Abstract
Fetal and placental magnetic resonance imaging (MRI) has grown from a tool for highly targeted examination in selected cases to a feasible and reproducible modality for a number of applications. However, MRI is not yet a standard screening tool and should be used only to answer specific questions raised by targeted ultrasound (US) or help refine prognosis in certain high-risk situations. Compared with US, MRI can provide important anatomic information; it is less limited by fetal lie, oligohydramnios, multiple pregnancy, or obesity and offers high-contrast images that can be acquired in true anatomic planes. MRI can also provide functional information, which may become a common tool for assessment of fetal cerebral or placental physiology. Importantly, the clinician performing and interpreting the MRI should have a clear understanding of previous US findings and of the relevant fetal anatomy and pathophysiology. This chapter summarizes the principles, benefits, limitations, and main indications for fetal or placental MRI.
Keywords
fetal MRI, magnetic resonance in pregnancy
Introduction
Assessment of fetal development and screening for congenital anomalies are essential elements of prenatal care. Ultrasound (US) imaging remains the first-line modality because of its convenience, low cost, and wide availability, but it can be limited by several factors, sometimes making US incompatible with women’s expectations, or subject to misdiagnosis-related legal issues. Compared to US, magnetic resonance imaging (MRI) is less limited by fetal lie, oligohydramnios, multiple pregnancy, or obesity. It offers high-contrast images that can be acquired in true anatomic planes. Over the past decade, in part because of shorter acquisition times that eliminate the need for fetal sedation, MRI has grown from a tool for highly targeted examination in select fetuses (primarily those who require brain evaluation) to a feasible and reproducible modality for a number of fetal applications.
MRI is not yet a standard screening tool, however, and should be used only to answer specific questions raised by targeted US, or help refine prognosis in certain high-risk situations. For instance, MRI may identify subtle signs of recurrence of an anomaly noted in a previous pregnancy, or when suspected fetal infection or ischemia may have caused fetal brain injury. MRI can also provide functional information, which may become a common tool for assessment of fetal cerebral or placental physiology. Importantly, the clinician performing and interpreting the MRI should have a clear understanding of previous US findings, and of the relevant fetal anatomy and pathophysiology.
Technique
Smith et al. reported the first use of MRI during pregnancy in 1983. Since then, techniques have evolved dramatically. Most fetal MRIs are performed at 1.5 tesla (T). Some recent studies have pointed out the potential benefits of stronger magnetic fields (3 T), but the advantages of additional signal intensity and resolution may be offset by increased artifacts because of fetal movement and by greater radiofrequency power deposition.
Developments in ultrafast acquisition have reduced fetal motion-based artifacts and, thus the need for fetal sedation. Fast (typically 20–30 s) T2-weighted acquisitions, as in single-shot fast spin echo (SSFSE) or h alf-Fourier a cquisition s ingle-shot t urbo-spin e cho- imaging (HASTE) (Siemens, Erlangen, Germany) and r apid a cquisition with r elaxation e nhancement (RARE; Brucker, Ettlingen, Germany), are the mainstay of fetal MRI. These sequences provide good resolution and perform well for surface delineation (because of the hypersignal of water in amniotic and cerebrospinal fluid [CSF]) and gyration analysis. Echo planar imaging with gradient-echo T2-weighted images (T2*) allows detection of chronic hemorrhagic lesions and calcifications, as hemosiderin deposits and calcifications lead to a loss of signal on sequences with a long echo time (TE).
T1-weighted sequences have lower spatial resolution and little inherent contrast, owing to the large water content. However, they may complement T2-weighed sequences by detecting lesions that the latter may overlook, such as laminar necrosis and calcified leukomalacia. In general, T1-weighted sequences are performed using gradient echo sequences and are useful for evaluating hemorrhage, the pituitary axis, and the thyroid, and the presence of meconium in the large bowel. They can also help to locate the liver in cases of diaphragmatic hernia.
Diffusion-weighted imaging (DWI) sequences, which can detect ischemic lesions at an early stage, may prove useful in high-risk settings. DWI is also useful for locating the kidneys, as functioning renal parenchyma gives a bright DWI signal. More generally, functional imaging sequences, especially for central nervous system indications, include diffusion tensor imaging with tractography, single-voxel spectroscopy for brain metabolism, and blood-oxygen-level dependent (BOLD) imaging. Functional imaging is also emerging for in vivo assessment of the placenta.
