Introduction to Radiography, Safety, and Student Success
OBJECTIVES
After studying this chapter, the student will be able to:
1. State who discovered x-rays in addition to when and how they were discovered.
2. Identify the different persons who play an important role in the history of radiology.
3. Describe the technologic advances made since the discovery of x-rays.
4. Explain the biologic effects of radiation.
5. Describe how to protect against radiation.
6. Define general positioning terminology.
7. Describe foundations for student learning achievement.
8. Identify best study tips and best practices for student success.
KEY TERMS
ALARA: Radiation protection standards that state radiation must be As Low As Reasonably Achievable
Anterior posterior (AP): The central ray entering the patient’s anterior surface and exiting the patient’s posterior surface
Central ray (CR): The center or midpoint of the x-ray beam
Crookes tube: A partially evacuated glass tube in which electrons can flow from the cathode to the anode
Emotional: Subject to or involving emotion or emotions; emotion is a generalized feeling or feelings
Genetic effects: Radiation damage that occurs to persons whose ancestors were irradiated
Kinesthetic learners: Learn through doing and touching
Posterior anterior (PA): CR entering the patient’s posterior surface and exiting the patient’s anterior surface
Somatic effects: Radiation damage that occurs to the person who received the radiation
Stress: Physical, mental, or emotional strain or tension
Whole body: Includes the trunk, arms above the elbows, and legs above the knees. It is used for purposes of radiation exposure doses
Welcome to the world of diagnostic imaging technology. As you enter the classroom and begin your course of study, you will often wonder what culture and language you will encounter on the day’s class session’s presentation of the course’s(s’) content lesson. If you are studying radiologic sciences, you might believe that learning a new language might indeed be easier. The challenges that await you over your next 2 years may be intimidating, but they can be overcome with due diligence and an eye on the goal of becoming a licensed health care professional.
This chapter is an introduction to some of the elements that you will encounter in other classes that are more specifically related to the in-depth study required to pass the American Registry of Radiologic Technologists (ARRTs) examination. The remainder of the book is devoted to different types of patients that you will encounter in the imaging department and how to care for them in a compassionate and professional manner.
Now it is time to settle in and begin. Patient care will become natural to you when you realize that it is the same “language” you already speak, except for a little “adjustment to the grammar.”
IN THE BEGINNING
Although one man is credited with the discovery, there were actually many pioneers who paved the way by using equipment and making improvements on the equipment that eventually led to the actual discovery.
In the early 19th century, many scientists were experimenting with electricity (discovered by Benjamin Franklin) and little else. William Watson demonstrated electrical current. Michael Faraday induced electricity by passing a magnet through the magnetic field of a coil of wire. This led to experimentation with electromagnetic induction and the advent of generators and transformers with higher voltages.
A scientist named William Goodspeed was experimenting with cathode rays and the different energies that they emit. He made the actual first radiograph on February 22, 1890; however, he did not publish his work and was therefore not credited with the actual discovery of x-ray. In the mid-1890s, Sir William Crookes, an English physicist, was studying gas, and in order to continue with his work, he created a vacuum tube. It was a glass tube that contained both positive and negative electrodes and an induction coil (Fig. 1-1). He passed high-voltage electrical currents through the tube, which would allow him to study the conductivity of gases that had been put into the tube. Crookes was able to demonstrate that matter emitted from a cathode-ray tube had enough energy to turn a wheel that was located in the tube. Shortly afterward, Philipp Lenard, a German scientist, discovered the cathode ray while he was working with a Crookes tube. Lenard changed the original tube to include an aluminum window that allowed the cathode rays to pass through to the outside. During one of his experiments, a piece of barium platinocyanide soaked paper glowed when the rays were directed at it. Unfortunately for him, Lenard failed to further investigate what made the paper glow, thus allowing Wilhelm Conrad Röntgen to make the discovery only a few months later.
![]() FIGURE 1-1 An early tube created by Dr. Crookes and used by scientists to investigate the nature of light. |
A New Kind of Ray
The discovery of x-ray has had a significant effect not only on medicine but also on travel, the food industry, industrial equipment, sterilization of products and insects, and other commercial uses.
