Performing intraoperative cardiovascular procedures inside an MR imaging scanner can potentially provide substantial advantage in clinical outcomes by reducing the risk and increasing the success rate relative to the way such procedures are performed today, in which the primary surgical guidance is provided by X-ray fluoroscopy, by electromagnetically tracked intraoperative devices, and by ultrasound. Both noninvasive and invasive cardiologists are becoming increasingly familiar with the capabilities of MR imaging for providing anatomic and physiologic information that is unequaled by other modalities. As a result, researchers began performing animal (preclinical) interventions in the cardiovascular system in the early 1990s.
Key points
- •
Advantages of MR imaging for cardiac interventions include visualizing vessel lumen and wall anatomy, scar tissue, acute ischemia and hemorrhage.
- •
Therapeutic outcomes may be improved utilizing real-time MR monitoring.
- •
MR imaging–guided cardiac intervention is finally becoming a clinical reality, after years of technological development and preclinical testing.
- •
Cardiac electrophysiology (EP) is the first field for which clinical grade device development is complete and in which human clinical trials are currently underway.
Introduction
Specific issues that result from performing procedures inside the beating heart are as follows: (1) the complex and nonrigid respiratory and cardiac motion of the heart affects both MR imaging and MR imaging–guided navigation and (2) the lack of robustness of MR imaging, in tissues other than the lumen and wall of the major vessels, makes the imaging of small and moving vessels difficult. A practical result of the current MR imaging limitations has been the concentration of efforts on regions where diagnostic cardiac MR imaging is more mature, namely, procedures in the greater cardiac vessels such as the ventricles and the atria.
With diagnostic cardiac MR imaging increasingly available in hospitals and clinics throughout the world, the most well-developed MR imaging–guided procedures are those in which the cardiac MR imaging is performed before or after the actual intervention, while the interventional procedure itself is performed in the classical X-ray-guided operating room (OR) or interventional suite. All commercial medical imaging equipment vendors now offer “X-MR suites,” which are MR imaging scanners and X-ray interventional suites that share a transport table, facilitating patient transfer between the imaging and interventional modalities. Commercial image processing tools are also available from these vendors and from the major catheter companies, which allows transformation of the images from the MR imaging to the X-ray or OR frame of reference (which can also be the frame of reference of an interventional workstation with integrated electromagnetic positional tracking ). As a result, in preoperative planning of cardiac EP procedures, in valve repair procedures, and for implantable cardioverter-defibrillator (ICD) placement procedures, use of MR vascular and scar images has become routine. Excellent reviews on these subjects are available.
This review focuses on intraoperative use of MR imaging during cardiovascular interventions. This field is clinically in its infancy. Present work is primarily dedicated to removing some of the major hurdles to its use, although several clinical efficacy studies have recently emerged. Prior reviews on this subject exist.
Although MR imaging–guided procedure development began with coronary intervention, the greatest effort today is on MR imaging–guided therapeutic cardiac EP, primarily using radiofrequency ablation. It is clear that MR imaging provides several imaging contrasts that are useful for the diagnosis of chronic (or temporally stable) cardiac disease. The ability to visualize fat, see scar tissue, detect fibrosis, separate edema and hemorrhage from myocardial tissue, visualize the vascular tree, and assess mechanical function are well-known advantages of MR imaging. In the context of intraoperative MR imaging, however, there is also a need to assess acute or temporally changing conditions. Understanding the appearance of pathologic conditions during the interventional procedure is an evolving field that may weigh heavily on the ultimate utility of MR imaging–guided procedures in the heart. Predicting the ultimate state of myocardium from its intraoperative appearance, where transient effects such as blood by-products, edema, hemorrhage, and reduced perfusion are prevalent, is a challenge that several research groups are currently addressing.
In addition, the need to provide solutions for emergency interventions inside the MR imaging suite is a challenge that must be resolved, because large patient populations are excluded from MR imaging–guided interventions because of the lack of equipment for rapidly detecting and treating cardiac events.
