- •
Large-vessel vasculitides are a broad spectrum of diseases that can affect the aorta and its main branches.
- •
Multimodality imaging plays an essential role in the diagnosis and management of large-vessel vasculitides.
- •
Morphologic imaging with cross-sectional techniques such as US, CCTA, and MRI/MRA allow for the assessment of the detailed anatomic changes of the vessels, such as vessel wall thickening, mural enhancement, and luminal changes including stenosis or aneurysmal dilation.
- •
FDG PET/CT has emerged as a unique modality for the assessment of disease activity in patients with large-vessel vasculitides and to monitor response to antiinflammatory therapy.
Introduction
Vasculitis (vasculitides) comprises a large group of heterogeneous diseases characterized by the inflammation of the blood vessel wall. Broadly, this entity can be dichotomized into inflammatory/noninfectious and infectious vasculitis. Inflammatory/noninfectious vasculitides are largely thought to have an autoimmune pathobiology. Nevertheless, understanding of the exact pathophysiology remains unclear. Consequently, the classification of vasculitides is based largely on the size of affected vessels. The large vessels include the aorta and its main branches, the medium vessels include the main visceral arteries and initial branches, and the small vessels include intraparenchymal vessels or capillaries and analog veins. Inflammatory vasculitides have been categorized as large-, medium-, small-, or variable-vessel vasculitis and vasculitis limited to a single-organ (single-organ vasculitis [SOV]), such as central nervous system vasculitis or isolated aortitis. Collectively, these categories are known as primary (or idiopathic ) vasculitides . Although categorization based on vessel size is practical, there is a significant amount of overlap among the vasculitis categories. For example, large-vessel vasculitis (LVV) predominantly involves large vessels but can also affect medium- or small-size vessels. Similarly, medium-vessel vasculitis can extend into the small vessels. Variable-vessel vasculitis does not have any vessel predominance and can involve any vessel throughout the body, including the large vessels. Vasculitides associated with systemic disease or with a probable etiology are considered a type of secondary vasculitis. These secondary vasculitides can also involve large vessels, such as in rheumatoid or sarcoid aortitis. Fig. 30.1 summarizes the widely adopted nomenclature and categorization of vasculitides. In contrast, infectious vasculitis of the large vessels is caused by a pathogen from either direct invasion of the adjacent structure or that is spread via the bloodstream into the vessel wall.
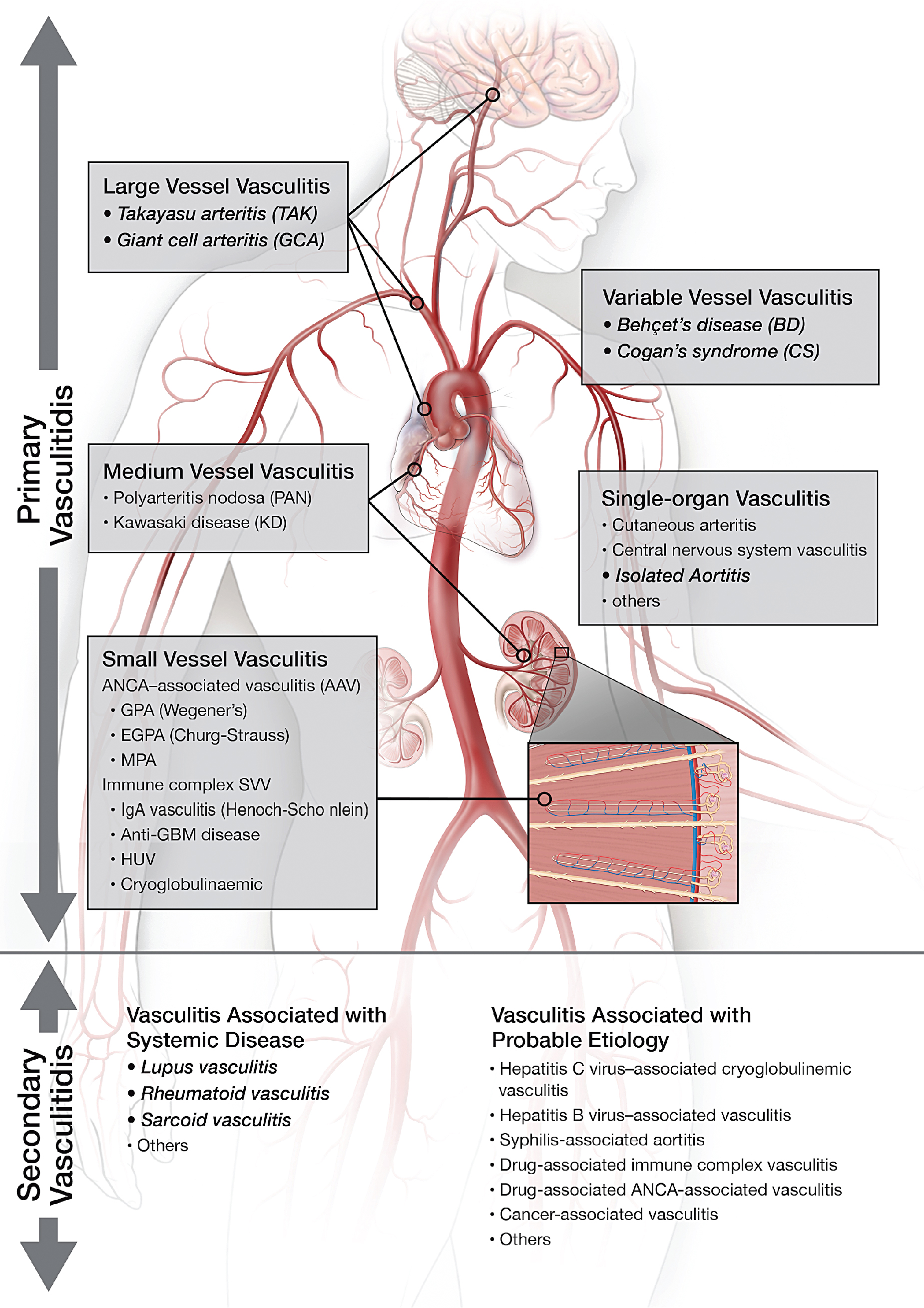
Although the LVVs characteristically consist of Takayasu’s arteritis (TAK) and giant cell arteritis (GCA), in this chapter, we have expanded to cover all vasculitides that affect the large vessels (i.e., aorta and main branches). We will discuss all available imaging modalities in the diagnosis and management of LVVs and illustrate the aforementioned vasculitides via image-rich case vignettes.
