a relatively hypoechoic background (Fig. 11–1). The reactive edema associated with brain tumors can decrease even further the echogenicity of brain substance and increase the conspicuity of focal masses.3 Meningiomas are the most highly echogenic primary brain tumors, and usually have a relatively smooth contour and sharp margination. Calcifications within meningiomas occur frequently and result in a further increase in echogenicity. Glioblastomas are also markedly hyperechoic and are often well marginated, but may have less well-defined margins when they are aggressive and invasive.4 Cystic degeneration may occur in glioblastomas as well as in cystic astrocytomas (Fig. 11–2), and the septations, cyst cavities, and areas of solid tumor and mural nodularity are well depicted by intraoperative ultrasound.5,6 The complex nature of these cystic neoplasms is more completely portrayed with intraoperative ultrasound than with other imaging modalities, including computed tomography (CT) and even magnetic resonance imaging (MRI). Complete definition of the various spaces and cystic compartments may be important to guide surgical decompression of cystic tumors by aspiration. Most brain metastases are also hyperechoic and well circumscribed (Fig. 11–3), with an appearance similar to meningiomas and gliomas.7 Liquefaction necrosis, which may occur spontaneously or as the result of therapy, may diminish the echogenicity of tumors centrally (Fig. 11–3). Low-grade astrocytomas can present a much more difficult imaging problem because they tend to be less echogenic and also to have very poorly defined infiltrative margins, insinuating into the adjacent brain substance in a very ill-defined manner.8,9 In addition, chronic edema associated with low-grade astrocytomas may actually increase brain echogenicity adjacent to the tumor, thus making tumor margins even more poorly defined.10 Figure 11–1 Hyperechoic glioma. Ultrasound scan shows a glioma (arrows) in the occipital lobe. Figure 11–2 Predominantly cystic astrocytoma. Ultrasound scan clearly shows mural nodularity and septation. Figure 11–3 Poorly differentiated brain metastasis from primary lung carcinoma. Ultrasound scan shows an echogenic rim, but the central portion is hypoechoic due to liquefaction necrosis. Note the gyral and sulcal detail, which is obliterated by brain edema surrounding the tumor nodule. In addition to defining the tumor site and assessing margins, intraoperative ultrasound is helpful to the neurosurgeon in selecting an approach to resection of the tumor that will hopefully minimize damage to surrounding functional brain tissue. Following resection, intraoperative ultrasound can be utilized to evaluate completeness of surgical resection by rescanning after filling the surgical cavity with sterile saline and seeking any residual tumor nodules. Intraoperative ultrasound imaging is also very helpful in guiding biopsy procedures, again with the goal of minimizing trauma to adjacent brain tissues.11 Specially designed probes can be used through a modified burr hole to allow precise needle placement with minimal patient trauma.12 Biopsies can be performed with electronic real-time biopsy guides, or freehand under direct real-time visualization.13 High-resolution endfire endoluminal probes have been quite useful to perform accurate real-time biopsies through small craniotomy sites utilizing electronic biopsy guidance (Fig. 11–4). Utilization of color flow imaging may help to choose a path for the biopsy needle that will minimize disruption of major blood vessels between the cortical surface and the tumor site. Real-time guidance can also be successfully used for tumor ablative techniques, placement of ventricular shunt catheters, and drainage of intracranial fluid collections and abscesses (Fig. 11–5).14 Following ultrasound-guided needle biopsies, we routinely rescan the patient for several minutes after the biopsy to assess for any bleeding within the lesion. Acute bleeding may appear as a small, highly echogenic focus within the mass or in the surrounding brain parenchyma. Occasionally, fluid/fluid levels may be seen, particularly when there is bleeding into a mass with cystic degeneration (Fig. 11–6). Scanning for several minutes is usually sufficient to ensure that any bleeding has stabilized. Figure 11–4 (A) Left temporal glioblastoma (arrows). (B) Color flow imaging helps target a safe, less vascular path for biopsy. The linear cursor (arrow) demarks the planned position of the biopsy needle. Gray-scale, Doppler, and color flow imaging have been used in neurosurgical approaches to aneurysms and arteriovenous malformations (AVMs).15 Assessment of blood flow is important during clipping or resection of aneurysms. Many AVMs are poorly visualized on gray-scale intraoperative ultrasound imaging, but the use of color flow imaging has been very helpful in defining their location and boundaries. Figure 11–5 Frontal lobe abscess. Ultrasound scan shows an echogenic rim and centrally located, moderately echogenic purulent material. Ultrasound-guided aspiration and evacuation of the abscess was performed. Many neurosurgical procedures now utilize frame-based or nonframe-based image guidance systems utilizing preoperative image information derived from MRI or CT scans, as a guide to surgical exploration and resection. However, brain tissue movement during surgery can be a significant source of error during image-guided surgical interventions. To compensate for this tissue motion, intraoperative ultrasound has been utilized to detect brain deformation during neurosurgery. Interactive image overlay systems allow projection of the region of interest on intraoperative ultrasound to overlay an associated MR image, helping the surgeon correlate the ultrasound anatomy to the more familiar MR images. The small discrepancies between the ultrasound and preoperative MR images can thus be utilized to assess tissue motion during neurosurgery.16 