© Springer International Publishing Switzerland 2016
Rajni A. Sethi, Igor J. Barani, David A. Larson and Mack Roach, III (eds.)Handbook of Evidence-Based Stereotactic Radiosurgery and Stereotactic Body Radiotherapy10.1007/978-3-319-21897-7_11. Introduction to Stereotactic Radiosurgery and Stereotactic Body Radiotherapy
(1)
Departments of Radiation Oncology and Neurological Surgery, University of California, San Francisco, 1600 Divisadero Street, Basement Level, San Francisco, CA 94143-1708, USA
Stereotactic radiosurgery (SRS ) and stereotactic body radiotherapy (SBRT ) have an established but evolving role in the management of malignant and benign conditions. They have altered the way clinicians think about fractionation, and therefore they rank among the most important advances in radiation oncology, along with the development of megavoltage treatment machines, imaging -based treatment planning, and intensity-modulated radiation therapy. SRS and SBRT technologies were developed largely by dedicated medical physicists, (Benedict et al. 2008) with input from clinicians. Approximately 5–10 % of US radiotherapy courses are delivered with SRS or SBRT, and these technologies and their clinical outcomes are now a firmly established part of the educational curriculum for resident physicians in radiation oncology and neurosurgery.
Historical Foundations
During the 1950s, neuroanatomists and neurophysiologists developed techniques to produce small, highly localized, ablative CNS radio-lesions in animals using a variety of radiation sources, including implanted radon seeds, implanted isotopes such as Au198 and Co60, betatron X-rays, and cyclotron-produced protons and deuterons. Swedish neurosurgeon Lars Leksell, a pioneer in the development of stereotaxy, recognized that small, accurately placed radio-lesions could be produced in humans. In 1951 he coined the term “radiosurgery ” and is recognized as the father of radiosurgery (Leksell 1951). He performed focal single-fraction experiments in the brains of goats, cats, and rabbits using multiple cross-fired proton beams as he sought an optimum dose to produce discrete CNS lesions of dimension 3–7 mm. He found that a suitable maximum dose for the production of a discrete lesion within 1–2 weeks was 20 Gy in a single fraction. In the 1950s and 1960s he pioneered X-ray and proton SRS for pain syndromes and movement disorders . In 1961 he used 3 mm cross-fired proton beams to perform thalamotomies for pain control. He invented the Gamma Knife and performed his first Gamma Knife procedure in 1967. In 1974 that first Gamma Knife was installed as an experimental tool at UCLA under the direction of neurosurgeon Bob Rand.
During the 1950s, in the USA, internist John Lawrence, often called the father of nuclear medicine, developed highly focal ablative radiation procedures with cyclotron -produced protons, deuterons, and helium ions at what is now called Lawrence Berkeley National Laboratory (where John’s brother, Ernest, invented the cyclotron, for which he was awarded the Nobel Prize). He took great interest in pituitary disorders and performed multi-fraction dose/targeting studies in dogs, rats, and monkeys using bone landmarks to target and ablate the pituitary with multiple cross-fired beams. He initiated human studies in 1954, initially to suppress pituitary function in breast cancer patients and subsequently to treat acromegaly . He tried numerous fractionation schemes, eventually settling on 300 Gy in six fractions over 2 weeks to ablate the pituitary without damage to the surrounding tissue.
In 1961, Massachusetts General Hospital neurosurgeons William Sweet and Raymond Kjellberg initiated treatment of pituitary tumors and arteriovenous malformations with single-fraction Bragg-peak protons. Kjellberg searched the literature for examples of brain radio-necrosis in humans, monkeys, and rats, and plotted his findings as log of dose sufficient to produce necrosis versus log of beam diameter, and connected the data points with a steep straight line demonstrating the strong relationship between treatment volume and likelihood of necrosis. His plot indicated that 10 Gy was sufficient to produce necrosis for a 10 cm beam diameter and 4000 Gy was sufficient to produce necrosis for a 10 μm beam diameter (Kjelberg 1979). Many of his initial SRS treatments involved doses considered just sufficient to cause radionecrosis , according to his necrosis plot.