The most dramatic recent progress has been in the field of MR imaging–guided EP. (1) In Europe, clinical trials are being carried out to assess the effectiveness of MR imaging–guided atrial ablation. (2) Several entrepreneurial companies, such as Imricor Medical Systems, Inc, and MRI Interventions, Inc, are developing MR imaging–compatible clinical grade EP catheters, sheathes, and guidewires in collaboration with major MR imaging vendors, such as Philips Healthcare and Siemens Healthcare. (3) It has been established that there is close agreement between the EP intracardiac voltage mapping as performed with commercial electroanatomic mapping (EAM) systems, such as the NavX-Velocity (St. Jude Medical, Inc, St Paul, MN, USA) or the CarTo3 (Biosense-Webster, Inc, Diamond Bar, CA, USA), and MR imaging–based late gadolinium enhancement (LGE) images in the case of chronic ischemic lesions. (4) There are pioneering results on visualizing acute tissue necrosis using T1 or T2* mapping methods, which are critical to the use of MR imaging for monitoring the quality of ablation lesions in the intraoperative acute setting.
Introduction
Specific issues that result from performing procedures inside the beating heart are as follows: (1) the complex and nonrigid respiratory and cardiac motion of the heart affects both MR imaging and MR imaging–guided navigation and (2) the lack of robustness of MR imaging, in tissues other than the lumen and wall of the major vessels, makes the imaging of small and moving vessels difficult. A practical result of the current MR imaging limitations has been the concentration of efforts on regions where diagnostic cardiac MR imaging is more mature, namely, procedures in the greater cardiac vessels such as the ventricles and the atria.
With diagnostic cardiac MR imaging increasingly available in hospitals and clinics throughout the world, the most well-developed MR imaging–guided procedures are those in which the cardiac MR imaging is performed before or after the actual intervention, while the interventional procedure itself is performed in the classical X-ray-guided operating room (OR) or interventional suite. All commercial medical imaging equipment vendors now offer “X-MR suites,” which are MR imaging scanners and X-ray interventional suites that share a transport table, facilitating patient transfer between the imaging and interventional modalities. Commercial image processing tools are also available from these vendors and from the major catheter companies, which allows transformation of the images from the MR imaging to the X-ray or OR frame of reference (which can also be the frame of reference of an interventional workstation with integrated electromagnetic positional tracking ). As a result, in preoperative planning of cardiac EP procedures, in valve repair procedures, and for implantable cardioverter-defibrillator (ICD) placement procedures, use of MR vascular and scar images has become routine. Excellent reviews on these subjects are available.
This review focuses on intraoperative use of MR imaging during cardiovascular interventions. This field is clinically in its infancy. Present work is primarily dedicated to removing some of the major hurdles to its use, although several clinical efficacy studies have recently emerged. Prior reviews on this subject exist.
Although MR imaging–guided procedure development began with coronary intervention, the greatest effort today is on MR imaging–guided therapeutic cardiac EP, primarily using radiofrequency ablation. It is clear that MR imaging provides several imaging contrasts that are useful for the diagnosis of chronic (or temporally stable) cardiac disease. The ability to visualize fat, see scar tissue, detect fibrosis, separate edema and hemorrhage from myocardial tissue, visualize the vascular tree, and assess mechanical function are well-known advantages of MR imaging. In the context of intraoperative MR imaging, however, there is also a need to assess acute or temporally changing conditions. Understanding the appearance of pathologic conditions during the interventional procedure is an evolving field that may weigh heavily on the ultimate utility of MR imaging–guided procedures in the heart. Predicting the ultimate state of myocardium from its intraoperative appearance, where transient effects such as blood by-products, edema, hemorrhage, and reduced perfusion are prevalent, is a challenge that several research groups are currently addressing.
In addition, the need to provide solutions for emergency interventions inside the MR imaging suite is a challenge that must be resolved, because large patient populations are excluded from MR imaging–guided interventions because of the lack of equipment for rapidly detecting and treating cardiac events.
The most dramatic recent progress has been in the field of MR imaging–guided EP. (1) In Europe, clinical trials are being carried out to assess the effectiveness of MR imaging–guided atrial ablation. (2) Several entrepreneurial companies, such as Imricor Medical Systems, Inc, and MRI Interventions, Inc, are developing MR imaging–compatible clinical grade EP catheters, sheathes, and guidewires in collaboration with major MR imaging vendors, such as Philips Healthcare and Siemens Healthcare. (3) It has been established that there is close agreement between the EP intracardiac voltage mapping as performed with commercial electroanatomic mapping (EAM) systems, such as the NavX-Velocity (St. Jude Medical, Inc, St Paul, MN, USA) or the CarTo3 (Biosense-Webster, Inc, Diamond Bar, CA, USA), and MR imaging–based late gadolinium enhancement (LGE) images in the case of chronic ischemic lesions. (4) There are pioneering results on visualizing acute tissue necrosis using T1 or T2* mapping methods, which are critical to the use of MR imaging for monitoring the quality of ablation lesions in the intraoperative acute setting.