Indeed, even today, there are advocates for intraoperative ultrasound as a much simpler, less expensive, and equally efficacious technique for guiding intraoperative procedures in comparison with the elegant, but highly complex and exceedingly expensive intraoperative MRI systems.17 Figure 11–6 Astrocytoma. Ultrasound scan shows fluid-blood level (arrow) within the cystic cavity of an astrocytoma after needle biopsy. Figure 11–7 Thoracic cord. Sagittal ultrasound scans show the central spinal canal (arrow) as a paired linear echogenic structure. The dura (arrowheads) is well seen posteriorly and anteriorly. Figure 11–8 Transverse ultrasound scan of the cervical cord demonstrates dentate ligaments (arrows) and several nerve roots cut in cross section (arrowheads) within the posterior dural sac. The uses of intraoperative ultrasound imaging in the evaluation of spinal cord abnormalities are similar to those for brain imaging. Endfire probes are essential and the optimal frequency would be in the 7 to 10 MHz range, although 5 MHz probes can be successfully utilized. Electronic curved array or linear array probes are preferable because they allow concomitant utilization of color flow imaging, but mechanical endfire probes can also be utilized. The surgical laminectomy site is filled with sterile degassed saline to provide an acoustic coupling medium. The probe is then placed onto the pool of degassed saline solution. Images are usually obtained through the dura without direct contact with the spinal cord. Both axial and sagittal planes are utilized. The spinal cord itself has a relatively low to moderate echogenicity and is quite homogeneous in texture.18 The central spinal canal or indentation of the cord at the site of the central canal can be visualized as an echogenic single or paired set of lines relatively central within the spinal cord, which is otherwise featureless (Fig. 11–7). The central spinal canal is an important normal landmark that is frequently disrupted by pathological conditions within the cord. The arachnoid membrane is difficult to visualize, but the dura is well seen both dorsally and ventrally, and a small amount of fluid is usually present between the dura and the arachnoid. The dentate ligaments are well visualized in axial views (Fig. 11–8). They arise from the dorsal and lateral margins of the sac and extend laterally to the adjacent spinal canal. Nerve roots are inconsistently imaged in the cervical and thoracic spine, but are well seen at the conus medullaris and distally in the region of the cauda equina. Nerve roots appear to be hypoechoic or anechoic, but are readily visualized within the spinal fluid by highly reflective dorsal and ventral margins, appearing as two parallel echogenic lines. Pulsations can be imaged in real time from the anterior spinal artery, which is deep to the cord. Color flow imaging and power Doppler imaging will show the vascularity in a more complete fashion. Assessment of the location and extent of masses is one of the principal uses of spinal intraoperative ultrasound. Lesion location can be assessed as intramedullary or extramedullary, and intradural and extradural components can be evaluated.19 This capability can be particularly useful in depicting tumors such as neurofibromas, which may have both intradural and extradural components. The spinal cord cannot be retracted extensively, and intraoperative ultrasound imaging can provide important information as to the full extent of such tumors.20 Many intramedullary tumors of the cord, including ependymomas, dermoids, and many metastases, are hyperechoic. Astrocytomas, however, are frequently isoechoic with extremely ill-defined margins, and consequently are very poorly visualized. These lesions may be recognized by effacement of the landmark echoes from the central spinal canal, as well as by fusiform swelling of the cord. Other nonneoplastic inflammatory and posttraumatic conditions can also cause swelling of the cord and may not be readily distinguishable from astrocytomas.21 Astrocytomas may show cystic degeneration, which can also be seen in ependymomas and hemangioblastomas (Fig. 11–9). These tumors can form fairly large cystic cavities and simulate syringomyelia. However, the presence of solid masses, mural nodules, or irregularly thickened septations will help to distinguish cystic tumors from a benign syrinx.22,23 Figure 11–9 Hemangioblastoma of the cervical cord. Ultrasound scan shows multiple cystic components and interruption of the central spinal canal (arrow), which indicates the presence of an intramedullary mass. Extramedullary tumors include meningiomas (Fig. 11–10), neurofibromas, lipomas, and dermoids, as well as malignant tumors, which are most often metastatic. Other conditions can also produce an extramedullary mass effect, including protruding discs, bony lesions (spurs and fracture fragments), hematomas, abscesses, and arachnoid cyst. Most of the neoplastic lesions appear as moderately echogenic masses with displacement or compression of the adjacent spinal cord and displacement of other structures, including nerve roots. Bony spurs and fragments are highly echogenic and may be associated with acoustic shadow. Herniated disc fragments are moderately echogenic, but substantially less so than bony spurs.24 Hematomas appear hyperechoic acutely,25 but may have a variable appearance, depending on their age and degree of liquefaction, whereas most abscesses and cysts have predominantly fluid components and increased transmission of sound through the fluid. Intraoperative ultrasound is very useful in evaluating the extent of surgical resections,26,27
Technique for Efficient Performance of Intraoperative Ultrasound
Application of Intraoperative Ultrasound
Neurosurgical Applications
Brain
Spine
Stay updated, free articles. Join our Telegram channel

Full access? Get Clinical Tree
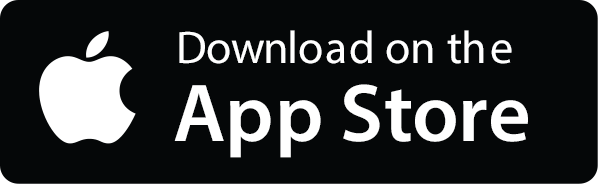
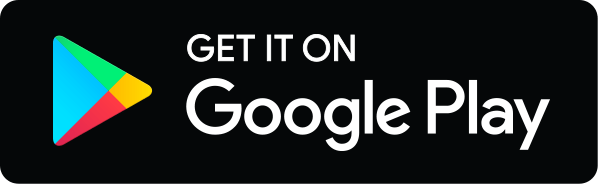