Classification and diagnosis of large-vessel vasculitides
Large-vessel vasculitis
LVV has two major and most common variants: GCA and TAK. These are immune-mediated granulomatous inflammations that may involve any vessel in the body but predominantly involve large vessels. Inflammation starts from the adventitia of the vessel wall and extends toward the media and intima, resulting in circumferential vessel wall thickening, luminal narrowing/stenosis, or aneurysmal dilatation. The histopathology of these two entities is indistinguishable, likely because of similar pathways of tissue inflammation. Both TAK and GCA have female predominance. TAK primarily affects young females (<50 years old) and is more common in Asia. GCA, on the other hand, is often seen in older patients (>50 years old) in Western countries. TAK involves the aorta and main branches and can lead to ischemia or end organ damage. The pulmonary arteries are rarely involved in patients with TAK. GCA is classically known to affect the head and neck arteries, particularly the temporal artery and branches. This form is also known as cranial GCA (c-GCA), temporal arteritis , or Horton’s disease . Autopsy and imaging studies, however, have shown that up to 80% of patients with c-GCA may have involvement of any of the extracranial large vessels, which is known as extracranial GCA or large-vessel variant GCA (LV-GCA).
Classical clinical presentation includes nonspecific constitutional symptoms in both TAK and GCA; carotidynia in TAK; and headache, jaw claudication, and scalp tenderness in GCA. During the later phase of TAK (the so-called pulseless phase), symptoms are associated with arterial stenosis or occlusion. A disorder known as polymyalgia rheumatica (PMR) belongs to the same disease spectrum as GCA, and both can coexist in the same patient. PMR is characterized by aching and morning stiffness of the neck, shoulders, and pelvic girdle.
Diagnostic criteria proposed by the American College of Rheumatology (ACR) for both TAK and GCA include clinical features, inflammatory markers (erythrocyte sedimentation rate [ESR], C-reactive protein [CRP]), and histopathology/biopsy. , Although these diagnostic criteria have been widely adopted for both GCA and TAK, they are not up to date with the current disease definitions and, thus, perform poorly. One of the major limitations of the ACR criteria is that LV-GCA without any c-GCA (isolated LV-GCA) would not be classified as GCA. Additional diagnostic criteria (e.g., National Institutes of Health [NIH] criteria) and scoring systems (e.g., the Indian Takayasu Activity Score) are recommended for TAK. , These are also based on only clinical symptoms and inflammatory markers and do not include imaging features.
Variable-vessel vasculitis
Behcet’s disease and Cogan’s syndrome involve any vessel in the body, including large vessels. Clinical features of these entities are extremely helpful in the diagnosis and classification of disease. Behcet’s disease predominantly affects young male patients with recurrent oral or genital ulcerations and ocular inflammation. Although venous involvement and thrombophlebitis is more common in Behcet’s disease, arterial lesions commonly occur in the aortic root and ascending aorta. The most common arterial pathology is aneurysmal dilatation, wall inflammation, and associated valvular abnormalities. Cogan’s syndrome also affects young patients and is associated with hearing impairment, dizziness, and visual disturbances, mostly because of small-vessel vasculitis and involvement of the large vessels, including aortitis.
Single-organ vasculitis
SOV refers to inflammation of arteries or veins confined to a single-organ (e.g., cutaneous vasculitis, testicular vasculitis, or isolated aortitis). Isolated aortitis or clinically isolated aortitis (CIA) is an example of SOV that affects the aorta and causes aneurysmal dilatation. Nevertheless, this entity does not demonstrate any of the clinical features of systemic vasculitides and is often found incidentally on surgical pathology. The histopathology of CIA and c-GCA/LV-GCA is indistinguishable, and both diseases have a granulomatous/giant cell pattern of inflammation.
Vasculitis associated with systemic disease
This is an entity characterized by large vessels affected by any systemic disease and is also known as secondary vasculitides . The wide spectrum of diseases affecting large vessels typically include a prefix term, such as rheumatoid, lupus, IgG4-related disease, or sarcoid. Rheumatoid arthritis is a common cause of LVV, with a 5.3% prevalence of aortitis.
Infectious vasculitis of the large vessels
This disorder is uncommon but is associated with high mortality and morbidity rates. Usually, vascular infection occurs when there is an abnormality of the vessel wall, such as with atherosclerosis, aneurysm, or graft repairs. Bacteria is the most common pathogen, including Staphylococcal species, Salmonella, Treponema pallidum, and Mycobacterium tuberculosis.
Multimodality imaging of large-vessel vasculitides
Tissue biopsy of large vessels is not feasible. Therefore, imaging plays an important role in the diagnosis and management of large-vessel vasculitides. Currently available modalities for anatomic or morphologic vascular assessment include ultrasound (US), computed tomography angiography (CTA), high-resolution cranial magnetic resonance imaging (MRI) of the superficial arteries, magnetic resonance angiography (MRA), and functional and molecular imaging positron emission tomography (PET)/CT. Invasive or catheter-based imaging is a part of the diagnosis and is still included in the ACR diagnostic criteria. Nevertheless, this technique assesses only the luminal changes and does not provide information about the vessel wall; therefore, it is reserved for treatment purposes only (e.g., balloon angioplasty/stenting of stenotic arterial segments). Each modality can be used individually or in combination, based on clinical questions, and they can provide unique information regarding the extent and severity of vascular abnormalities, which are helpful in tracking response to therapy.
Morphologic imaging
Ultrasound
US/color Doppler US is a well-established imaging modality for vessel assessment in patients with suspected vasculitis. It is easy to perform, cost effective, and does not require exposure to ionizing radiation or IV contrast. Two main imaging features or signs are described as characteristics of vasculitis: (1) the so-called halo sign ( Fig. 30.2 ) and (2) the compression sign. , The halo sign describes an inflamed, edematous, and thickened vessel wall that appears as a hypoechoic rim around the vessel lumen. The compression sign refers to the persistence of visible lumen after compression with the US transducer, as a result of the inflamed and thickened vessel wall. In contrast, a normal temporal artery is completely collapsible after compression with the US probe and is extremely difficult to differentiate from the adjacent soft tissue. Although both signs have been introduced as imaging biomarkers for c-GCA/temporal arteritis, they can be applied to other body parts as well, including the extremity arteries and vasculitis types such as TAK. , US for c-GCA was introduced in the late 1990s, and since then, extensive data on the performance characteristics of this modality have been presented. Compared with the gold standard of temporal artery biopsy, the pooled sensitivity and specificity of the halo sign for c-GCA diagnosis are 77% and 96%, respectively, and the specificity can be increased when the compression sign is added. Nevertheless, a multicenter study that examined the clinical effectiveness of US compared with temporal artery biopsy in 381 patients found low sensitivity (54%) and high specificity (81%) for the diagnosis of GCA with US when only clinical diagnosis was used as the reference standard. Compared with temporal artery biopsy, sensitivity and specificity were 39% and 100%, respectively. The European League Against Rheumatism (EULAR) recommends US of the temporal arteries and axillary arteries as a first-line imaging modality in patients with suspected predominantly cranial GCA. One of the major limitations of US is that it is operator dependent, which could explain the wide range of sensitivities in published studies. Another limitation is that it is not suitable for intrathoracic or intraabdominal vessels. Therefore, in clinically suspected LVV with negative temporal/extremity artery US or temporal artery biopsy, vasculitis involving the aorta may still be present, and additional imaging is required. US is not well established for disease monitoring, although the halo sign can resolve with treatment.