Topics
Coronary Artery Interventions for the Diagnosis or Treatment of Coronary Artery Disease
Coronary artery disease (CAD) is one of the most frequent causes of patient morbidity. The common intervention for CAD is X-ray-fluoroscopy-guided angioplasty in which the culprit (partially or completely) occluded vessels are either opened by balloon expansion followed by stent placement or replaced with a grafted bypassing vessel.
There are several reasons for replacing X-ray as the modality used for procedural guidance. (1) X-rays can visualize only the vessel lumen and not the surrounding soft tissue such as the vessel wall; this reduces the clinician’s ability to gauge the extent of injury to surrounding myocardial tissue during the procedure or the amount of restenosis that occurs afterward because of soft tissue regrowth. (2) When the coronary vessel is entirely occluded, chronic total occlusion (CTO), X-rays cannot visualize the occluded region because contrast cannot enter this region, which makes it difficult to guide a device to the proper location for opening the occlusion. Such poor visualization may result in vessel perforation. (3) The X-ray dose that clinicians carrying out coronary procedures are exposed to is quite high, which limits the number of procedures they perform. In addition, the heavy lead aprons clinicians wear to shield from radiation are a significant cause of back pain.
Another role for MR imaging guidance is to monitor the treatment of preexisting cardiac myocardial infarcts. Several innovative therapeutic strategies have been proposed to prevent the remodeling of the myocardium postinfarction and the ensuing progression to heart failure. There are also experimental therapies to induce the (re)growth of myocytes within the infarct such as the induction of specific pharmaceuticals, viruses, and stem cells. For these applications, MR imaging–guided therapy delivery is highly advantageous because of the following reasons. (1) MR imaging visualizes the infarct borders, including the inactive but perfused (stunned) myocardium that surrounds the necrotic area, identifying optimal regions for therapy delivery. (2) MR imaging can monitor the concentration of agents that enters into the injected region during the delivery process. (3) Longitudinal MR imaging can be used to assess the effectiveness of therapy, such as the improvement in myocardial mechanical function.
Coronary vascular access
In animal models, MR imaging–guided navigation through the arterial tree and into the coronary artery was performed early on, using dedicated MR imaging–compatible guidewires and sheathes.
The invasive devices were visualized (1) passively, by virtue of their enhanced susceptibility to artifacts, usually resulting in a hypointense appearance of the device on MR imaging; (2) semiactively, by adding radiofrequency coils onto portions of the device so that these regions appear hyperintense on MR imaging, or (3) actively, via microcoils placed on the device’s shaft, combined with specific positional tracking sequences to detect the position of these coils. Collectively, such procedures generally required collection of vascular roadmaps using MR angiography, followed by navigation of the devices on these roadmaps by using imaging sequences that provided the position of the devices over time.
Building MR imaging–compatible coronary devices, such as guidewires and sheathes, was a serious challenge because coronary artery diameter is small (<2 mm) and its path tortuous, requiring the construction of small and highly flexible interventional devices. Commercial interventional devices for coronary use require the use of highly specialized materials and fabrication methods. In order to make the devices compatible for MR imaging, it was necessary to replace many of the conventionally used materials (such as stainless steel) with materials with inferior mechanical properties, which sometimes required use of larger dimensions in order to attain equivalent performance. SurgiVision, Inc (now MRI Interventions, Inc) built the first MR imaging–compatible guidewire, based on a simple radiofrequency coil design (the loopless antenna), which was subsequently cleared for clinical use. Other devices were subsequently built by other groups.
In most cases, the MR imaging–guided coronary procedures required much greater time to perform vascular navigation into the coronaries than equivalent X-ray procedures. More importantly, problems with the robustness of MR coronary imaging, such as high-resolution imaging of the lumen or wall of the coronaries, prevented capitalization on the advantages of MR imaging.
In opening coronary CTOs, extensive animal experimentation was performed, and it was shown that MR imaging guidance has distinct advantages that could be captured with greater ease in occlusions in larger vessels. From the scientific perspective, the coronary MR imaging procedures were very instructive. These procedures forced the development of novel MR imaging–compatible materials and taught the field about the complex motion of the coronary arteries. At present, there are 2 companies, MaRVis Medical GmbH, Hannover, Germany, and Nano4imaging GmBH, Aachen, Germany, that are engaged in the construction and testing of MR imaging–compatible guidewires, so MR imaging–guided coronary intervention may soon be reexamined. Some of the devices that were developed for coronary intervention are used for less-complex vascular procedures, such as for MR imaging–guided carotid, aortic, and peripheral interventions.