Dr. Wilhelm Conrad Röntgen was not the model of scientists. He never received his high school diploma, was well known to be absentminded, and would spend his time alone rather than with others in the pursuit of science. Röntgen was an assistant to Dr. August Kundt, a physicist at the University of Zurich. Later, he became an instructor at the University of Strasbourg. Although he was not well thought of as an instructor, he enjoyed and excelled at creating laboratory experiments that held outstanding results.
In 1888, Röntgen was offered the job of head of the physics department at the University of Wurzburg in Germany. He kept up his projects of cathode ray experiments with the Crookes tube after he accepted this offer of employment. On the evening of November 8, 1895, Röntgen was working with his Crookes tube in his basement laboratory. The tube was sitting on a bench, and across the room lay a plate that had the letter “A” painted on it with barium platinocyanide. The lights were dim so that he could see any change in gas color or electric current through the tube. When an electric current was passed through the tube, Röntgen noticed that the plate
on the opposite side of the room was “glowing” and he could readily see the letter “A.” Röntgen knew that this was not from any kind of light or electricity but had to be caused by something coming from the tube. Röntgen did not know what he had produced, but only that it was some type of ray, which he named the “X”-ray because the mathematical symbol for the unknown is “X.”
on the opposite side of the room was “glowing” and he could readily see the letter “A.” Röntgen knew that this was not from any kind of light or electricity but had to be caused by something coming from the tube. Röntgen did not know what he had produced, but only that it was some type of ray, which he named the “X”-ray because the mathematical symbol for the unknown is “X.”
Continuing with his experimentation over the next 6 weeks, he was able to reproduce the fluorescence with each passage of current through the tube despite the various objects that were placed between the plate and the tube. One of the things that were placed in front of the plate was a wooden box with metal weights inside. The ray did not pass through the entire box, but left a shadow on the plate. In December, Röntgen held a metal pipe in front of the plate and noticed the fingers of his hand were reflected on the screen. It was at this time that he persuaded his wife to allow him to take the now famous radiograph of her hand. On December 28, 1895, Röntgen submitted his report called “On a new kind of rays” to the Wurzburg Physico-Medical Society. Wilhelm Röntgen received the first ever Nobel Prize in Physics in 1901 for this world-changing discovery. He died in Munich on February 10, 1923, just a month shy of his 78th birthday.
Early Uses
In those early years, the scientific world talked of nothing but x-ray and cathode-ray tubes. Thomas Edison was just as caught up in the mania as everyone else. In 1896, he developed a handheld Crookes tube with a screen at one end and an eyepiece at the other. He called this new invention a Vitascope. It was the first fluoroscope and the forerunner of all of today’s fluoroscopy tubes.
Everyone wanted in on the excitement. Demonstrations were held in Bloomingdale’s and Macy’s in New York City; entertainment came in the form of x-ray machines that allowed the patron to view the bones of his or her hand for a coin put in the slot. Portrait studios offered x-rays of hands entwined to newly married couples to give as wedding souvenirs. At this time, the dangers were not known, and the novelty of seeing bones of the body continued to increase the demand for the phenomenon. Even the spiritual world used x-ray as a link to the other side and the fourth dimension. After the discovery of radium by Marie Pierre Curie in 1898, the upper crust of society would throw radium parties where the cocktails were laced with radium, the lights would be lowered, and the guests could watch their drinks glow in the dark!
X-ray was touted to cure cancer and skin lesions and was used to remove facial hair of women in beauty clinics. However, x-ray did have the most obvious use in medicine. As the negative side of x-ray use became apparent, it was soon realized that hospitals had to take control of the use of x-ray. Boston Hospital was the first to take control by making actual rooms for the use of x-ray and “training” people to take those x-rays. By 1905, Boston Hospital had five full-time technicians operating the equipment and taking medical x-rays.
Advances
As the gas tubes were improved and a vacuum was created, the modern tube was born. The newer tubes were stable, more flexible, and safer than the traditional tube used in the past. It was these advances that made possible the invention of the new imaging equipment used today. One such piece of equipment is the computerized axial tomography (CAT) machine. In 1972, Godfrey Hounsfield and Allan Cormack created an x-ray tube that encircled the patient who was lying on a table. Although the first scans took hours to complete, the advances have improved the equipment to today’s standards that ensure an entire body is scanned in less than 5 minutes.