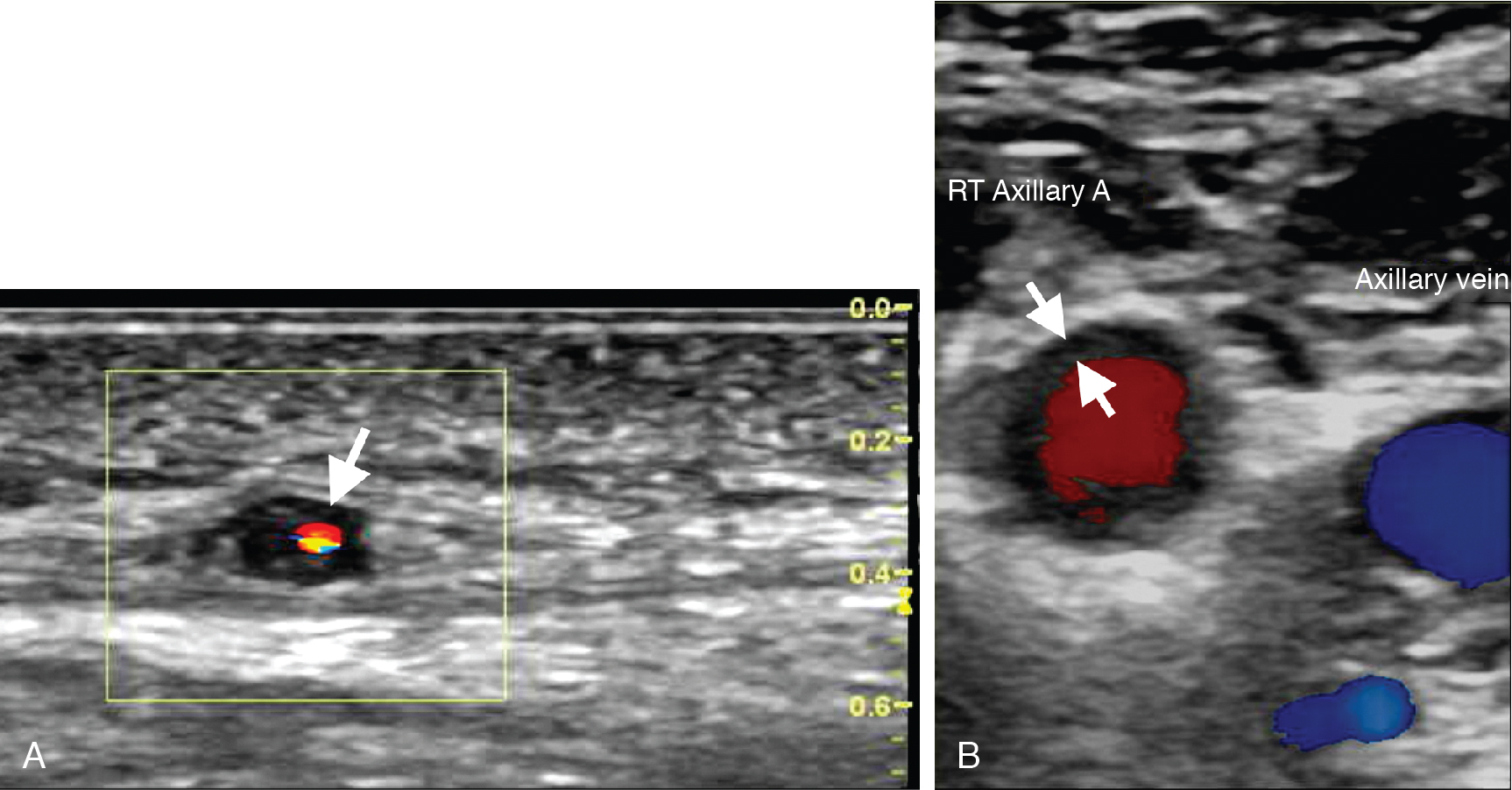
Computed tomography angiography
CT has excellent spatial resolution for detecting abnormalities of large-vessel walls in patients with suspected vasculitis. Advantages of CTA include wide accessibility, convenience, a faster scan time, and coverage of the whole aorta and branches. It can be used for the diagnosis of any type of LVV, including TAK and GCA. Classic imaging features for inflammatory vasculitis are circumferential mural thickening and enhancement, perivascular fat stranding, and associated luminal changes, including narrowing/stenosis or aneurysmal dilatation ( Fig. 30.3 ). Diffuse circumferential mural thickening of the vessel wall of more than 2 to 3 mm is considered a positive finding for vasculitis. , Mural enhancement in CCTA images, particularly on delayed images, is considered a sign of active inflammation, which can resolve quickly after glucocorticoid treatment, whereas thickening of the vessel wall can persist for a longer period of time. , The presence of circumferential mural thickening along the vessel wall is a key feature, compared with focal thickening, which could be because of atherosclerotic plaques or infectious vasculitis. Focal perivascular inflammation with adjacent outpouching of the vessel wall on CCTA is a feature of infectious vasculitis or the so-called mycotic aneurysm ( Fig. 30.4 ). Precontrast images help differentiate intramural hematoma (IMH) from vasculitis ( Fig. 30.5 ). IMH presents with high attenuation (>50 Hounsfield units [HU]) on precontrast images compared with the low attenuation (<40 HU) of the inflamed vessel wall of vasculitis. Complications associated with vasculitis can be imaged with CCTA, including vessel dissection, severe stenosis of the major aortic branches, and aneurysmal dilatation of the aorta. The addition of CCTA can detect a higher prevalence of coronary artery abnormalities (28% ostial stenosis, 40% nonostial stenosis, and 8% aneurysmal dilatation) in TAK patients regardless of their symptoms ( Fig. 30.6 ).
