© Springer-Verlag London 2015
Andrew Gaya and Anand Mahadevan (eds.)Stereotactic Body Radiotherapy10.1007/978-0-85729-597-2_11. Introduction to Stereotactic Body Radiotherapy
(1)
Department of Clinical Oncology, Guy’s Hospital, Guy’s & St. Thomas’ NHS Foundation Trust, London, UK
(2)
Department of Radiation Oncology, Beth Israel Deaconess Medical Center, Harvard Medical School, Boston, MA, USA
Abstract
Indications for Stereotactic Radiotherapy have expanded in recent years from intracranial treatment to extracranial, leading to the development of a thriving subspecialty within radiation oncology. The evidence base is growing exponentially. However there is still a lack of good quality prospective randomized clinical trial data. This chapter provides an introduction to the subject and a broad review of the major indications, which are then covered in more depth within individual chapters.
Keywords
Stereotactic Body RadiotherapySBRTSABRAblationCyberknifeStereotactic body radiotherapy (SBRT) refers to the precise irradiation of an image-defined extracranial target using a small number (usually 1–5) of high-dose fractions. It has developed as an extension of intracranial stereotactic radiosurgery (SRS), and is conceptually different from conventionally fractionated external beam radiotherapy (CFR).
In CFR the tumour volume is irradiated together with a margin to account for tumour and organ motion, and the inaccuracies of planning, setup and delivery. The total dose is limited by the tolerance of normal tissue within, or close to, the planning target volume (PTV). Conventional fractionation (typically 1.8–2 Gy per fraction) optimises the therapeutic ratio.
In SBRT, the PTV contains the target lesion together with a much smaller margin of normal tissue. The intention is to deliver an ablative radiation dose to all tissue within the PTV, exploiting the potent radiobiological effect of large fraction sizes (see Chap. 5).
The safe delivery of ablative doses of radiation requires effective patient immobilisation, precise target localisation (which may involve fusion of different imaging modalities), sophisticated planning software, accurate treatment delivery, and the ability to produce a steep isodose gradient outside the target volume. In addition, extracranial lesions pose further challenges to treatment delivery due to inter- and intra-fraction tumour and critical organ motion. Until recently this has limited our ability to deliver stereotactic radiotherapy to targets outside the brain. However, advances in image guidance have allowed treatment systems to account for such motion, and consequently the use of SBRT is increasing.
1.1 History of SBRT
Stereotactic surgery was first described by Horsley and Clarke in 1906. They developed a method of locating deep-seated brain lesions by assigning coordinates in three planes to neuroanatomical structures, based on cranial landmarks [1]. In 1947 Spiegel and Wycis introduced frame-based stereotaxy using a plaster head cap known as a stereoencephalatome, and a 3-D coordinate system relative to this [2].
Lars Leksell, a Swedish Neurosurgeon, was the first person to marry the two developing fields of stereotaxy and radiation therapy, and introduced the term “Radiosurgery” in 1951. He used a rigid metal stereotactic head frame fixed to the skull. Small intracranial targets were localised relative to the frame, and radiation was delivered in a single high-dose fraction [3]. The technique initially employed 250 KV x-rays, but in 1967 the first Gamma Knife prototype was developed, using 179 Cobalt-60 sources focused on the target. Since then, Gamma Knife has become widely used for intracranial stereotactic radiosurgery, with sub-millimetre total system accuracy [4].
The 1980s saw the adaptation of linear accelerators for intracranial stereotactic delivery, again using rigid stereotactic head frames, and specialist dosimetry software e.g. X-Knife (Radionics, Boston, MA).
In 1995, Hamilton et al. proposed a method of delivering linac-based stereotactic radiotherapy to spinal lesions using a prototype rigid “extracranial stereotactic frame” and associated 3-D coordinate system. Immobilisation was achieved by transcutaneous frame fixation to spinous processes superior and inferior to the target. They reported an overall treatment accuracy of 2 mm, but the technique was time-consuming, cumbersome, and limited to the delivery of single fractions [5].