In similar fashion, the magnetic resonance imaging (MRI) equipment was started in 1946 by Felix Bloch and Edward Purcell. In 1972, Dr. Paul Lauterbur first described the MRI technique in medicine and published the first MRI image in 1973. In 1977, Raymond Damadian showed how MRI could be used on the whole body. MRI works similarly to computer tomography (CT) but does not use radiation to image the body. Other advances in the field of x-ray are positron emission tomography (PET), single-photon emission CT (SPECT), mammography, and ultrasound. Not only has the field advanced with new modalities, but x-ray itself has gone from film to digital images. More than a century after it was discovered, the importance of x-ray continues to grow and the technology continues to amaze.
EARLY EFFECTS OF RADIATION
The x-ray was seen as a miracle to the world of medicine when it was discovered in 1895. In fact, it was the negative side of the miracle that was beginning to show itself. In the early 1900s, many problems, such as burns, infections, swelling, and cancer, finally forced the scientists to reinvestigate the whole process of x-ray and how it worked. Even amputations had to occur because of the lesions caused by overexposure to x-ray. Thomas Edison’s assistant and good friend Clarence Dally lost all of his hair, all of the fingers on both hands, and finally both of his hands before he finally succumbed to the effects caused by excessive radiation. He was in constant pain and finally died in 1904. He is the first known fatality of overexposure. Edison himself complained of skin rashes and sore eyes. It was this event that caused Edison to stop his work on radiation and start to look at the problems it caused. X-ray technicians fell victim to the terrible side effects. Twenty-eight Americans suffered the fatal effects from experimentation.
Early radiologist Mihran Kassabian photographed the horrible effects to his hands in hopes that future technicians would not suffer the same deformities. Kassabian was an early radiologist who would hold the fluoroscope with his right hand and put his left hand into the beam to show the patient that there was nothing to be afraid of. After months of constant exposure, the third and fourth fingers of the left hand had to be amputated.
The public was frightened when it became aware of these deaths and demanded that something be done. Physicians tried to calm the patients by telling them that they could not be harmed. The damage had been caused by overexposure to electricity, allergies, and even circumstances of the ozone created by static, excessive heat, and moisture. Scientists, on the other hand, knew that the x-ray was the cause and warned against its unwarranted use. If it must be used, lead shielding should be applied. Figure 1-2 shows the early protective suits and metal helmets that were introduced to protect against x-radiation.
Genetic and Somatic Effects of Radiation
Studies done on people throughout the years who were exposed to radiation have led to a greater understanding of what effects can happen through the use of radiation. Scientists concluded that the effects of radiation had to be broken down into two categories: acute (early) radiation syndromes (ARS) and long-term effects. ARS occurs with a large dose (larger than 100 R) to the whole body. These effects will cause varying degrees of damage to the body up to and including death.
Long-term effects will occur years after the radiation was administered, sometimes as long as 25 to 30 years afterward. There are two main categories of long-term effects: genetic and somatic effects.
Genetic effects are those that are manifested in persons born of people who were actually irradiated. They might not show for several generations because several criteria must be met before the mutations can be seen. Genetic effects were first studied on fruit flies in 1927 by Hermann Muller. After exposing both male and female flies to radiation in increasing doses, Muller studied the effects it had on the offspring. Others studied irradiated mice in 1946 to continue the investigation into the effects of the offspring.
Somatic effects are those damages that will occur to the person who received the radiation. The types of cells that receive the radiation will help determine the type of effect that manifests itself. Cells that are young, rapidly dividing, undifferentiated, and oxygen rich are more radiosensitive than others. The effects that are considered long term are carcinogenesis, cataractogenesis, and life-span shortening. Each of these conditions will occur based on the location of the radiation received and the amount of radiation that passed through the patient.
RADIATION PROTECTION
Protection from radiation exposure is two-fold: protection for the patient and protection for the occupational worker. The National Council on Radiation Protection and Measurement (NCRP) established dose limits for both the patient and the occupational personnel. These dose limits must not be exceeded. The current annual occupational dose limit is 5 rem (50 mSv). In 2009, NCRP no. 160 determined that the new annual dose limit should be 2 rem because continuing long-term studies have continued to indicate that radiation is three to four times more damaging than originally thought. Although the NCRP is currently reviewing the dose limits, the limit remains at 5 rem. The annual dose limit for the general public is one-tenth the amount of the occupational dose: 0.5 rem (5 mSv). Students in radiology training younger than 18 years have an annual dose limit of 0.1 rem (1 mSv).
