KEY POINTS
Proliferation, neuronal migration, and organization occur relatively late in pregnancy and do not end until after delivery.
Malformations of cortical development (MCD) may be diagnosed during fetal life; the chances for diagnosis are better in severe cases and in those with associated anomalies.
MCD assay may in same cases diagnosed by using ultrasound. Characteristic features are shown in Figure 7–1.
Even when MCD is suspected, a definitive diagnosis is usually difficult during pregnancy.
In patients at risk, search systematically for signs of MCD. Check for the size of the lateral ventricles and the regularity of their walls; also, the presence of abnormal underdeveloped or overdeveloped sulci.
When a suspicion is raised, consult with a genetician and a pediatric neurologist. In low-risk patients, these signs may be the only possibility for prenatal diagnosis.
The processes of primary neurulation and ventral induction that result in the formation of the neural tube and in the formation of the prosencephalon, respectively, are completed by the second month of gestation.1 This is followed by three overlapping phases of cortical development, which are under the control of numerous genes: proliferation, migration, and organization. Stem cells at the surface of the ventricles proliferate and divide into glial cells and neuronal cells. The glial cells migrate to the cortex in a very regular radial pattern, leaving a radial scaffolding along which the neurons migrate (radial migration) to the surface. The later arriving neurons migrate in stages through the inner layers and ultimately lie outside them (inside out migration). Ultimately, six layers are formed. Once the neurons arrive at the cortex, they organize local connections. In addition to this radial migration, there is a tangential migration of neurons to form what are believed to be controlling tracts.2 The genes that control neuronal development also function in other parts of the body, so it is not unusual to find cerebral malformations associated with diverse somatic manifestations, such as skeletal dysplasia as present in thanatophoric dysplasia. This normal orderly developmental process can be disturbed by genetic, teratogenic, and environmental conditions. Because the cerebrum develops simultaneously with other structures, an insult at a specific time can affect the normal development of all the structures that are vulnerable at that time, including the eyes, face, and hindbrain. Hence the importance of assessing all of these areas if abnormality is suspected in any one of them. Over the past decade, the knowledge regarding the genetics, morphology, and clinical aspects of these conditions has expanded significantly, and new developments in this field have occurred rapidly.
Different classifications of malformations of cortical development (MCD) have been proposed3,4,5 (Table 7–1). Fundamentally, they are based on two factors: gene abnormality and timing of the first abnormal developmental event. Final phenotypic outcomes are often more dependent on the time that an insult occurs and interferes with normal development than its specific nature. Although Barkovich et al’s3 and Volpe’s5 classifications are particularly useful in clinical management, they are acknowledged to be in evolution and will change as new information becomes available. Sarnat’s4 classification is more centered on the genetic and embryologic mechanisms of the different diseases. The reader is referred to Chapter 2 for information on the normal sonographic appearance and development of the sulci and gyri.
Malformations due to abnormal neuronal proliferation |
Microcephaly Macrocephaly Hemimegalencephaly Tuberous sclerosis complex |
Malformations due to abnormal neuronal migration or organization |
Lissencephaly/subcortical band heterotopia spectrum Cobblestone complex syndromes Heterotopia Schizencephaly/polymicrogyria |
Micrencephaly
Microcephaly means small head. More specifically, it is intended to mean small brain (micrencephaly). In children and adults, microcephaly is defined as low brain weight and a small occipitofrontal head circumference (HC) >2 standard deviations (SDs) below the mean or below the third percentile (see Chapter 3). The implications of a diagnosis of fetal microcephaly may be grave. Measurements should be carefully obtained, and normograms used should take into consideration fetal gender, ethnic background, parental size, and family history. Diagnosis using –2 SDs as the lower limit will automatically categorize 2% of the population inappropriately as microcephalic. Such a broad definition obviously includes normal individuals. It is clear that there is an inverse relationship between the HC and the probability of associated mental retardation. Prenatally, there is no consensus regarding the exact definition of an abnormally small HC; some authors propose the –2 SD cutoff,3 whereas others propose the –3 SD cutoff.6,7 Using the –3 SD definition, Chervenak et al6 showed that prenatal HC measurement was sensitive for diagnosing microcephaly with no false-negatives; –4 SD was a specific test with no false-positive cases (see Chapter 3).
Seto et al8 noted in a population-based study performed in Japan a significant increase in the incidence of microcephaly as reported on birth forms from 0.37 per 10,000 births to 0.86 per 10,000 when comparing two consecutive 10-year periods between 1981 and 2000. Similar results (0.67 per 10,000 births) were found in a large Chinese study that recorded all the cases of microcephaly in newborns and stillborns of more than 28 weeks’ gestation diagnosed during the first 7 days of life.9 In a Canadian study,10 the incidence of microcephaly dropped from 0.3 per 10,000 live births to 0.1 per 10,000 when the diagnosis was made by HC measurements instead of relying only on the clinical impression. In 1999 in the United States, the nationwide rate of microcephaly was much higher and reached 5.9 per 10,000 live births;11 epidemiologic data from a single U.S. state showed a rate of 7 per 10,000 children under the age of 1 year.12 These differences are due to the fact that in the majority of cases, microcephaly is not present at birth but develops by the age of 1 year. In a study published in 1977, Sells13 found that 1.9% of the children attending regular classes in Seattle had an HC measurement <2 D of the mean for age and sex, but their IQs were not significantly different than those of the control group.