Ventricular access and therapy for coronary artery disease
Obtaining vascular access into the lumen of right ventricle or left ventricle (LV) was possible from the early days of MR imaging–guided intervention. As traversing the intra-atrial septum with MR imaging was difficult before the development of an MR imaging-compatible transseptal needle, the left side of the heart was mostly accessed through the arterial vasculature.
Injection of therapeutic agents into the infarcted left ventricular wall was performed primarily by the National Heart, Lung, and Blood Institute and Johns Hopkins groups. Much of this work used MR imaging–compatible myocardial injection needles for this purpose ( Fig. 1 ). As initially the stem cells did not survive for a long time after implantation, methods were devised to feed and protect the stem cells in situ.
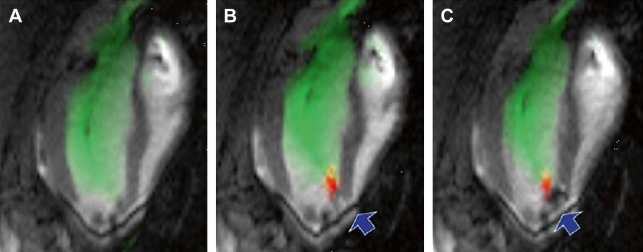
In recent years, most myocardial injections have been performed using a combined X-ray and MR imaging (X-MR) suite, which allows for a faster procedure and reduces the mechanical demands on the MR imaging–compatible myocardial injection needles.
Cardiac Electrophysiology Procedure for the Diagnosis and Treatment of Rhythm Disorders
Some of the pioneering work in MR imaging–guided cardiac interventions was directed at the treatment of EP disorders. This focus resulted from multiple factors. (1) The incidence of ventricular and atrial rhythm disorders is growing rapidly, as the population ages, with the incidence of atrial fibrillation (AF) and ventricular tachycardia (VT) now approaching 4 million and half a million subjects, respectively, in the United States. (2) The pharmaceutical treatments of these diseases are not very effective and carry considerable side effects. (3) Despite the large increase in the number of ablative procedures for treating VT and AF, the success rate for such procedures, as measured by the lack of recurrence of syndromes, is only approximately 50% and 70%, respectively. Much of the failure is attributed to insufficient ablation of the electrical circuits that cause the arrhythmias due to the creation of ablation lesions that are insufficiently deep or interconnected. (4) EP procedures form a major source of income for cardiology departments. There is great commercial interest in developing solutions, with several catheter companies developing clinical systems for diagnosing and treating rhythm disorders. (6) MR imaging of the ventricles has been a robust clinical technique for several years, and with the development of navigator echo methods, several MR imaging contrasts for use in the atrium are commercially available. When the above-mentioned facts are combined with the unique MR imaging intertissue contrasts, MR imaging guidance for EP procedures is quite attractive.
Use of MR imaging or computed tomographic (CT) imaging for preoperative planning, performed before VT or AF procedures, has become routine in the field. CT or MR angiography is used to map the cardiovascular luminal anatomy. When scar tissue from a prior infarct or previous ablations is present in the LV or left atrium (LA), performing preoperative LGE MR imaging to map the scar tissue provides distinct advantages. This technique can reduce the duration and increase the sensitivity of intracardiac EAM, the diagnostic portion of the EP procedure, by pointing to interventional electrophysiologists as to where, within the LV or the LA, they should go with the mapping catheters to map the pathology. This feature becomes extremely important when the scar tissue is located in regions that are difficult to access or contact with the EP mapping catheters. One example is when the scar tissue is located behind the papillary muscle in the LV ( Fig. 2 ). Another important case is when the scar does not cover the entire width of the chamber wall (a nontransmural lesion), where conventional catheter-based EAM requires extensive mapping to provide the scar dimensions. It has recently been shown that some of the LGE image characteristics (intensity, shape) observed in infarcts correlate extremely well with some EAM voltage characteristics (size and shape of voltage), enabling the prediction of the location of reentry circuits within the LV lesions, areas that should be ablated to prevent tachycardia ( Fig. 3 ).