Around the same time, Lax et al. developed a stereotactic body frame which, together with a vacuum bag, immobilised the patient from head to mid-thigh. They found the setup reproducibility for liver and lung lesions to be within 5–8 mm for 90 % of the patients. Diaphragmatic movements were reduced to 5–10 mm by applying pressure on the abdomen [6]. Many stereotactic radiotherapy systems today use a similar setup of body frame immobilisation, and some centres use corsets to limit diaphragmatic movement.
However for most extracranial sites the position of the tumour does not enjoy a fixed relationship relative to the external body contour, and can move both between and during each fraction of radiotherapy. An external body frame alone is therefore not sufficient to ensure accurate delivery of radiation to the target. Safe delivery of large fractions of radiotherapy requires sophisticated image guidance.
Image guidance in radiotherapy became a realistic concept with the development of the electronic portal imaging device (EPID) and software to aid quantitative evaluation of patient setup, thus allowing correction of translational errors. The next step was moving from “off-line” to “on-line” image guidance (ie adjusting patient position on the basis of imaging, before each fraction). Accurate identification of tumour position has improved with the use of inserted metal fiducial markers with planar images, or alternatively with the development of volumetric image guidance (eg cone beam CT, or in-room CT on rails). More recently, improved software, together with more sophisticated treatment couches, have meant that correcting for rotational setup errors is now possible. Finally, intra-fractional image guidance is now available, and is a key component of some of the stereotactic treatment systems described below.
Stereotactic systems which use planar imaging have great flexibility with respect to taking multiple intra-fraction images, but largely rely on implanted fiducials. Percutaneous fiducial insertion can be technically difficult, especially in the upper abdomen where it may be necessary to pass through other organs to reach the target lesion. See also Chap. 2.
1.2 Fractionation and Radiobiology
The therapeutic benefit achieved with dose fractionation has been recognised for over 100 years [7]. Conventional fractionation has emerged from such early observations, with subsequent refinement as our knowledge of radiobiology has developed. The linear quadratic model [8] and its ability to describe cellular response to radiation, together with Withers’ “4 Rs” of radiotherapy—DNA Repair, Reoxygenation of the tumor, Redistribution within the cell cycle, and Repopulation of cells [9], have had a big influence on modern CFR regimes.
In contrast, intracranial radiosurgery exploits the ablative power of large single doses of radiation, which transcends the considerations proposed by Withers. Considerable dose inhomogeneity within the target volume is standard practice, due to the internal dose gradient achieved by using a low prescription isodose (commonly 40–60 % with gamma knife radiosurgery). There is some evidence to suggest that, rather than being a problem, target dose inhomogeneity may enhance the tumoricidal effect [10].
SBRT sits somewhere between the extremes of CFR and radiosurgery. Large doses per fraction are used, and a moderate internal dose gradient achieved, with a typical prescription isodose of around 60–80 %. However, unlike intracranial radiosurgery, inter- and intra-fraction target movement is a significant problem, increasing the risk of irradiating normal tissue, or missing the tumor, during treatment. Also we are treating at sites where the overwhelming clinical experience and evidence is with conventional fractionation. Conceptually, moving to a single large fraction is big step. The linear quadratic model and its derivatives can help clinicians to predict tissue response to altered fractionation regimes. However, there has been concern that it does not accurately predict tumor cell response at the higher doses per fraction (>10 Gy) seen with stereotactic treatment [11]. At these doses it is not clear to what extent modest fractionation (2–5 fractions) differs from a single fraction with respect to tumour response and normal tissue effects.
Unsurprisingly, therefore, there has been a large variation in dose and fractionation across SBRT series published to date. Whilst some SBRT centres adopt a “single large fraction” strategy for many patients, other centres would prefer to fractionate in similar cases. Current regimes have in many cases been derived empirically, often the result of cautious dose escalation.
See also Chap. 5.
1.3 Overview of SBRT Systems
A number of modern linacs with on-board imaging capabilities meet the basic image guidance requirements for delivering SBRT (e.g. Varian TruBeam, Elekta Synergy). A micro-multi leaf collimator can be added to produce the required degree of conformality for stereotactic plans.