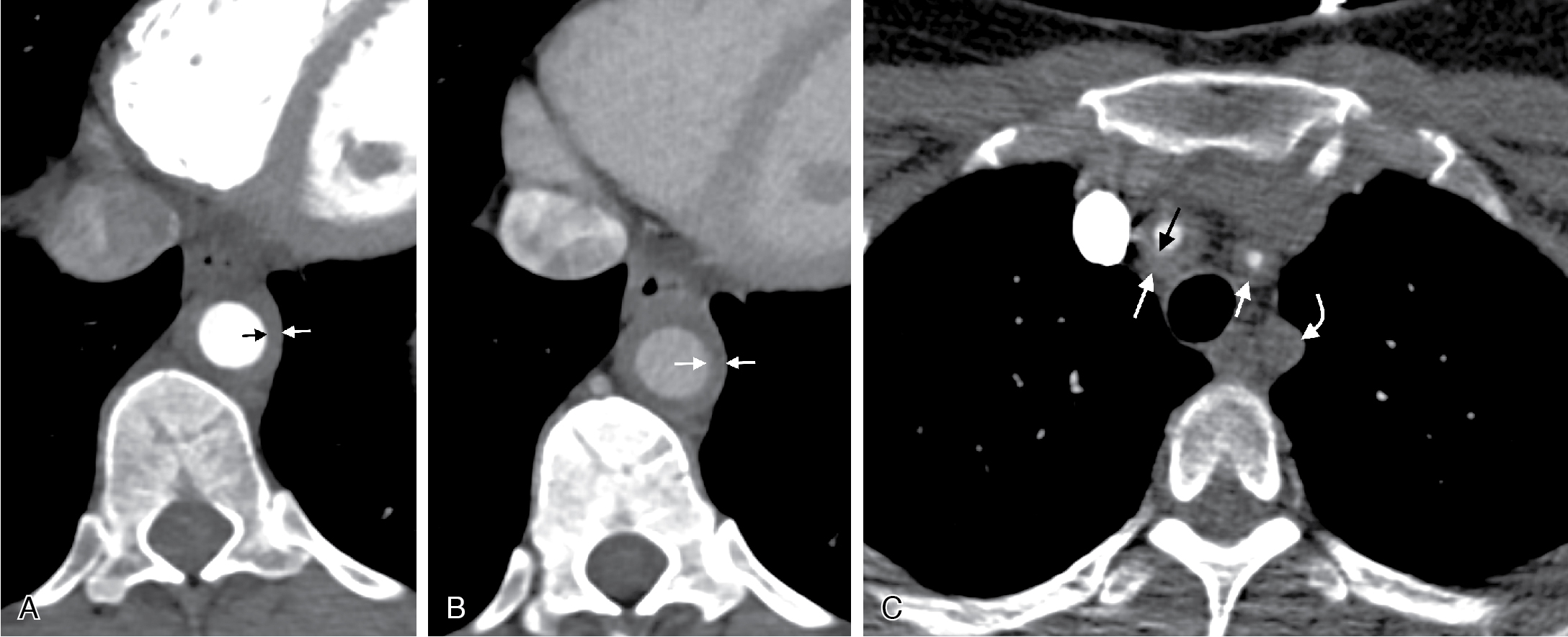
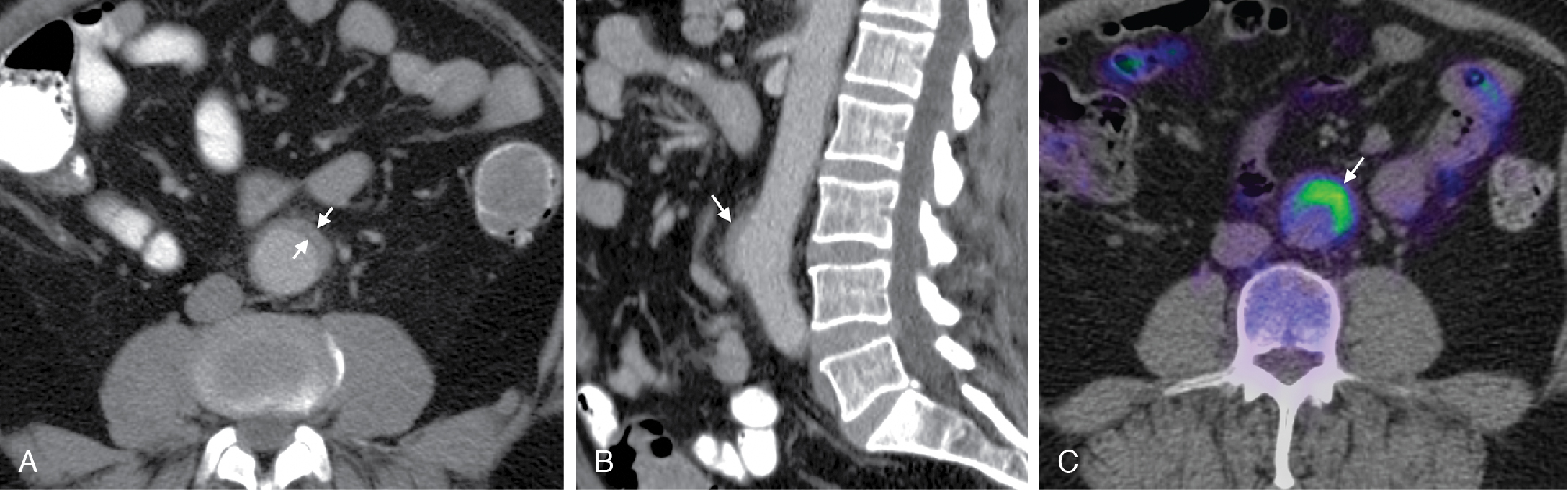
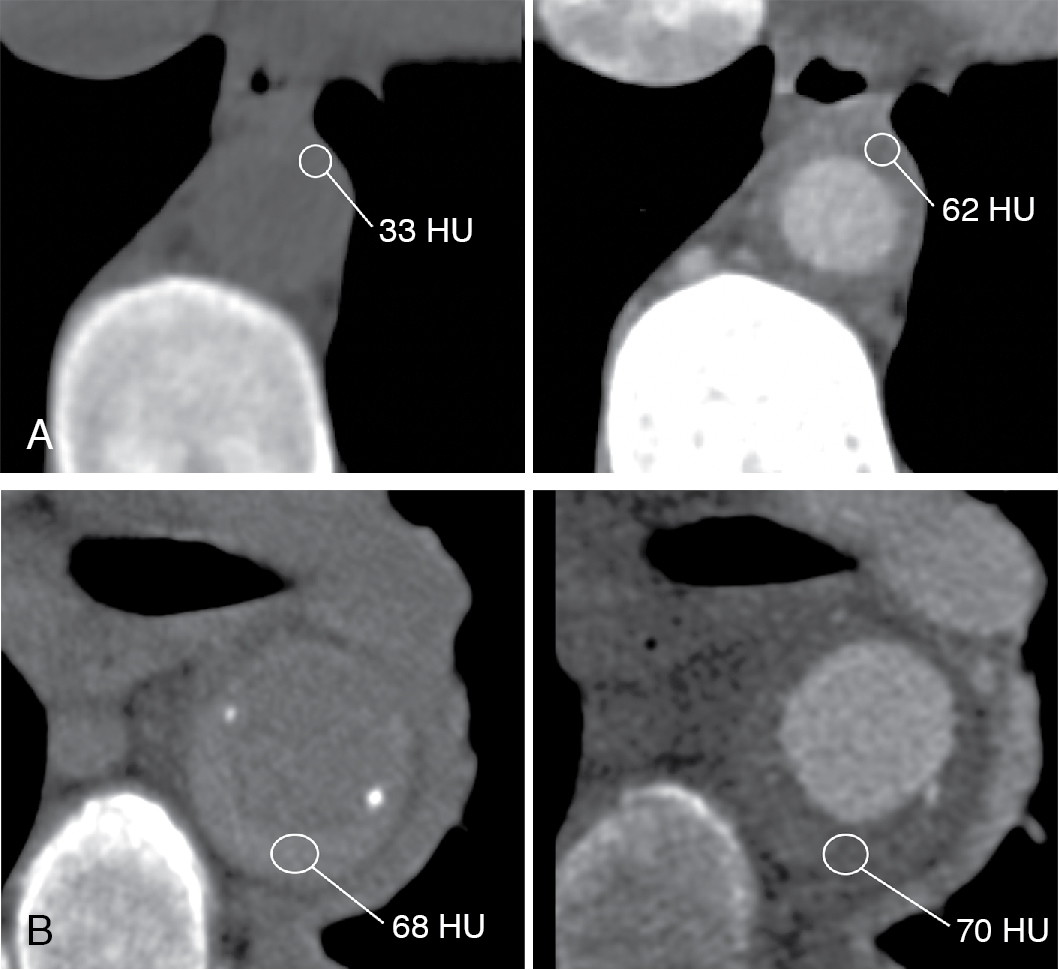
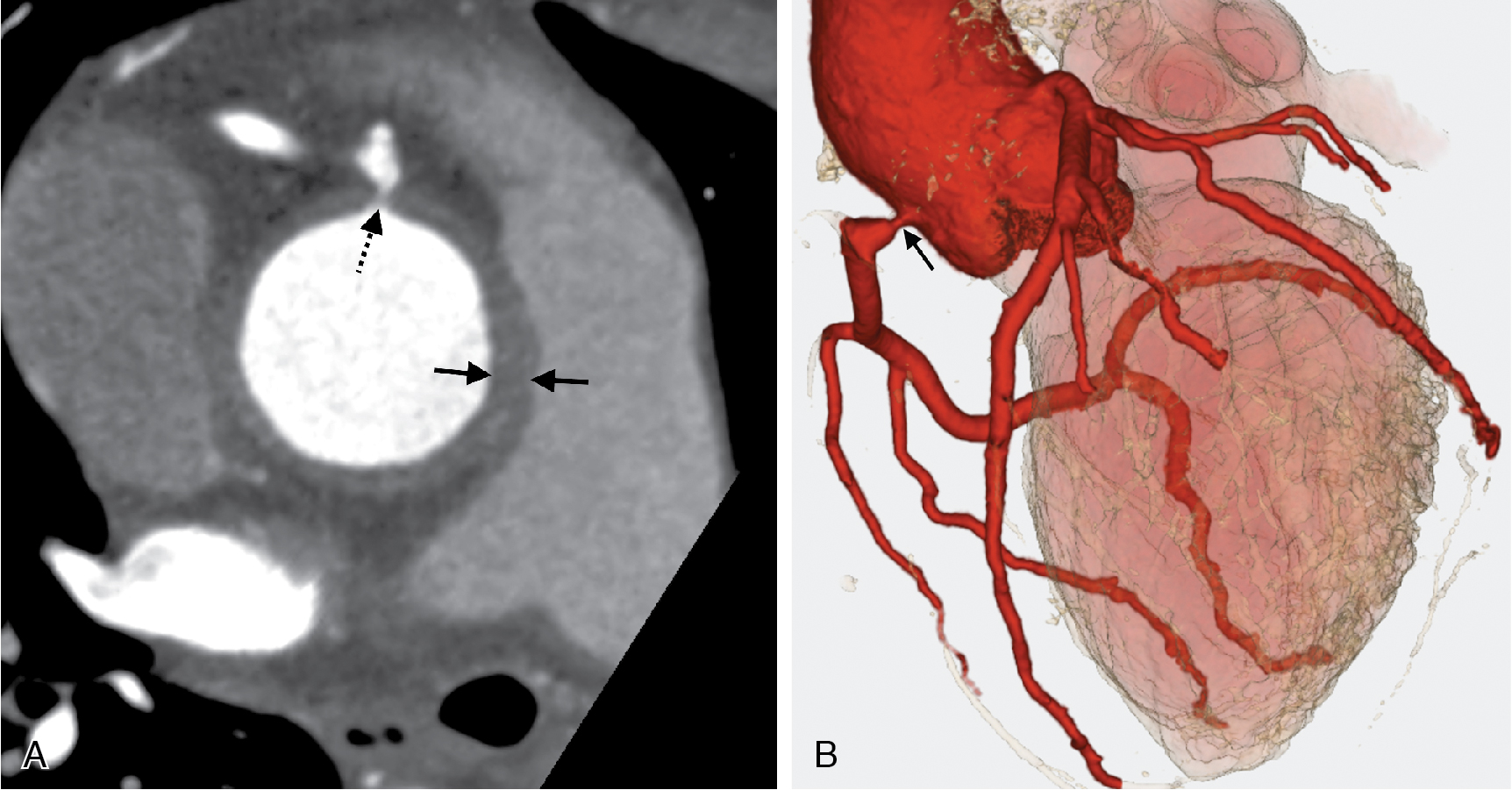
There are sparse data in the literature to support the diagnostic accuracy of CCTA. One study reported a sensitivity and specificity of 73% and 78%, respectively, for the diagnosis of LV-GCA. One of the major limitations of this modality is radiation exposure, particularly for young patients for whom serial/multiple imaging is being considered. Another disadvantage is associated with iodine contrast injection, which cannot be done easily in patients with an iodine allergy or kidney dysfunction.