Timing of Examination
There are no contradictions to MRI in any trimester of pregnancy, although it is typically performed from 20–22 weeks’ gestation until birth because small fetal size and motion artifacts tend to compromise the exam before 18 weeks. Its intended role in guiding pregnancy management is key; if the purpose is to confirm ominous fetal findings, local laws regarding pregnancy termination may dictate timing. However, questions such as adequate cortical development or information about airway impingement at delivery can only be addressed late in pregnancy.
Fetal Anatomic Evaluation
Brain
Thanks to its superior soft-tissue contrast resolution, MRI is able to distinguish fetal brain structures such as cortical sulcation/gyration, posterior fossa structures, and CSF spaces. However, because brain development is a dynamic process, it is important to recognize the normal MRI aspect of the brain at different gestational ages to avoid false-positive or false-negative diagnoses. For instance, during the second trimester, cerebral malformations may result in morphologic features that differ from those seen in a more mature brain. Thus the sulcation pattern should always be analyzed ( Fig. 172.1 ) and measurements should be compared with published reference ranges. Importantly, MRI allows not only the skull but also the developing brain to be measured, thus avoiding errors caused by abnormal pericerebral spaces.
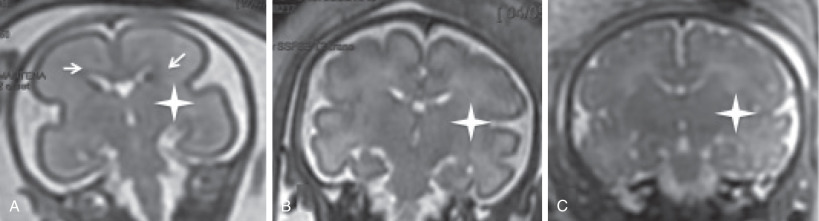
MRI can delineate fetal brain structures because it produces different signals for different structures. For instance, the high neuron density in the germinal matrix (a cell-dense layer immediately adjacent to the ventricular walls) produces a low signal on T2 and a higher signal on T1-weighted images. Fetal white matter, however, has the opposite signal characteristics (high T2 and lower T1) because of its higher water content and lack of myelination. Neurons from the germinal matrix migrate from the ventricular wall to the surface of the brain during the second trimester, producing a five-layered appearance of migrating cells between 20 and 28 weeks of gestation (see Fig. 172.1 ). Myelination mainly occurs after birth, but can be detected in the fetus by high signal intensity on T1-weighted images in certain structures.
Common indications for fetal brain MRI include (1) ventriculomegaly, (2) midline defects, such as agenesis of the corpus callosum, (3) posterior fossa anomalies, (4) cerebral cortical malformations, and (5) screening in a family with history of brain abnormalities such as tuberous sclerosis, corpus callosal dysgenesis, or lissencephaly. Although most of these pathologies are amenable to US diagnosis, MRI appears to have consistently higher reproducibility and sensitivity, and can often improve US diagnosis.
Cortex
MRI of the normal fetal cerebrum is characterized initially by a smooth surface that matures into cortical sulcations. The degree of sulcation on fetal MRI is an indicator of gestational-age–related cortical development. The main landmark is the Sylvian sulcus, which is typically developed by 28–30 weeks, and therefore exclusion of cortical malformations cannot be excluded until after this point in gestation. Disorders of cortical development should be suspected in the case of abnormal gyration mimicking the preterm appearance of the gyri. Cortical lesions can be defined by pathophysiologic processes, broadly subdivided into undermigration, overmigration, and organizational disorders. Undermigration leads to lissencephaly type 1 (pachygyri and shallow sulci) and gray-matter heterotopia (T2 hyposignal foci, underlying the ventricle wall). Heterotopias must be distinguished from subependymal nodules of tuberous sclerosis. Tubers appear hypointense on T2-weighted images and hyperintense on T1-weighted images. Subependymal giant astrocytoma is rare, typically occurring in the wall of the lateral ventricle near the foramen of Monro ( Fig. 172.2 ). Overmigration leads to lissencephaly type 2 (Walker-Warburg syndrome) with a typical Z-shaped brain stem. Polymicrogyria is caused by abnormal organization of neuronal cells, with excessive folding of the cerebral cortical surface, and numerous small and shallow gyri. Associated causes such as infection (commonly cytomegalovirus), genetic abnormalities, and ischemia should be suspected. The most commonly affected area is around the Sylvian fissure.