More recently we have seen the introduction of linacs fully adapted as integrated stereotactic delivery systems. Novalis TX has a Varian Trilogy linac base with micro (2.5 mm) MLC, together with Brainlab ExacTrac image guidance and 6D Robotic Treatment Couch, and associated software. Elekta Axesse is a similar integrated system.
The TomoTherapy System (Accuray Inc, Sunnyvale, CA) has a ring gantry as used in diagnostic CT scanners, and delivers helical IMRT via thousands of small beamlets. Couch movement is continuous during radiation delivery. The system has on-board image guidance with megavoltage CT.
The Cyberknife (Accuray, Sunnyvale, CA) is an image-guided robotic radiosurgery system. A compact 6 MV x-band linac is mounted on a six joint robotic arm. This provides huge flexibility in beam pattern generation, allowing the system to produce very conformal, non-isocentric plans with a steep dose gradient around the target. Near real-time imaging is achieved using two diagnostic x-ray sources positioned orthogonally in the treatment room. Targets that move with breathing can be tracked using the Synchrony respiratory tracking system. Thus the system can correct for both inter- and intra-fraction target movement, which obviates the need for a stereotactic frame. Total system accuracy has been found to be less than 1 mm [12, 13].
See also Chap. 3.
1.4 Respiratory Motion
With thoracic or upper abdominal SBRT we face the additional challenge of accounting for intra-fraction target movement with breathing. The majority of the published data come from centres using gantry-based linacs with vacuum and/or frame body immobilisation, and diaphragmatic pressure to reduce breathing movement. Respiratory gating methods such as Active Breathing Control (used in some series) have sought to reduce the volume of tissue irradiated by requiring a smaller GTV-PTV margin. The latest gantry-based stereotactic systems (eg Novalis TX) allow respiratory gating using infra-red chest wall tracking and intra-fraction x-ray imaging. 4-D CT planning allows the construction of an internal target volume which takes into account the tumour position at all phases of the respiratory cycle. It has been shown to increase the accuracy of, and reduce the volume of normal tissue irradiated in conformal radiotherapy, and is now being used in some lung SBRT centres.
For Cyberknife, the Synchrony Respiratory Tracking System monitors chest wall movement continuously via an infra-red camera and LEDs placed on the patient’s chest. Regular static KV images of the tumour are taken during setup and treatment, and correlated with chest wall movement. A regularly updated predictive model is generated which anticipates future tumour movement with breathing, and the robotic arm moves the radiation beam accordingly. The total accuracy for moving targets has been reported as 1.5 mm. “Xsight Lung” is a further software development for Cyberknife, which allows the tracking of certain peripheral lung lesions (within strict parameters) without the need for implanted fiducials.
1.5 Summary of Major SBRT Indications
1.5.1 Primary Non-Small Cell Lung Cancer (NSCLC)
There are several important published series of lung SBRT. The largest is a Japanese retrospective review of 257 patients from 14 institutions [14]. Patients had resectable stage I disease, but were either medically inoperable or declined surgery. There was considerable variation in immobilisation and respiratory motion management protocols, and also heterogeneity of dose and fractionation (30–84 Gy in 1–14 fractions). Five year actuarial local control rates were 84 % for patients receiving a BED of 100 Gy or more (based on assumed tumour α/β of 10) and 37 % for those receiving less than 100 Gy. Five year overall survival for medically operable patients receiving the higher dose range was 71 %. This was achieved with relatively low rates of radiation toxicity.
There have also been a number of published series of linac-based lung SBRT from European centres [15–19], reporting 88 % local control at around 3 years.
A phase 2 study enrolled 70 inoperable Stage I patients with peripheral or central (within 2 cm of the proximal bronchial tree) tumours, giving 60–66 Gy in 3 fractions. Two year LC and OS were estimated at 95 and 54 % respectively. However, eight patients developed grade 3–4 pulmonary or skin toxicity, and there were six possible cases of grade 5 pulmonary toxicity. The risk of severe pulmonary toxicity was 11 times higher for central, compared to peripheral, tumours [20]. On the basis of these results, centrally located tumours, as defined above, were excluded from two subsequent phase II trials.
