Alioscka A. Sousa and Michael J. Kruhlak (eds.)Methods in Molecular BiologyMethods and ProtocolsNanoimaging2013Methods and Protocols10.1007/978-1-62703-137-0_1© Springer Science+Business Media, LLC 2013
1. Introduction: Nanoimaging Techniques in Biology
(1)
National Institute of Biomedical Imaging and Bioengineering, National Institutes of Health, Bethesda, MD, USA
(2)
Experimental Immunology Branch, National Cancer Institute, National Institutes of Health, Bethesda, MD, USA
Abstract
To dissect the astonishing complexity of the biomolecular machinery functioning within a cell, imaging has been an integral tool in biology, allowing researches to “view” the detailed molecular biology responsible for coordinating cellular life. To visualize the molecular components of cellular structures requires, in particular, imaging techniques capable of reaching nanoscale spatial resolutions. Such nanoimaging techniques are the focus of this volume. Chapters in the current volume are divided into four parts and include specialized techniques in the areas of light, electron, and scanning probe microscopy, as well as methodologies employing combinatorial and complementary imaging approaches.
1 Introduction
Despite the diverse physiological properties of different cell types, they are composed, generally, of the same biochemical and molecular components. The coordinated assembly of these components provides the functional characteristics of the cell and, ultimately, the viability of the organism. A common goal in biology is, therefore, to dissect the astonishing complexity of the cellular molecular machinery, seeking to understand how individual components of this machinery assemble to form higher-order structures, navigate the cell with synchronized dynamics, and interact to produce a given biological outcome. To realize this common goal, imaging has been an integral tool in biology, allowing researches to “view” the detailed molecular biology responsible for coordinating cellular life.
To directly observe the molecular components of a cell, either in isolated form or within a cellular context, biologists have at their disposal an array of established techniques in the major areas of light, electron, and scanning probe microscopy. While pivotal changes in biology have repeatedly occurred following key developments in microscopy, the quest to explore the inner workings of cells at ever-increasing levels of detail has been, at the same time, a tremendous stimulus to the development of novel and ingenious approaches to imaging. Thus, to some extent, biology has led to a continuous reinvention of the light, electron, and scanning probe microscopes, in addition to nurturing the development of completely new tools in microscopy. Today, advanced imaging modalities enable the visualization of biological specimens at a range of length scales and resolutions, in multiple dimensions (both time and space), with chemical or molecular specificity, and from the surface or bulk of the specimen, revealing the secrets of the molecular bionetwork within the cell.
Starting with the optical microscope, one of the most important historical developments in this field can be assigned to Ernst Abbe, whose rigorous description of wave-based optical theory supporting image formation in the microscope provided the rationale for the limitation of resolution and, thereby, the expected barrier to observing fine details of the molecular machinery within a specimen. The resolution limit as imposed by diffraction still holds true, but advances in instrumentation and a greater understanding of the physical photochemistry of labeling fluorophores have allowed microscopists to achieve resolutions beyond Abbe’s diffraction limit. This includes the recent development of numerous ingenious super-resolution optical imaging methods that reveal specimen details on the nanometer scale.
The legendary work by Ernst Ruska and colleagues used Abbe’s wave-based optical theory to great advantage in developing the electron microscope. The dramatically shorter wavelength of electrons employed in the electron microscope allowed biologists for the first time to examine the internal architecture of cells with nanoscale spatial resolution. The conventional view of electron microscopy, however, is that thin and exhaustively processed specimens can be observed only with rather nonspecific contrasting agents, thereby limiting the specificity of the molecular information obtained. Modern electron microscopes are based on Ruska’s prototype but have developed specialized imaging arrangements that provide greater contextual information about the sample while overcoming some of the traditional limitations of specimen preparation. The diverse modes of operation of the modern electron microscope are able to provide images of native cellular structures, in three dimensions, from large sample volumes, and containing relevant quantitative information.