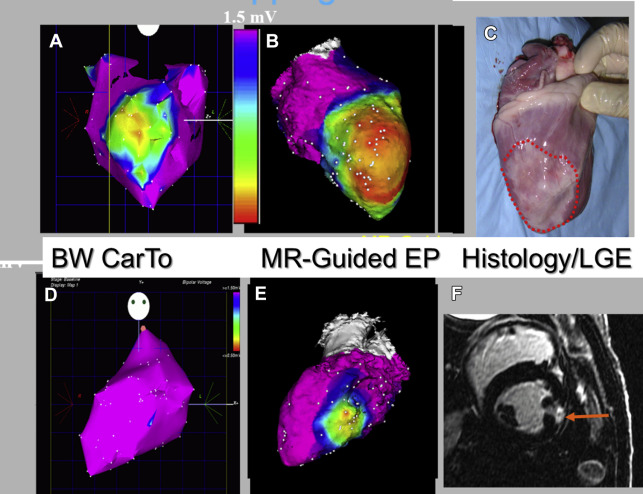
Ventricular Diagnostic and Therapeutic Interventions
This section focuses on the use of interventional methods to diagnose and treat, with radiofrequency ablation, inducible VT, the cause of sudden cardiac death. In brief, the diagnostic approach requires finding the regions within the LV (or right ventricle) where the errant electrical circuits can occur during a cardiac event. The common practice is to move along the LV walls with an EP catheter whose location is identified by the EAM system, deliver a voltage to the wall (pace), and use a second EP catheter to measure the tissue electrical response, which is recorded by the EAM system in the form of an intracardiac electrocardiographic trace (EGM) that is then associated with the specific point on the LV wall. If a strong response is obtained when pacing is performed at a certain location, a potential aberrant circuit is identified. At the end of EAM mapping, the regions identified as possible sources of VT are ablated. These areas can be along the borders of, or within, the scar tissue, which was left over after the heart attack that the subject incurred. After each ablation, catheter-induced pacing is performed, and if the previously recorded EGM tissue response is no longer observed, it is assumed that the source of VT has been terminated. However, this is not always the case. Recurrence occurs if some live circuits are not treated or if some circuits are insufficiently ablated, so that the conducting elements (chains of myocytes) reconnect when transient effects resulting from the ablation (edema, hemorrhage) recede.
Intraoperative use of MR imaging improves the localization of the aberrant circuits, and it can also assist in monitoring the radiofrequency ablation process, by determining that the lesions created are sufficient to prevent recurrence of inducible VT.
Although LV interventions are easier to perform from the MR imaging perspective, they primarily treat life-threatening diseases such as sudden cardiac death. As a result, a LV ablation procedure typically requires a high standard of physiologic monitoring, such as for rapid detection of acute ischemia, via the clear observation of ECG S-T segment elevation, and/or for the rapid detection of ventricular fibrillation. There is also a need for MR imaging–compatible means for rapid resuscitation, principally based on a defibrillation unit that can be rapidly used within the MR imaging suite. An additional issue with performing ventricular interventions is that many of the LV patients already have implanted pacemakers or ICDs. Although increasing numbers of MR imaging sites are performing diagnostic cardiac MR imaging on such patients, most of the sites shy away from the increased procedural risk that may be incurred because of malfunctioning of these devices inside the MRI.
As the required monitoring and resuscitation devices are not yet available, LV EP therapeutic interventions have only been performed in animals. MR imaging–compatible 12-lead ECG and defibrillation systems are currently under development, so human MR imaging–guided ventricular ablation procedures may begin soon.
An integrated MR imaging–guided EP laboratory, which included many of the devices and visualization tools required for the performance of interventions inside the MR imaging, was constructed in the mid-2000s as a collaboration between General Electric and St. Jude Medical and installed at Massachusetts General Hospital. MR imaging–compatible radiofrequency ablation generators, ablation catheters, and sheathes were developed at that time. Animal interventions were performed, demonstrating the clinical advantages of MR imaging–guided EP ablation. One of the chief novelties of the system was use of MR-tracking-based catheter positional localization, which required that the entire toolset ( Fig. 4 ) be equipped with MR-tracking coils. MR tracking provided catheter navigation capabilities of a similar spatial resolution and temporal rate ( Fig. 5 ) as available in the conventional EP laboratory with EAM mapping systems, which rely on electromagnetic tracking. Without MR tracking, navigation inside the MR imaging relied on real-time MR imaging, which is of a substantially lower spatial and temporal resolution than that possible with commercial EAM systems.