For central tumours, the maximum tolerated dose is still under investigation. Chang et al. treated a series of 27 centrally or superiorly located lesions with a slightly more modest dose of 40–50 Gy in 4 fractions. At a median of 17 months, there was no local recurrence seen in the 20 patients receiving 50 Gy (BED 112.5 Gy). There were three cases of grade 2–3 skin/chest wall toxicity and one brachial plexopathy, related to a large volume of plexus receiving 40 Gy. However there was no observed grade ≥3 pulmonary or oesophageal toxicity [21]. RTOG 0813, a phase I/II dose escalation trial, is underway for central stage I tumours in medically inoperable patients.
There have been a number of published series of primary lung SBRT using Cyberknife [22, 23]. Brown et al. treated 59 stage I patients with peripheral tumours. Doses ranged from 15 to 67.5 Gy in 1–5 fractions. With follow up ranging from 1 to 33 months, only 10 % patients had persistent or recurrent disease, and OS was 86 % [23].
SBRT appears to be a safe and effective treatment for early stage NSCLC. With respect to LC, achieving a BED of >100 appears to be very important. Unfortunately there are still no published randomised data comparing SBRT and surgery for operable patients with early stage disease. However, these trials are now underway. ROSEL is a Dutch multi-centre phase III randomised study of linac-based SBRT vs surgery for peripheral Stage 1A NSCLC. The Lung Cancer STARS Trial is an international phase III trial of SBRT with Cyberknife vs Surgery for Stage IA or IB patients (maximum diameter <4 cm). Peripheral tumors receive 60 Gy in 3 fractions, and central tumors 60 Gy in 4 fractions. It will be some time before these trials produce mature data, and in the meantime surgery remains the standard of care. For the large number of medically inoperable patients, SBRT has emerged as the best treatment option.
See also Chap. 8.
1.5.2 Lung Oligometastases
The term “oligometastases” refers to a finite small number of metastases (usually ≤6) confined to a single or limited number of organs. Long term follow up of patients following surgical resection of lung and liver metastases has shown that some of these patients are effectively cured following surgery [24, 25]. For example, in an analysis of over 5,000 patients with lung metastases, the survival following complete surgical resection was 36 % at 5 years and 22 % at 15 years [24]. Thus in some cancers, there appears to be a stable tumor state somewhere between purely localised and widely metastatic. This, together with the results of administering ablative doses of radiation to primary lung tumors, has led to increasing interest in the use of SBRT for oligometastases.
The early data on SBRT for pulmonary metastases emerged as heterogenous published series including both primary and metastatic lung lesions. Wulf et al. [15] demonstrated that with 9 months median follow up, local recurrence/progression was seen in 5 of 51 metastatic lesions. Once again there was evidence of a dose-response relationship, as four of these five lesions had received the lowest dose (3 × 10 Gy). In a subsequent publication from the same centre, at 18 months median follow up they reported recurrence in 6 of 48 metastatic lesions, giving a crude LC rate of 88 %. Symptomatic pneumonitis was seen in 10 % of patients, with one case of grade 3 pneumonitis [26]. Similar results have been seen in other such series [27, 28].
More recently, several series of purely metastatic lung cases have been reported [29–31] with similar results.
As with primary NSCLC, there are as yet no published randomised data comparing surgery and SBRT for oligometastatic lung disease, but the results seen are encouraging, and the non-invasive approach with low risk of toxicity makes it an attractive option for these patients.
1.5.3 Liver Metastases
As with lung metastases, surgical series of metastectomy for liver metastases have shown a proportion of long term survivors. In a series of 1,000 patients from Memorial Sloan-Kettering Cancer Center with resectable liver-only metastases from colorectal cancer, survival was 37 % at 5 years and 22 % at 10 years [25]. Surgery remains the gold standard for resectable disease, but many patients are unresectable, either due to the extent of metastatic disease, insufficient functional liver reserve or general medical condition. There has therefore been interest in potentially curative, non-surgical options for unresectable patients with oligometastatic disease. Radiofrequency ablation (RFA) is widely practiced with local control rates comparable to surgery for lesions less than 3 cm [32], but lesions close to large vessels or the diaphragm are contraindicated for this technique. Transarterial embolisation, chemoembolisation, irreversible electroporation and radioembolisation are further options.