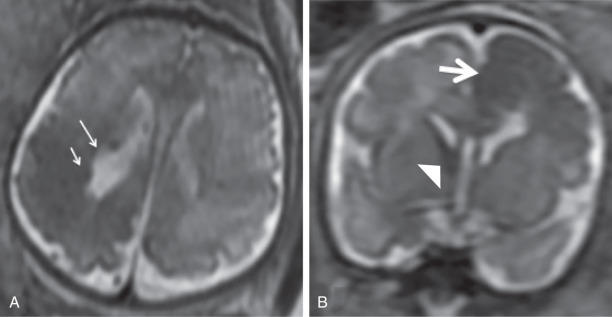
Ventricles
Like the cortex, the fetal ventricles change during gestation. Early, they appear prominent because of the relative paucity of brain parenchyma. Physiologically disproportionate enlargement of the occipital horns in relation to the frontal horns is apparent on fetal MRI until 23 weeks, after which the occipital horns gradually diminish. In the absence of ventriculomegaly, the atrial diameter of normal lateral ventricles remains constant (≤10 mm) from 15 to 35 weeks and the lateral borders are concave.
Ventriculomegaly on US is one of the main indications for fetal MRI, which is used to look for associated cerebral lesions. The shape of the ventricles can provide important clues: colpocephaly is associated with corpus callosum agenesis and pointed posterior horns with Chiari II malformation. Aqueductal stenosis is associated with widened posterior horns and third ventricle, and reduced extracerebral fluid. A potential cause of aqueduct obliteration is hemorrhagic intraventricular material, which can be detected with MRI (T1 hypersignal). Ventriculomegaly arising from ischemic or infectious events that cause cerebral atrophy can be associated with porencephaly, which may be seen with MRI.
Midline Structures
The septum pellucidum is a thin vertical membrane walled off by the corpus callosum above and the fornix below, separating the frontal horns of the lateral ventricles ( Fig. 172.3 ). The septum pellucidum is in close developmental association with the corpus callosum. During fetal development, the septum pellucidum is filled with CSF and is referred to as the cavum septi pellucidi. The corpus callosum appears on midline sagittal T2-weighted images as a C-shaped hypointense structure at the upper margin of the cavum septi pellucidi. It increases in length, width, and thickness with gestational age.
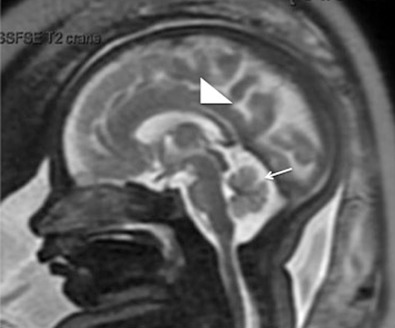
Agenesis/hypogenesis of the corpus callosum ( Fig. 172.4 ) ( Chapter 34 ) is one of the most common congenital brain malformations suspected upon prenatal US by the presence of a colpocephalic configuration of the occipital horns with parallel orientation of the lateral ventricles, and absence of the septum pellucidum. MRI should be used to rule out associated intracranial or extracranial anomalies, such as cortical malformations, parenchymal lesions, and brain-stem or cerebellar abnormalities. Lesions such as interhemispheric lipoma and interhemispheric cyst can coexist.
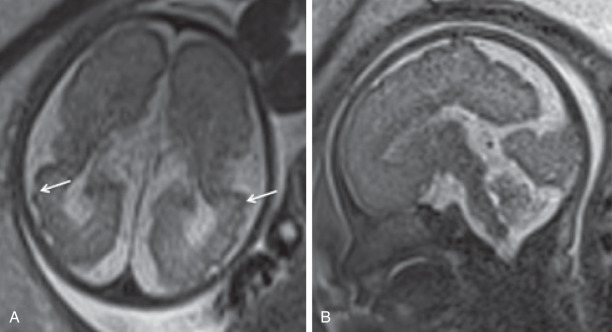
Posterior Fossa
The pontine flexure develops at the same time as the cerebellar hemispheres, and the midsagittal plane is essential to assess the primary flexure after 25 weeks. The transverse cerebellar diameter increases throughout gestation and can be measured on axial and coronal MRI. The vermis can be detected on midsagittal and axial plane MRI after 20 weeks of gestation. The dorsal part of the midbrain and pons has low signal intensity on T2-weighted images and high signal intensity on T1-weighted images after 24 weeks of gestation, because of myelination.