High-resolution cranial magnetic resonance imaging
High-resolution cranial MRI is an alternative imaging modality to US for the assessment of scalp arteries, particularly temporal arteritis via a postcontrast T1-weighted fat-saturated spin-echo MRI sequence ( Fig. 30.7 ). This modality was initially introduced in 2005 to diagnose c-GCA, and one of its major benefits is that all of the head vessels, including cranial and extracranial branches, can be evaluated at the same time. A large prospective study showed a sensitivity and specificity of 94% and 78%, respectively, for the diagnosis of c-GCA, when temporal artery biopsy was used as a gold standard. Similar to US, however, sensitivity was significantly lower (39%) when the clinical diagnosis based on ACR criteria was used as the reference standard. In the same study, the negative predictive value of MRI was reported to be as high as 98.2% (95% confidence interval [CI], 93.6 to 99.8), which could be used to avoid temporal artery biopsies when temporal arteries are normal on MRI. High-resolution cranial MRI, which is important for early diagnosis, is not yet widely adopted because of its limited availability and challenges with the interpretation of the studies/images. EULAR recommends high-resolution cranial MRI as an alternative imaging modality for c-GCA diagnosis when US is not available or inconclusive.
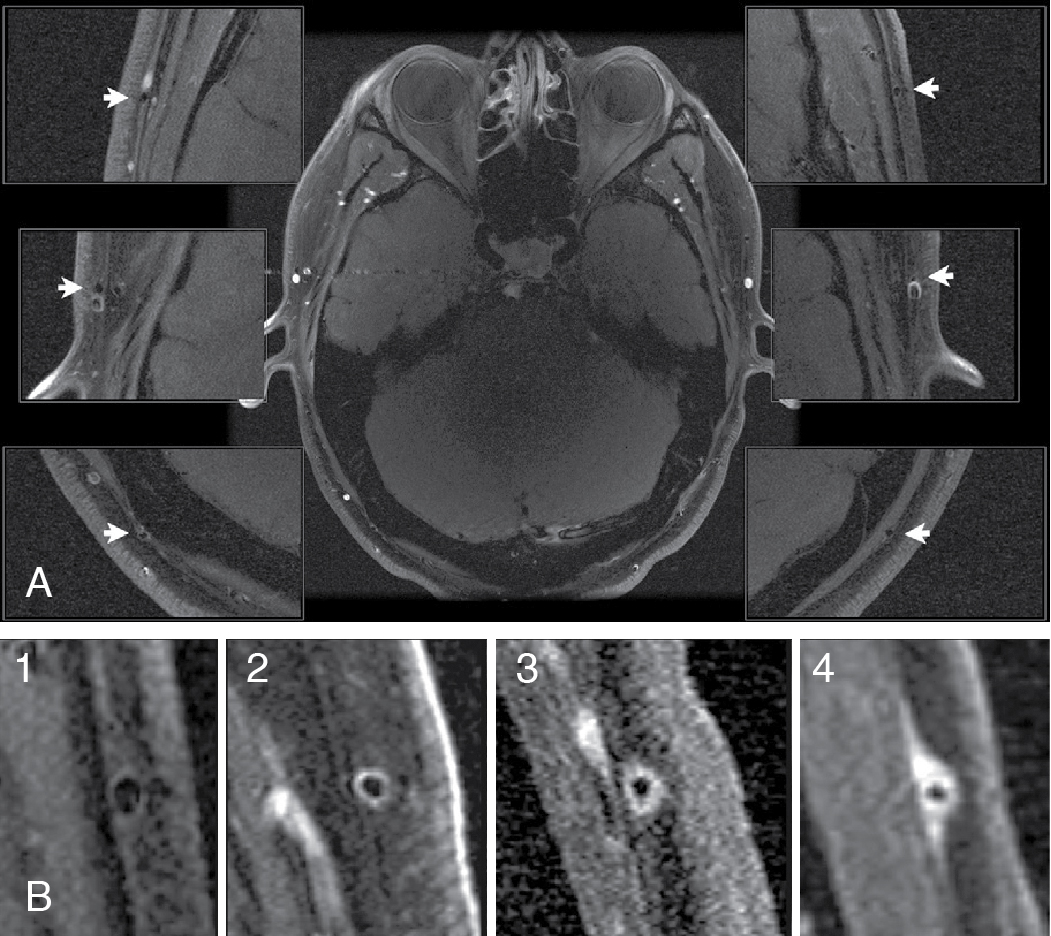
Magnetic resonance angiography
MRA has been used to assess multiple pathologies of the aorta and its main branches. Although it has slightly lower spatial resolution compared with CCTA, the tissue contrast resolution (tissue characterization) is superior to any other modality. Therefore, MRA can provide a detailed assessment of the mural changes in the large-vessel wall. Another advantage of MRA is that because there is no associated radiation exposure, it can be used for the longitudinal follow-up of younger patients throughout the course of the disease.
Characteristic imaging features ( Fig. 30.8 ) of LVV in MRA images are diffuse, circumferential mural thickening of the vessel wall; associated edema on T2-weighted images; and enhancement on postcontrast images. In addition, luminal narrowing or aneurysmal dilatation can be seen easily on maximum intensity projection (MIP) images. On delayed postcontrast images (a spoiled three-dimensional [3D] gradient echo sequence), the enhancing component of the aortic wall (adventitia and media) and the nonenhancing intima create a “double-ring sign.” Similar to CCTA, focal fat stranding and vessel outpouching on MRA images are consistent with mycotic aneurysm in the appropriate clinical context.