Contrary to light and electron microscopy in which a magnified image of a specimen is generated by a lens-based optical system, techniques in scanning probe microscopy rely on the general principle of raster-scanning a sharp probe across a surface to produce a magnified view of the specimen topography. The hallmark scanning probe instrument is the atomic force microscope (AFM) that differs significantly from conventional lens-based microscopes in the underlying principle of image formation, and not surprisingly offers its own set of attributes and opportunities, such as the possibility to probe specific chemical and biophysical interactions between a functionalized scanning tip and the underlying sample surface. Near-field scanning optical microscopy (NSOM) is another type of scanning probe technique of current use in biology. It involves scanning with a small probe positioned less than a wavelength of light from the sample whereby light is transmitted through a small aperture in the probe tip, thus providing a means to circumvent the diffraction-limited far-field resolution of optical microscopes.
Several other specialized nanoscale imaging techniques exist that do not fall within the more general categories of light, electron, and scanning probe microscopy as highlighted above. One such technique, soft X-ray microscopy, has a history that dates back several decades, although only more recently has it become more adept at imaging biological specimens. Today, soft X-ray tomography is emerging as a powerful method to visualize entire eukaryotic cells in three dimensions with a spatial resolution that bridges that of the light and electron microscope. Another specialized technology, imaging mass spectrometry, combines attributes of both secondary ion mass spectrometry and imaging. This relatively new technique offers the exciting possibility of obtaining very specific compositional information about a sample’s surface with a lateral resolution in the tens of nanometers.
A feature common to all imaging techniques is the need to produce contrast, and in fact the success of some of the most modern techniques relies on ingenious ways in which structures can be induced to reveal themselves. Of course, an important characteristic of any imaging modality is the achievable spatial resolution, and here a diverse range of methodologies exist that cover resolutions from the nano to the macro length scales. To visualize cellular molecular components and substructures requires in particular imaging techniques capable of reaching nanoscale spatial resolutions. Such nanoimaging techniques are therefore the focus of this volume.
Chapters in the current volume are divided into four parts and include specialized techniques in the areas of light, electron, and scanning probe microscopy (Parts 1, 2 and 3, respectively), as well as methodologies employing combinatorial and complementary imaging approaches (Part 4). Nanoimaging of biological samples involves precise logistical considerations that are more acute than for conventional imaging, such as illumination timing and intensity, signal information sampling, specimen preparation and labeling, to list a few. Given the added complexity of imaging biological specimens on the nanometer scale, we hope that the chapters comprising this volume provide valuable information from successful application of a broad range of important imaging methods for the nanoscale visualization of biological structures.
2 Part 1: Light Microscopy
Light microscopy is invaluable to biologists, but its application to resolving molecular details is limited by the physical properties of light, principally the diffraction of light upon passing through a fixed aperture. Diffraction of light results in the blurring of a point object in the image and is dependent on the wavelength of light and the numerical aperture of the collecting lens. The expanded observed intensity profile of the point is known as the point spread function, with the actual object located at the center of the intensity profile. The dimensions of the point spread function are larger than the physical size of the individual molecular components within the specimen. To truly image specimen molecular details, the diffraction barrier needs to be surpassed. A collection of super-resolution optical imaging methods have been able to attain resolutions beyond the diffraction limit by employing sophisticated, and elegant, illumination parameters, optical configurations, or fluorophores with unique biophysical properties. A number of methods exploit nonlinearity in the reflected light from the specimen and often employ a timing mechanism to achieve the improved resolution.