Whilst whole liver radiation has been used in the past for palliation [33], the radiosensitivity of normal liver tissue has limited the ability to deliver a radical dose to oligometastases in the liver using conventional radiotherapy techniques. Radiation induced liver disease (RILD) is now well documented as a potentially life-threatening condition. SBRT offers the opportunity to deliver ablative doses, whilst staying within acceptable liver DVH constraints.
Patients with liver metastases formed part of Blomgren et al’s early series of SBRT using the Elekta stereotactic body frame [34]. Since then there have been a number of published series and early phase trials in this area [35]. Stanford University has published single fraction Cyberknife experience in liver mets [36].
Other centres have adopted a 3-fraction approach to treating liver metastases. In a phase I study by Schefter et al. for 18 patients with 1–3 metastases, the dose was escalated from 36 to 60 Gy without causing any dose-limiting toxicity. In fact, no toxicity >grade 1 was recorded. Patients were treated with linac-based SBRT, using a body frame and either abdominal compression or Active Breathing Control to account for respiratory motion. Final results of a subsequent multi-institutional phase II study from the same group have recently been published [30]. All patients received 60 Gy in 3 fractions. Thirty six of the 47 patients were assessable for LC, which was estimated to be 92 % at 2 years (100 % for lesions <3 cm diameter). OS was 30 % at 2 years, although 45 % patients had active extrahepatic disease at the time of treatment. Other series also show promising results [37, 38].
See also Chap. 9.
1.5.4 Primary Liver Tumours
Wherever possible, surgery is the treatment of choice for primary hepatocellular carcinoma (HCC), but a significant number of patients are not suitable for either resection or liver transplantation. Studies have shown RFA and Transarterial Chemoembolisation (TACE) to be effective treatments, but again not all patients are suitable. In primary liver disease, SBRT has been used predominantly in patients in whom other local treatments are not suitable, or who have recurred following previous local treatment. There have also been exclusive published SBRT series of primary liver tumors [39, 40].
Princess Margaret Hospital published a parallel phase I study of 41 patients with unresectable HCC or intrahepatic cholangiocarcinoma (IHC). Patients were required to have Child-Pugh A liver function, and >800 cc of uninvolved liver, but tumor sizes were large. One year LC was 65 %, with 51 % 1 year OS. There was no RILD or DLT, but a high incidence of grade 3 liver dysfunction [39]. Choi et al. reported results of SBRT for 20 patients with HCC. 80 % patients had at least partial response and 2 year OS was 43 %, with no toxicity ≥grade 3 [40].
Mirabel has reported the Lille experience of treating HCC and liver metastases with Cyberknife. Twenty one patients with HCC received 45 Gy in 3 fractions. One year progression free survival was 94 % and median survival estimated at 18 months. There was one case of grade 3 duodenal stenosis [41]. There have also been published series of both Cyberknife and linac-based SBRT used in combination with TACE for HCC, showing that these two modalities can be safely used together where appropriate [42, 43].
See also Chap. 9.
1.5.5 Pancreas
Surgery is the standard of care for pancreatic cancer, but unfortunately only 10–20 % of patients are diagnosed with resectable disease. Patients with metastatic disease at diagnosis proceed directly to systemic therapy. For locally advanced non-metastatic or medically inoperable patients, the optimum treatment is less clear. Trials have shown chemoradiotherapy to improve survival compared to radiotherapy alone [44, 45], but there is conflicting evidence as to whether chemoradiotherapy is superior to chemotherapy alone, both in comparisons with 5 FU-based chemotherapy [46, 47], and with newer gemcitabine-based regimes [48, 49].
Local failure is still a problem in patients treated with CFR. The radiotherapy dose prescribed is limited by small bowel and, especially, duodenal toxicity, although the latter becomes less important following palliative gastrojejunal bypass surgery, which is performed in some centres. The development of conformal radiotherapy has led to interest in the possibility of safe dose escalation to achieve better LC. A Dutch phase II study treated patients with a dose of 70–72 Gy in 2 Gy fractions, but LC with median 9 months follow up was only 56 % [50]. There were also unacceptable normal tissue effects, with 9 % grade 3 acute toxicity and 18 % grade 3–5 late toxicity, including three deaths due to GI bleeding.