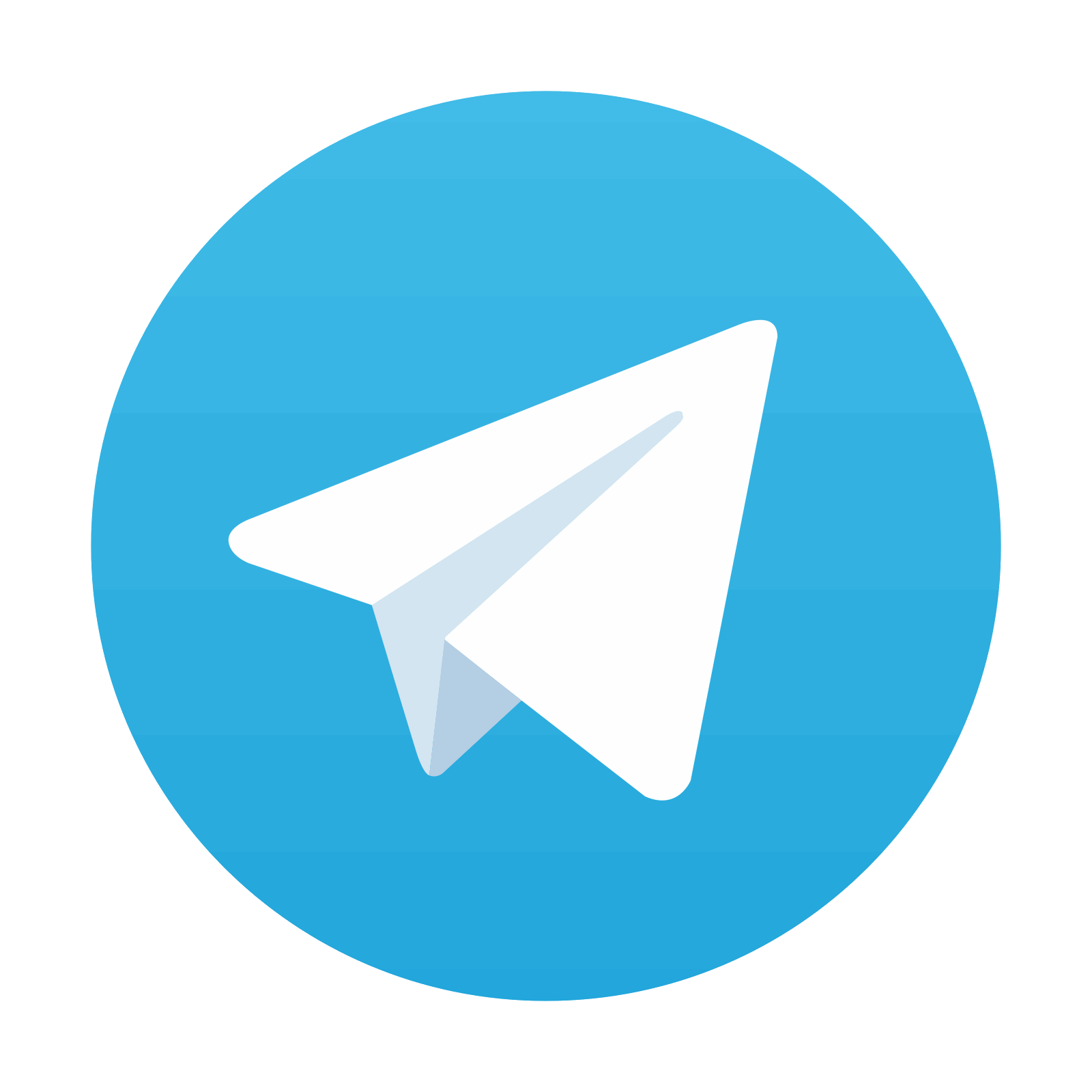
Stay updated, free articles. Join our Telegram channel

Full access? Get Clinical Tree
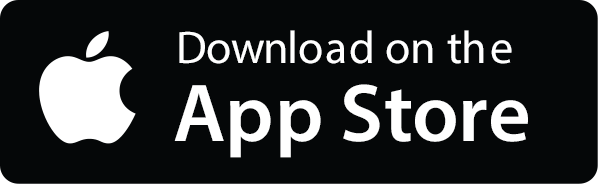
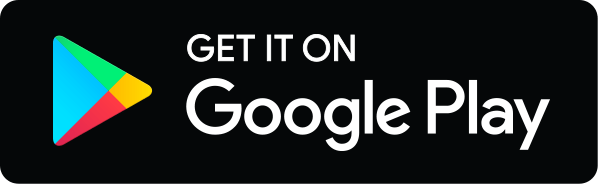