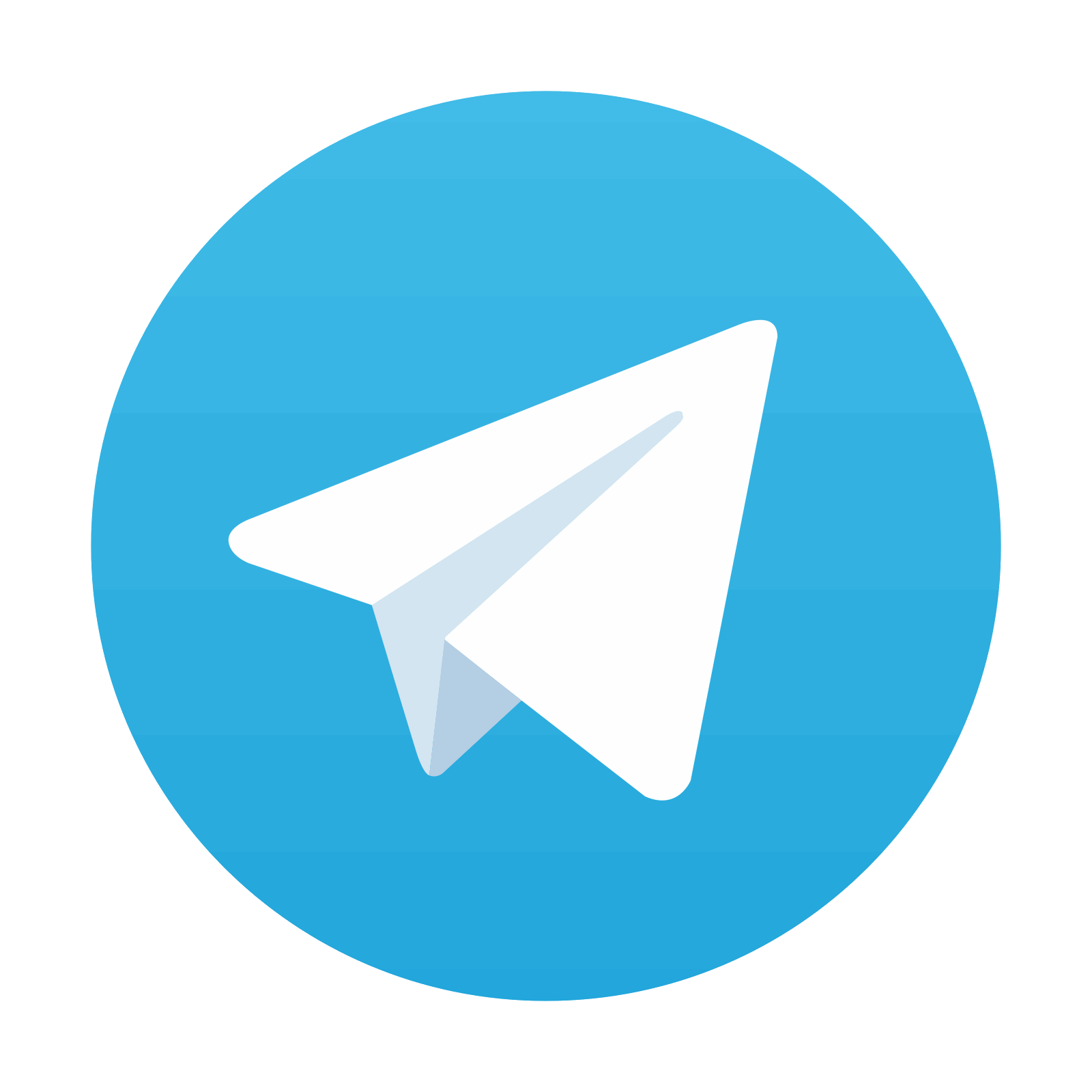
Stay updated, free articles. Join our Telegram channel

Full access? Get Clinical Tree
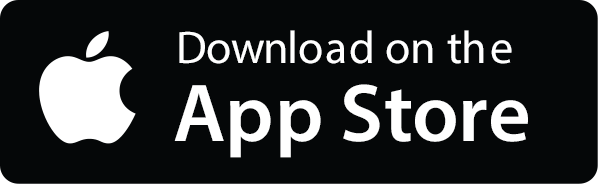
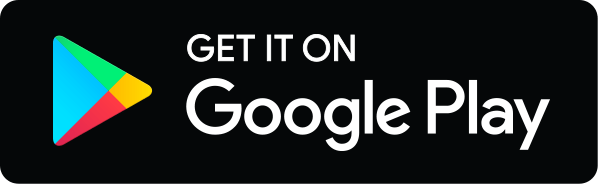