Pregnant workers are allowed to receive 0.5 rem (5 mSv) in a 9-month gestation period and 0.05 rem (0.5 mSv) in any given month. Radiation workers are allowed to receive more per year than the general population because the workforce is a much smaller group as compared with the general public. In addition, radiation personnel are taught the safety measures necessary to protect themselves from large doses. Display 1-1 shows the effective dose limits for each of the different populations receiving radiation.
Pregnant workers are allowed to receive 0.5 rem (5 mSv) in a 9-month gestation period and 0.05 rem (0.5 mSv) in any given month. Radiation workers are allowed to receive more per year than the general population because the workforce is a much smaller group as compared with the general public. In addition, radiation personnel are taught the safety measures necessary to protect themselves from large doses. Display 1-1 shows the effective dose limits for each of the different populations receiving radiation.
DISPLAY 1-1 Effective Dose Limits for Different Populations Receiving Radiation
|
In order to observe these protection standards, the concept of As Low As Reasonably Achievable (ALARA) must always be practiced. The three parts of this concept include time, distance, and shielding.
Occupational Protection
First, it is important to know that the major source of radiation dose to the occupational worker is the patient! Scatter radiation coming from the patient travels in all directions. Looking at each one of the three concepts of ALARA will provide insight into the logic of personnel protection. “Time” refers to the time in the radiography room while the beam is energized—known as beam on-time. The longer the patient is irradiated, the more scatter that is produced and the more radiation the worker receives. The obvious solution is not to be in the same room as the patient; however, this is not always possible. When it is not, the technologist and other personnel should be in the room for no more than the required amount of time necessary to achieve a quality image.
If the technologist and others are not able to leave the room, then the second concept of ALARA should be practiced—distance. The farther away from the source (the patient) the technologist is, the less radiation that is received. A law that will be memorized and must be completely understood by the student is the inverse square law, which states that the intensity of the radiation is inversely proportional to the square of the distance of the object from the source. In other words, if the technologist stands 2 ft from the patient and receives an exposure of 1 mR per hour, the technologist will receive one-fourth of the exposure if he or she moves back to 4 ft from the patient. It is not hard to see that the farther away from the patient the technologist is, the lesser the radiation dose that the latter will receive.
Finally, shielding is a concept that must always be adhered to. Shields are aprons that can be worn over the arms and tied in the back, or short overcoat and skirt type that will protect the worker from all sides. The shields are made of lead and must be no less than 0.25 mm of lead (Pb) (Fig. 1-3). Shields of this lead weight will absorb 97% of all radiation below 50 kVp. An apron of 0.5 mm Pb will absorb 99% of the radiation. Any time the technologist or other personnel must be in the room, an apron must be worn as added protection in addition to staying away from the primary beam if the patient must be held in place for the image. Other protective apparel includes lead goggles, gloves, and thyroid shields, as pictured in Figure 1-4.
Patient Protection
The dose received by the patient will depend not only on the exposure factors (kVp and mAs) set by the technologist but also on the size of the field irradiated, sometimes known as the field of view, the number of images taken, and the location of the area of interest. Patients can be protected by two of the three ALARA concepts: time and shielding. Distance cannot play a part in protection for the patient because the patient must be in the primary beam at specific distances that will produce a diagnostic radiographic image. Therefore, the other two concepts plus two other important factors are the main ways to provide protection for the patient.