A common reason for MRI referral is an enlarged retrocerebellar fluid space (>10 mm) ( Chapter 37 ). MRI is better than US for the evaluation and multidimensional analysis of the cerebellum, cerebellar parenchyma, vermis, and brain stem. For instance, a significant reduction in the transverse cerebellar diameter is seen with cerebellar agenesis or hypoplasia, the cerebellum can be compressed in the case of an arachnoid cyst and the position of the tentorium cerebelli and the shape of the fourth ventricle can determine diagnosis of Dandy-Walker malformation ( Fig. 172.5 ). Other causes of enlarged CSF-filled spaces include Blake’s pouch cyst, defined as failure of regression of the rudimentary fourth ventricle. The retrocerebellar fluid space may be reduced in the case of Arnold-Chiari malformation, which should raise suspicions of myelomeningocele and prompt further MRI evaluation.
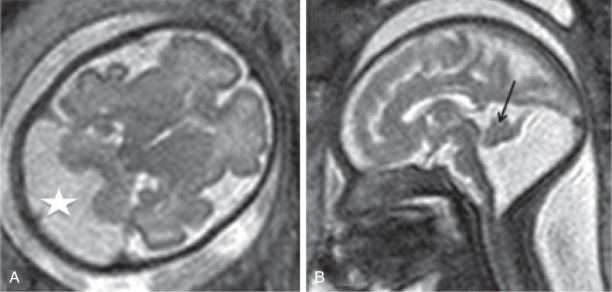
Acquired Brain Lesions
Causes of acquired fetal brain lesions include ischemic infarction, hemorrhage, tumor, or infection. The main intracranial signs of prenatal infection are ventriculomegaly, periventricular calcifications, microcephaly, polymicrogyria, and cerebellar hypoplasia. MRI can detect cortical lesions and hypersignal in the white matter, specifically the temporal lobe, and should be performed systematically in cases of proven fetal infection. Ischemic lesions can lead to a reduction in brain tissue, with porencephaly or schizencephaly. MRI can diagnose brain injury, including after laser therapy for twin-twin transfusion syndrome.
Hemorrhagic lesions can be detected by T1-weighted and echoplanar sequences. Hemorrhagic and parenchymal atrophy may be seen with the most common vascular lesion, vein of Galen malformation ( Chapter 42 ).
Thorax and Lungs
The most common lung lesions in the fetus are congenital diaphragmatic hernia (CDH), bronchopulmonary sequestration, congenital cystic adenomatoid malformation, bronchogenic cyst, congenital high airway obstruction syndrome, and bronchial plug. Because normal fetal lungs and airways are mainly filled with amniotic fluid, they have a relative hypersignal on T2-weighted sequences, allowing easy evaluation of lung volume and anatomy ( Fig. 172.6 ). MRI can distinguish cystic spaces from cysts and sequestration in the fetal lung, as well as identify vascular communication with the bronchial tree. The cystic spaces can be distinguished from cysts and sequestration as they communicate with the bronchial tree and are fed and drained by arteries and veins derived from the pulmonary circulation (in contrast with sequestration). Depending on the subtype, with variable-size cysts, their exact appearance can be better described with MRI. In the case of bronchial atresia or plug, segmental or subsegmental occlusion will lead to a dilated T2 hyperintense tubular structure that can be easily seen on MRI. MRI can evaluate pulmonary sequestration, which arises as an accessory pulmonary bud, composed of nonfunctioning lung tissue with no normal communication with the airways and an abnormal systemic arterial supply.