There has been hope that SBRT may succeed where CFR has failed in safe and effective dose escalation. Hoyer et al. treated 22 patients with locally advanced pancreatic cancer, with a dose of 45 Gy in 3 fractions. LC at 6 months was again disappointing at 57 %, and median survival only 5.4 months, with 95 % developing metastatic disease at the time of recurrence. Furthermore, 64 % patients experienced ≥grade 2 toxicity, including one case of non-fatal gastric ulcer perforation [51].
Other published results have, however, been more promising. Koong et al. used single fraction Cyberknife radiosurgery for patients with locally advanced disease. Two of the 15 patients had received prior chemoradiotherapy to 50 Gy. The dose started at 15 Gy and was increased to 25 Gy in 5 Gy increments. No toxicity ≥grade 3 was reported, although median follow up was only 5 months. In the group receiving 25 Gy, LC was achieved until death or end of follow up. However, median survival was only 11 months for the whole study group [52].
More recently, an updated series of 77 patients treated with a 25 Gy single fraction has been published by the same institution, with 6 months median follow up [52]. Whilst this is the largest series of SBRT for pancreatic cancer to date, it is heterogenous. Nineteen percent patients had metastatic disease and 10 % had recurrent local disease. Also 27 % were resectable, with surgery not possible due to either medical reasons or metastatic disease. Nevertheless, the results confirm that this regime provides good LC, with estimated 84 % freedom from local progression at 12 months. Unfortunately median survival was still only 6.7 months from time of radiosurgery treatment, although only 9 % of patients had received chemotherapy. Ten percent patients developed ≥grade 3 acute or late GI toxicity.
Whilst SBRT can significantly reduce local recurrence, and may improve quality of life as a result, there is no evidence yet that it improves overall survival. It should be stressed that systemic therapy is central to the management of locally advanced patients, as most will still die of metastatic disease. With this in mind, a short course of stereotactic radiotherapy is much less likely to interfere with a patient’s systemic therapy regime, than 5–7 weeks of CFR. Patients with at least stable disease after initial chemotherapy and a PET scan negative for distant disease at that time are the ones most likely to benefit from stereotactic radiotherapy to the primary tumour.
See also Chap. 10.
1.5.6 Kidney
Renal cell carcinoma (RCC) has traditionally been viewed as a radioresistant tumour, as results of CFR on primary and metastatic lesions have been disappointing. However, published series of radiosurgery for metastatic RCC in the brain have shown that the tumour is sensitive to hypofractionated treatment [53, 54]. This has led to interest in the use of SBRT for primary RCC [55], and for extracranial oligometastatic RCC [56].
Wersall et al. published the Karolinska Institute 5 year experience of linac-based SBRT in RCC (primary and metastatic tumors). They treated 162 lesions in 58 patients, including eight inoperable primary tumors and 117 lung metastases. Dose was heterogenous, varying between 25 and 45 Gy in 2–5 fractions according to the size and location of the lesions. Overall LC was impressive at 90 % with median 37 months follow up. However, 40 % patients experienced side effects, and half of the registered side effects were grade 3 in severity [57]. The authors published prospective phase II results the following year, which confirmed the impressive LC seen with their technique (98 % in the 82 treated RCC lesions) [58]. Seventy-three percent of patients with metastatic disease at the time of treatment developed new metastases during follow up.
Given the unpredictable natural history of renal cell metastases, we need randomised data to determine whether an aggressive management strategy, involving SBRT, significantly improves survival in oligometastatic RCC.