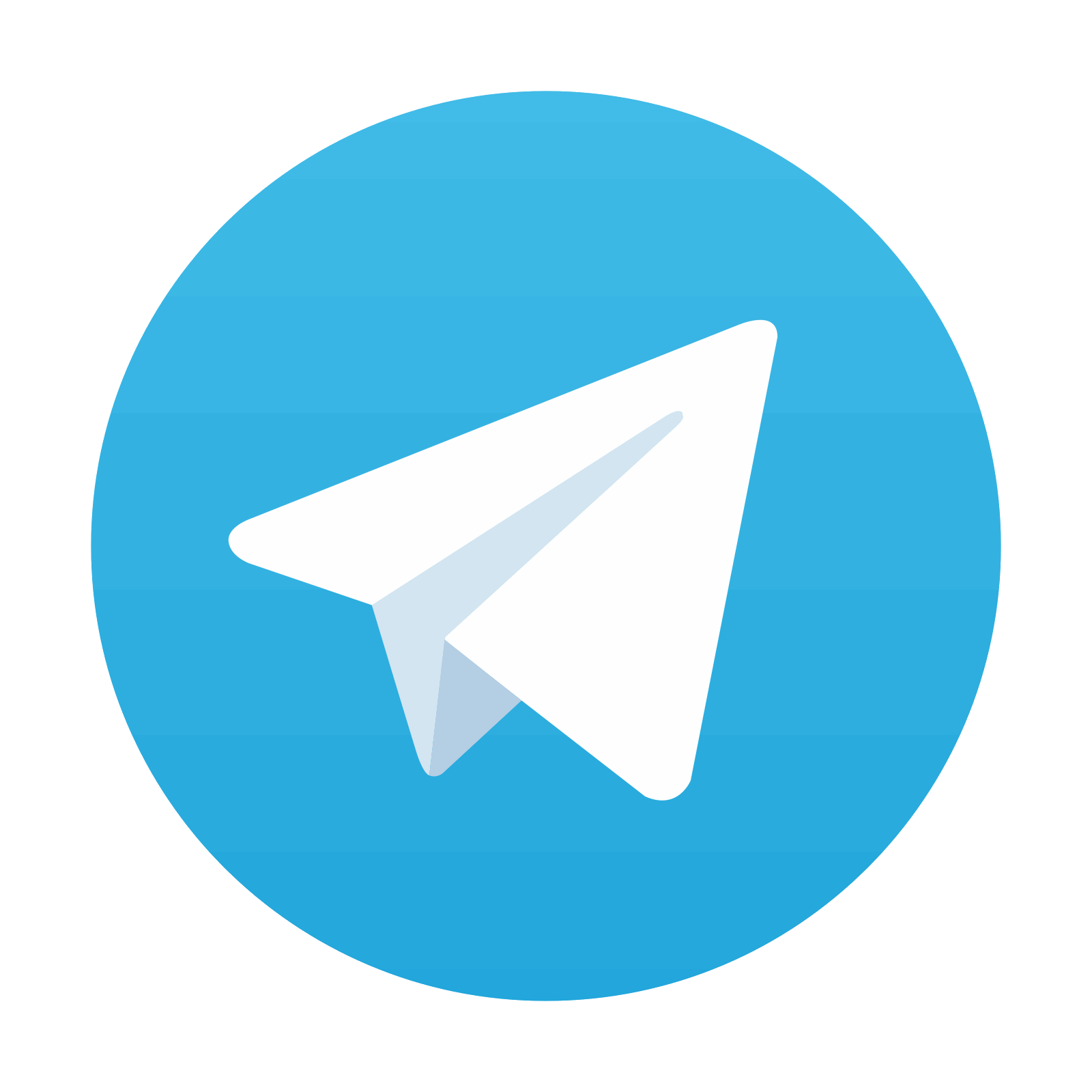
Stay updated, free articles. Join our Telegram channel

Full access? Get Clinical Tree
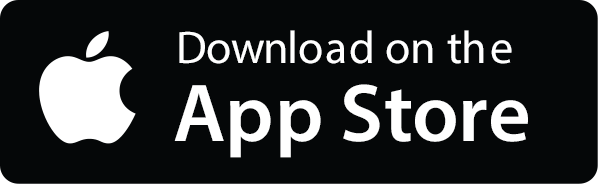
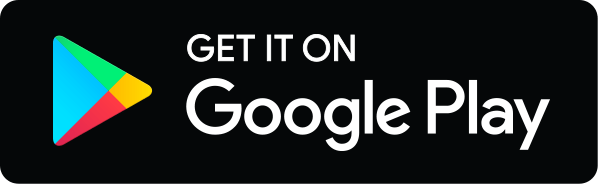