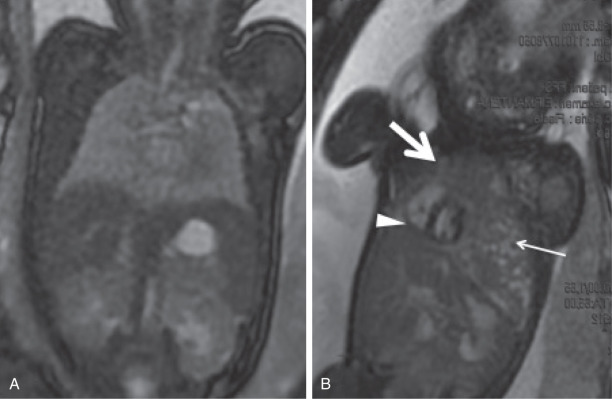
MRI has several advantages over US alone in the workup of fetal lung lesions: it can help to confirm a diagnosis, evaluate tracheal anatomy and lung lesion size, detect subtle accompanying anomalies, and improve the detection of chest anomalies. The biggest contribution of MRI is probably in CDH (see Fig. 172.6 ). Indeed, MRI can help to determine the position of the liver, assess the herniated content, detect commonly associated abnormalities, and evaluate the volume of the normal lung. Indeed, pulmonary volumes reflect the degree of lung hypoplasia and correlate directly with postnatal outcome. Pulmonary volume measurements must be standardized by gestational age, using the lung to head ratio and/or volumes reported to expected measurements. MRI may also reveal the presence of a sac around herniated organs, a finding associated with better outcome. Finally, MRI may prove useful in the evaluation of lung maturity, based on diffusion-weighted imaging and spectroscopy