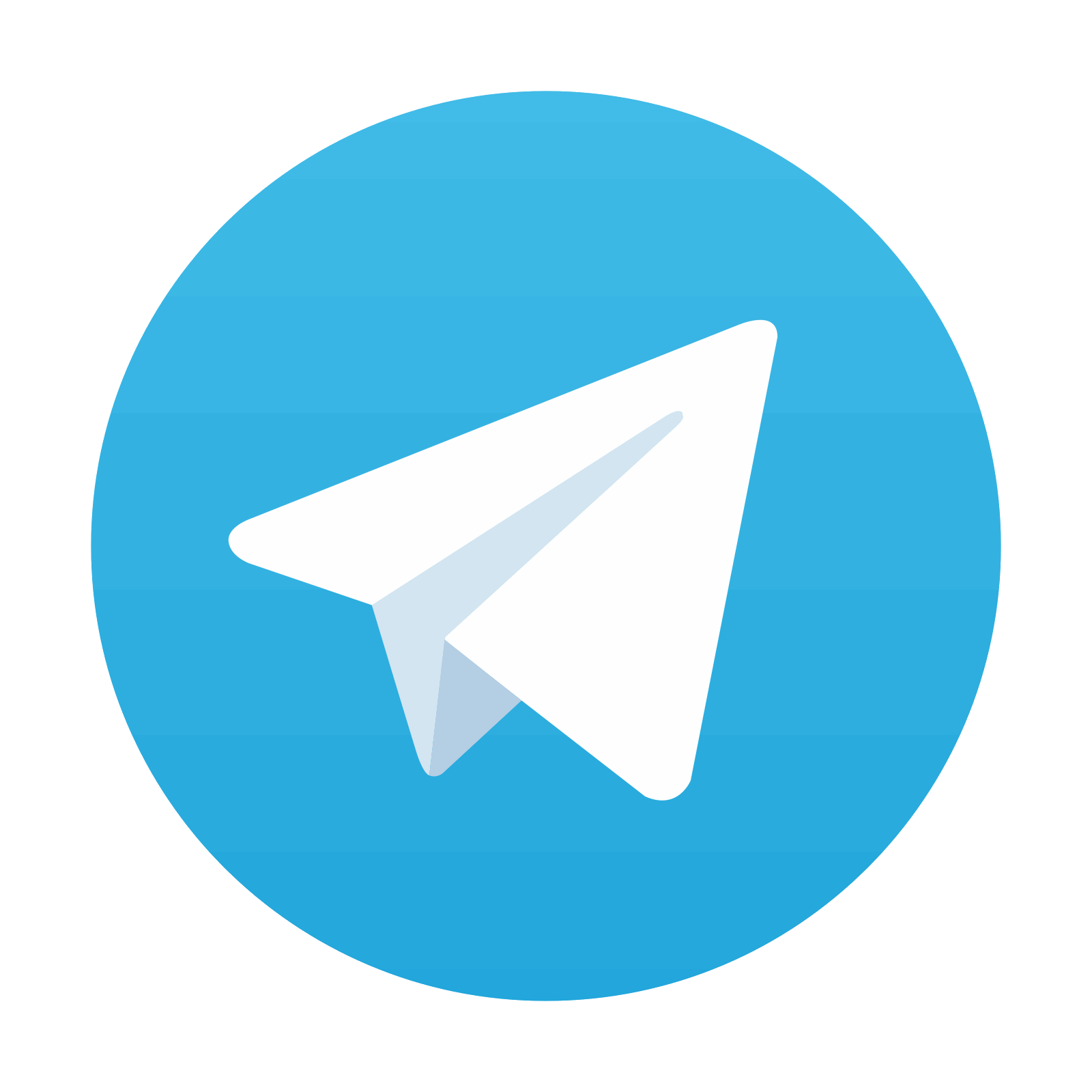
Stay updated, free articles. Join our Telegram channel

Full access? Get Clinical Tree
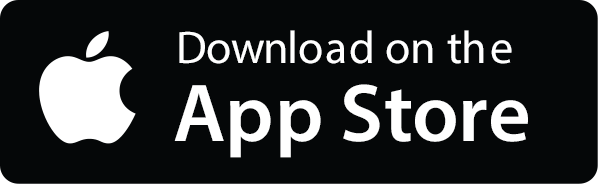
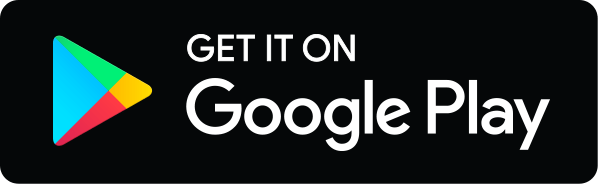