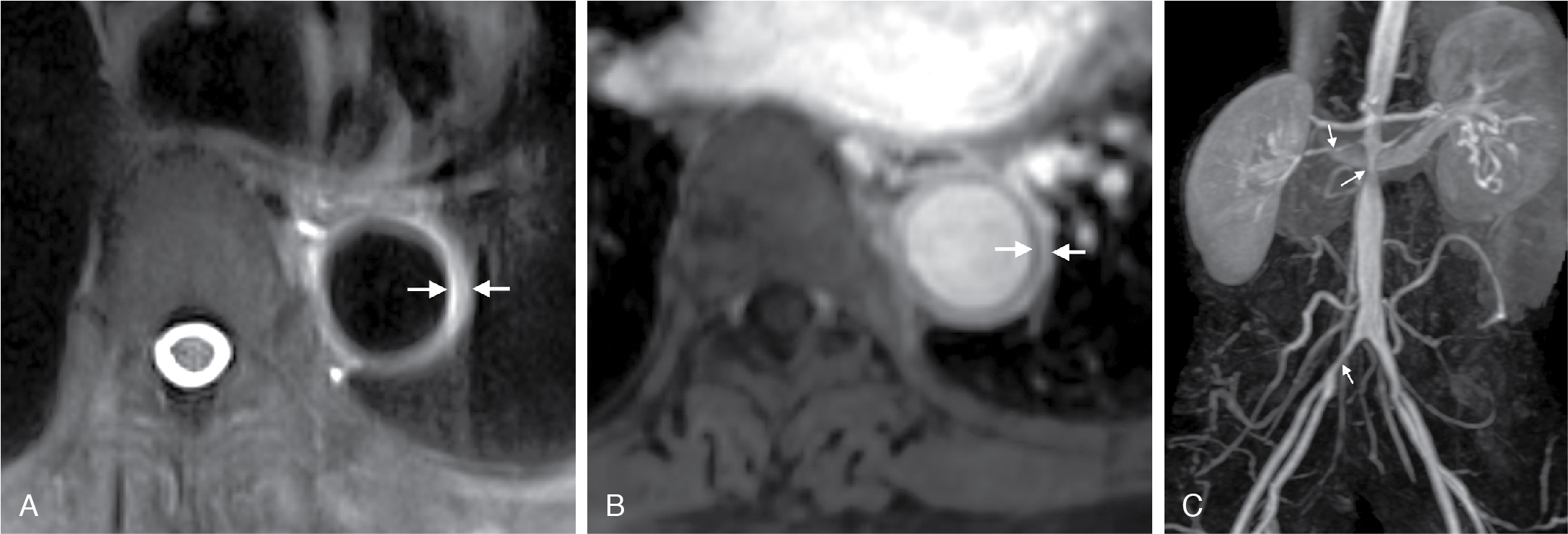
Although MRA can be used in any LVV type, most of the literature is based on TAK, particularly because of the young age range of this population. Multiple studies have compared postcontrast MRA images with invasive digital subtraction angiography and have shown a strong correlation regarding the stenotic segments of the large vessels. Some studies have demonstrated mural edema in the thickened wall on T2-weighted images, which could be a sign of active inflammation/vasculitis. , Recent studies, however, raise concerns about this finding, and it is considered that edema on T2-weighted images is less sensitive and prone to artifact. , Another important imaging feature in vasculitis is the enhancement of the thickened vessel wall, which is considered to be a sign of active inflammation. , A study by Quinn et al. compared MRA with fluorodeoxyglucose (FDG) PET/CT in LVV (both GCA and TA) patients and found that MRA better demonstrated the disease extent; however, there was a lack of correlation between disease/vascular inflammation based on FDG PET and MRA findings, particularly when it came to the enhancement of the thickened wall. Enhancement of the thickened wall could be because of only vessel remodeling/fibrosis rather than active inflammation. Therefore concentric vessel wall thickening and the associated enhancement are excellent imaging features to diagnose vasculitis; however, disease activity cannot be assessed reliably. , Given the tissue resolution of MRA, it is an ideal imaging modality for differentiating other etiologies (such as IMH, infection, or aortic wall mass) from vasculitis. Similar to CCTA, the main limitations of MRA are the sparse literature and lack of standardized definitions of imaging abnormalities for diagnosis and follow-up.
Molecular/metabolic imaging
Fluorodeoxyglucose positron emission tomography/computed tomography
As described in Chapter 23 , FDG accumulates in inflammatory cells, particularly in the monocytes and macrophages, because of high glycolytic activity. Therefore inflammatory cells in the thickened and inflamed vessel wall from vasculitis can accumulate FDG and be used in PET/CT imaging. FDG PET/CT for LVV was first introduced in the late 1990s as an accidental discovery in patients with fever of unknown origin. Since then, it has emerged as a promising imaging modality in the diagnosis of LVVs. Patient preparation includes 6 hours of fasting before the tracer injection and limiting strenuous exercise before the study. In patients with suspected aortic root or coronary involvement, it is necessary to suppress FDG uptake in normal myocardium, as described in Chapter 23 . Delayed image acquisition (at 180 minutes after tracer injection) is beneficial for vasculitis patients. Recently, FDG PET/CT has been used to diagnose head and neck vessels, particularly temporal arteries, and thus c-GCA. ,
Classic imaging findings of FDG PET/CT in patients with inflammatory vasculitis include segmental intense FDG uptake along the aorta and main branches ( Fig. 30.9 ). FDG PET/CT is also a valuable modality for the diagnosis of PMR as a type of inflammation around the joint/synovium, given its overlap with GCA and often coexistence in the same patient ( Fig. 30.10 ). Combining CCTA or MRA with FDG PET can help assess luminal narrowing or complications associated with active versus chronic vasculitis. , The absence of mural thickening on CCTA or MRA does not exclude the presence of vasculitis and should not limit the use of FDG PET/CT, especially when there is high clinical suspicion. Lastly, FDG uptake in patients with LV-GCA is associated with later aortic dilatation; therefore, close follow-up of these patients should be considered. Infectious vasculitis displays focal FDG uptake associated with mycotic aneurysm and adjacent infectious structures (see Fig. 30.4 C).