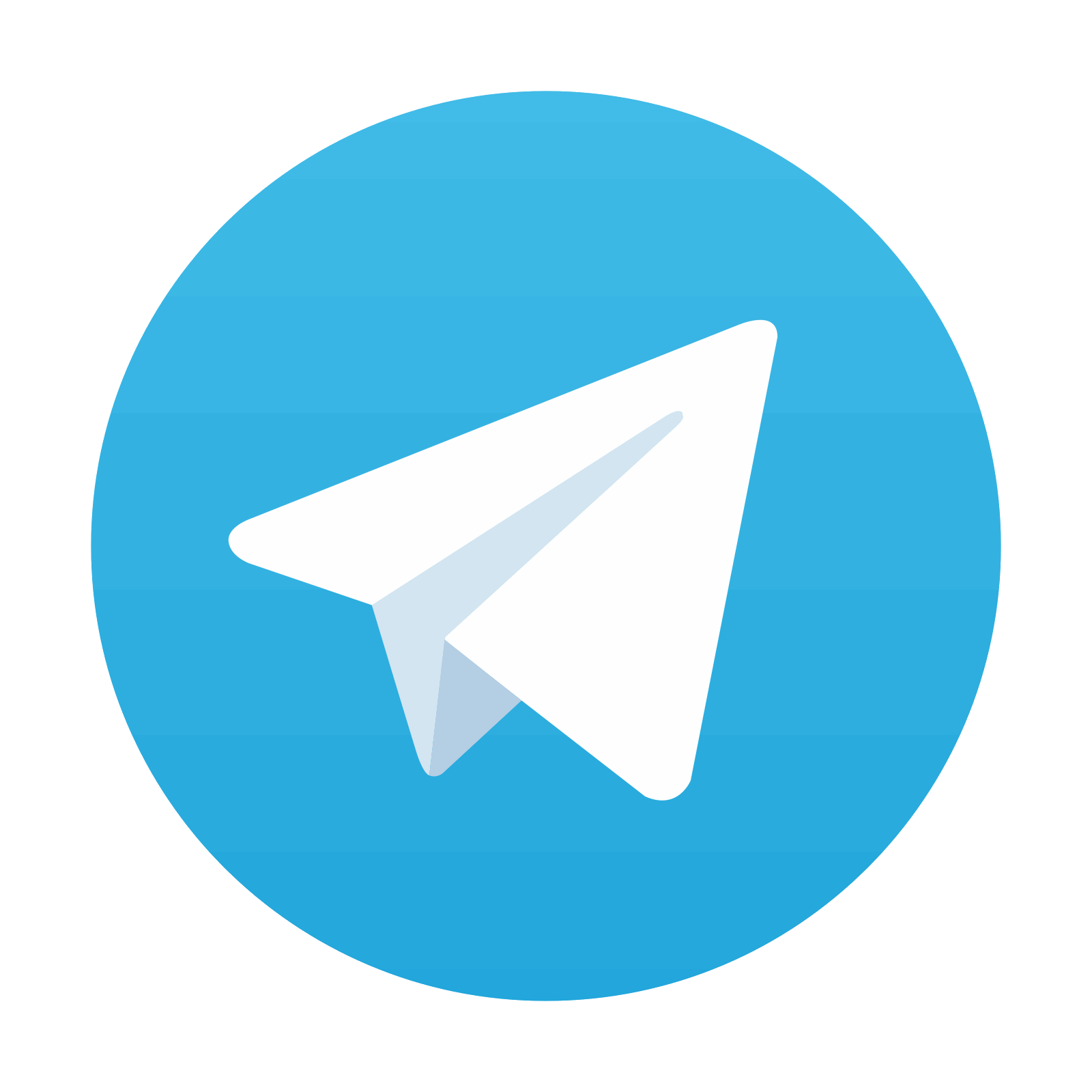
Stay updated, free articles. Join our Telegram channel

Full access? Get Clinical Tree
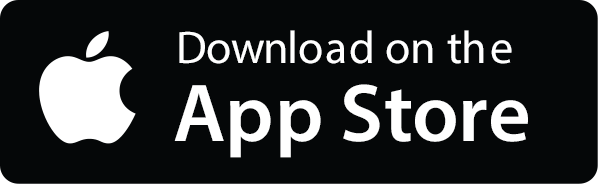
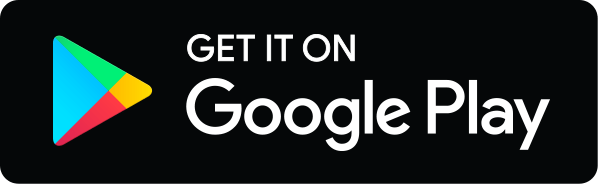