Neck and Face
Neck masses are rare, and MRI is particularly helpful for detecting associated brain abnormalities, as well as assessing the extent of lesion to assist in delivery planning (e.g., need for ex utero intrapartum treatment).
Gastrointestinal and Genitourinary Tracts
MRI can improve prenatal diagnosis of esophageal atresia by demonstrating the pouch sign on dynamic sequences. It is also useful for detecting associated malformations, including tracheoesophageal fistula. Because of its large field of view and better tissue contrast than US, and because of the T1 hypersignal of meconium, MRI can assist in the evaluation of dilated bowel loops, and differentiation of proximal from distal obstruction ( Figs. 172.7 and 172.8 ).
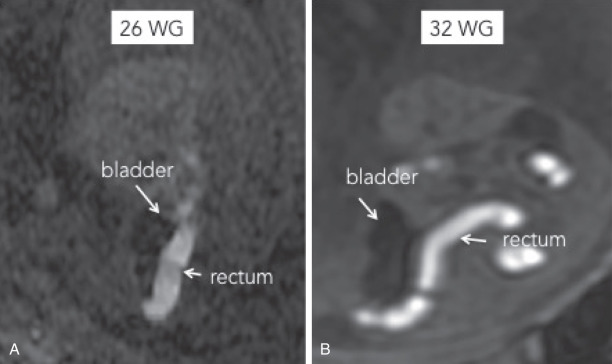
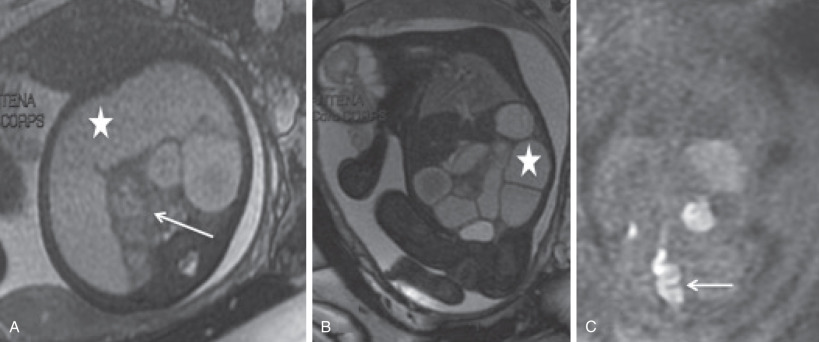
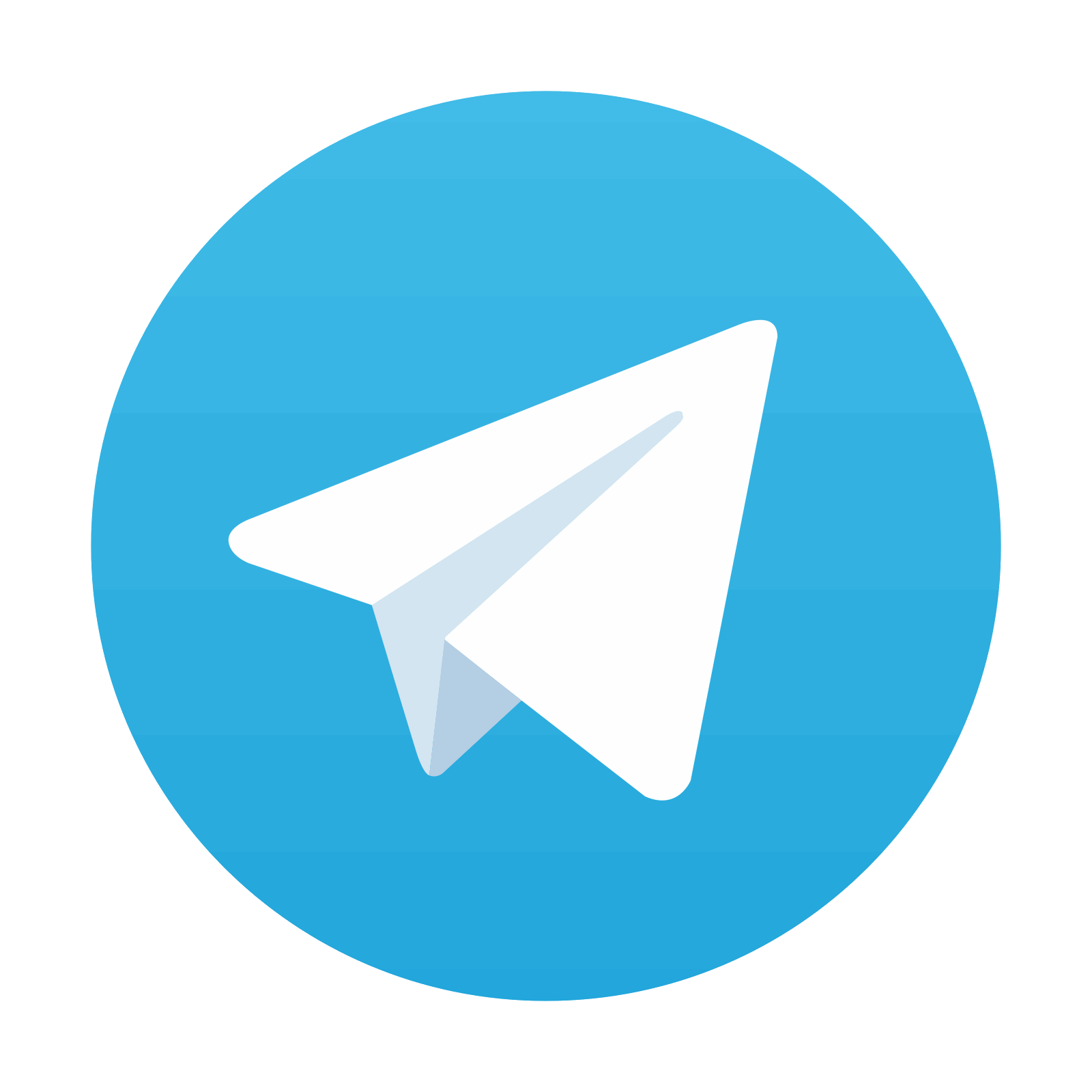
Stay updated, free articles. Join our Telegram channel

Full access? Get Clinical Tree
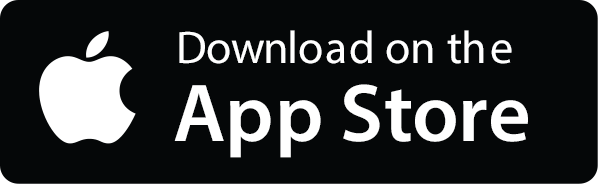
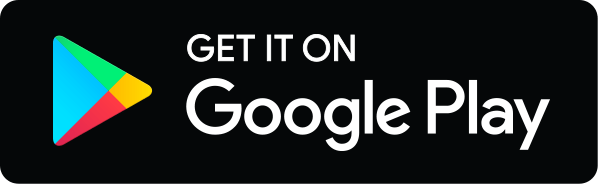