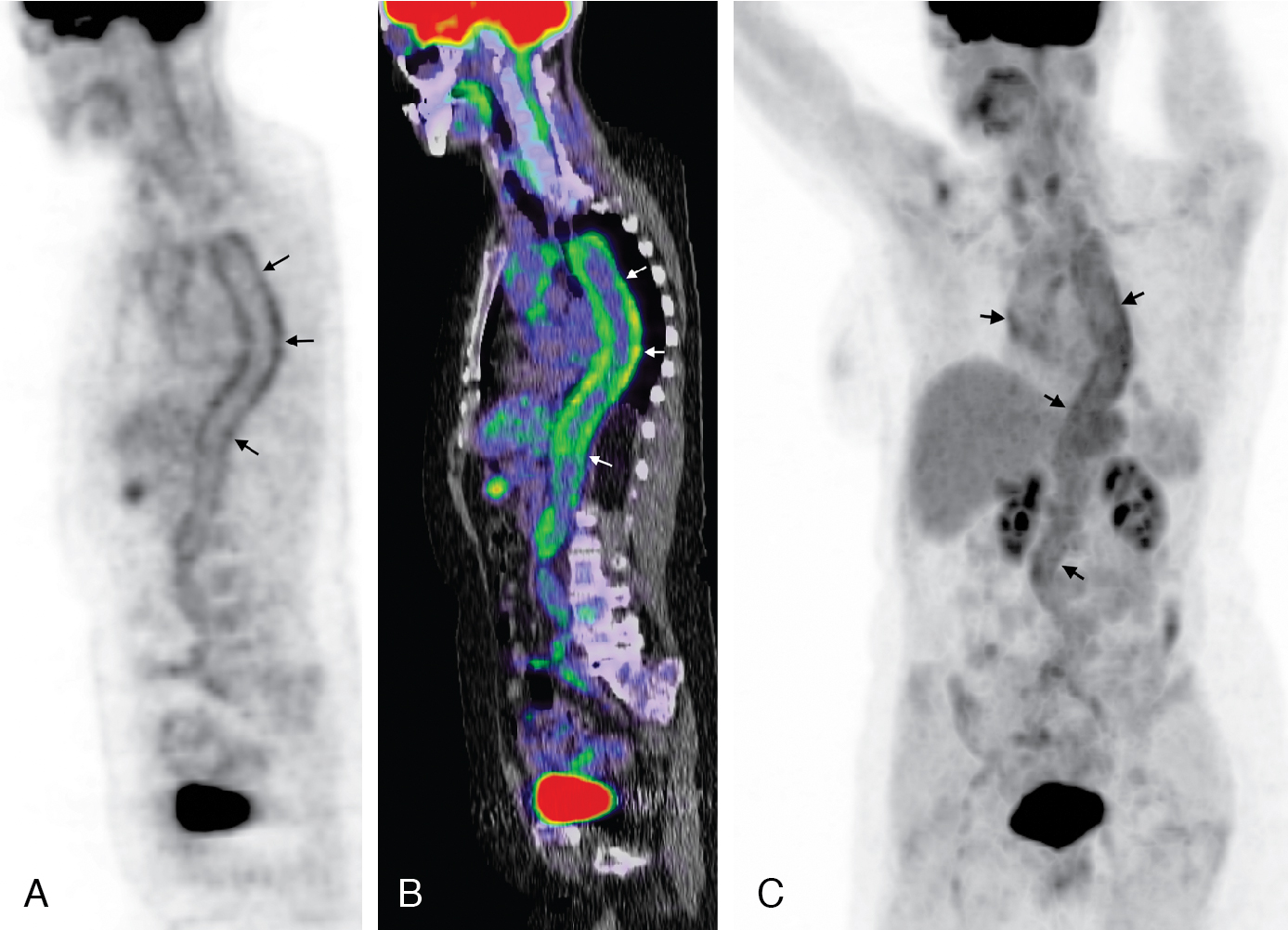
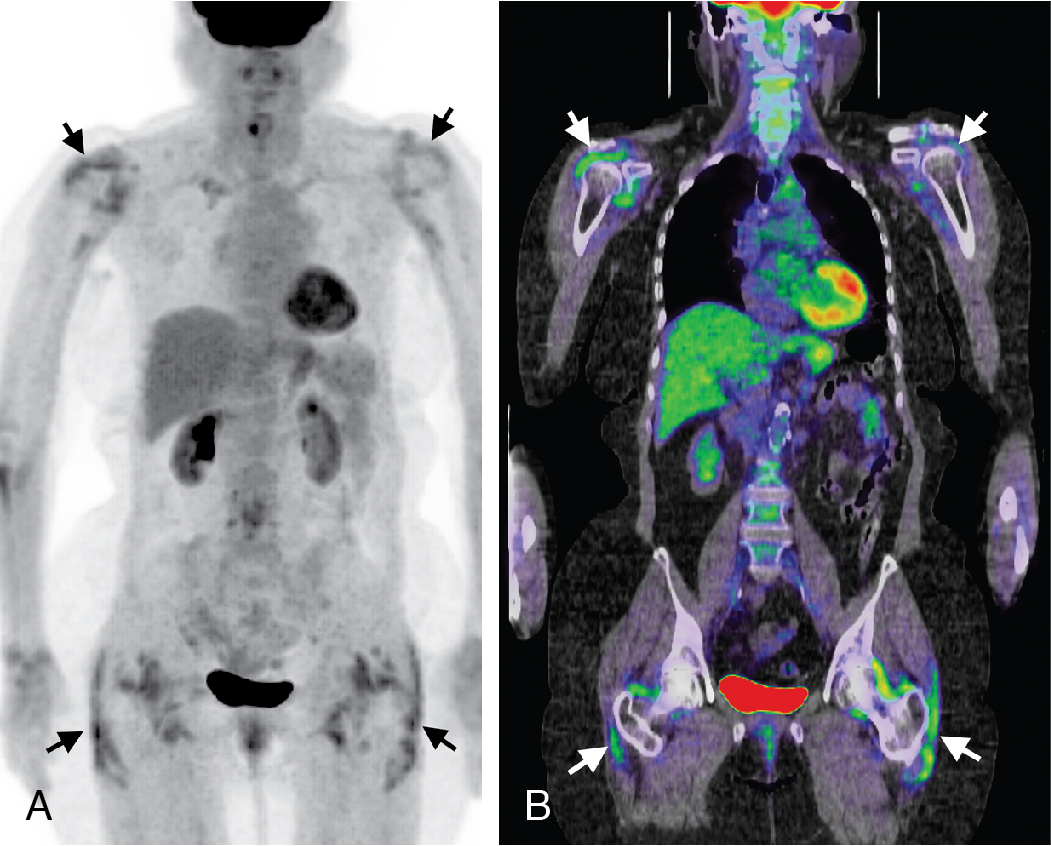
Various interpretation criteria have been proposed, including visual interpretation and semiquantitative techniques. The Society of Nuclear Medicine and Molecular Imaging and the European Association of Nuclear Medicine proposed visual interpretation in routine clinical settings. With this technique, vascular FDG uptake is compared with the liver using a four-point grading system ( Fig. 30.11 ):
- •
Grade 0 = no uptake (< mediastinal or blood pool uptake),
- •
Grade 1 = low-grade uptake (< liver),
- •
Grade 2 = intermediate-grade uptake (= liver),
- •
Grade 3 = high-grade uptake (> liver).