According to Rakic’s2,14 studies, cells in the ependymal layer of the lateral ventricle are the precursor of neuron and glial cells; during early proliferative stages, these progenitor cells start to divide symmetrically until the ventricular zone becomes highly cellular. A reduction in the total number of progenitor cells will cause a severe and lethal form of microcephaly known as radial microbrain. Decreased progenitor stem cell divisions result in microcephaly vera and reduced neuron numbers. In these cases, the weight of the brain is reduced, but macroscopically, it will appear almost normal.5
Microcephaly can result from many different processes, including chromosomal abnormalities, single gene defects, infections, and environmental effects, all of which can impair neuronal proliferation (Table 7–2). Microcephaly may be present as an isolated finding or it may be part of a more complex condition. Only some of these conditions may be apparent at prenatal examination. A search in the Online Mendelian Inheritance in Man (OMIM) database found 548 entries for microcephaly, but only some of these conditions are apparent in utero or in the neonatal period. When isolated, it is termed primary microcephaly. Autosomal recessive inheritance is described with gene mutations involving MCPH, ASPM, CDK5RAP, or CENPJ in some mild cases, and ALM, ARFGEF2, or RAB3GAP in more severe cases.3
Earliest Reported Diagnosis | Methods of Diagnosis | Associated Anomalies | |
---|---|---|---|
Syndromes | |||
Cerebrooculofacioskeletal syndrome (AR)188 | Second trimester | US, DNA repair analysis | Microphthalmia, CNS Skeletal |
Cockayne syndrome (AR)189 | First trimester | DNA analysis | IUGR, brain calcifications, cataracts, skeletal |
Cornelia de Lange syndrome (AD, S)190 | First trimester | US, mutation analysis | IUGR, GUT, CHD, facial, skeletal |
Meckel-Gruber syndrome (AR)191 | First trimester | US | Encephalocele, polycystic kidneys, polydactyly |
Mowat-Wilson syndrome (AD)195 | Birth | US, MRI | ACC, CHD, facial, megacolon, hypospadias. |
Neu-Laxova syndrome (AR)193 | Second trimester | US | IUGR, CNS, skeletal, facial |
Warburg micro syndrome (AR)194 | Birth | Clinical presentation | ACC, microphthalmia, cataracts |
Migration disorders with associated microcephaly | |||
Galloway-Mowat syndrome (AR)195 | Second trimester | US, MRI | IUGR, facial, MCD |
Miller-Dieker lissencephaly syndrome (AD)120,196 | Late second trimester | FISH, US, MRI | Lissencephaly, ventriculomegaly, MR |
Norman-Roberts syndrome (AR)197 | Second trimester | US, MRI | IUGR, facial, lissencephaly |
Metabolic diseases | |||
3-hydroxyisobutyric aciduria (AR)198 | Second trimester Third trimester | 3-OHB elevated in AF US, MRI | MCD, brain calcifications, facial |
Maternal phenylketonuria embryopathy199 | Second trimester | US | IUGR, CHD, MR |
Recessive hereditary methemoglobinemia type II (AR)200 | Birth | Clinical presentation, low cytb5r activity | IUGR |
Smith-Lemli-Opitz syndrome (AR)201 | Second trimester | Low E3, elevated 7-dehydrocholesterol, mutation analysis, US | IUGR, CNS, facial, CHD, renal, micropenis, polydactyly |
Skeletal dysplasias | |||
Microcephalic osteodysplastic primordial dwarfism type I (AR)202,203 | Second trimester | US | IUGR, CNS, skeletal, CHD, facial, micropenis |
Juberg-Hayward syndrome (AR)204 | Second trimester | US | IUGR, CNS, facial, skeletal |
Seckel syndrome (AR)205 | Second trimester | US, MRI | IUGR, skeletal, facial |
Chromosomal disorders | |||
Trisomy 2171 | First trimester | US, karyotype | Increased NT, CHD |
Trisomy 135 | First trimester | US, karyotype | CNS, facial, CHD |
Trisomy 185 | First trimester | US, karyotype | CNS, facial, CHD, skeletal |
5p deletion (cri du chat)206 | Second trimester | US, karyotype | IUGR, facial |
Mosaic variegated mutation syndrome (AR)207 | 22 weeks | US, karyotype | IUGR, ACC, CH, DWM, facial |
Nijmegen breakage syndrome (AR)208 | First trimester | DNA analysis | |
Triploidy209 | First trimester | Karyotype, US | |
Wolf-Hirschhorn syndrome210 | Second trimester | FISH, US, MRI | IUGR, PVPC, facial, CHD, MRI |
The whole picture regarding the genetics of microcephaly is far from complete, and new genes have been described, some of them transmitted as an X-linked trait as MRXS9 on chromosome Xq12-q21.3115 or as an autosomal dominant trait with incomplete penetrance due to microdeletions on chromosome 1q21.116 or microduplications on chromosome 9q22.32.17 It is likely that current microarray-based comparative genome hybridization (CGH) techniques will demonstrate additional gene abnormalities that are currently undetectable using conventional cytogenetic methods.
The information available in the literature regarding the pathology of microcephaly is scant. Reported cases consistently describe reduced brain weight with sulcal patterns ranging from normal to patterns described as “simplified gyral pattern” or microlissencephaly. The actual microscopic structure of the cortex in these cases is not clear. An example of the cerebral histology in a fetus at 26 postmenstrual weeks with microcephaly vera published by Evrard et al18 showed a depleted germinal layer, white matter without migrating neurons, and abnormal superficial cortical layers. Garel described a fetus with microlissencephaly at 26 postmenstrual weeks showing normal myelination, cortical thickness, and neuronal differentiation with a very small number of cortical neurons and simplified gyration.19
The presence of associated anomalies and their identification using imaging or pathologic description is paramount in reaching a definitive diagnosis. The anomalies present in microcephalic individuals may be classified into three groups: associated anomalies directly related to the small brain (sloping forehead, apparent large ear size, and increased amount of extra-axial cerebrospinal fluid [CSF]) (Figures 7–2 and 7–3) or to abnormal cortical migration (microlissencephaly, periventricular heterotopia, and dysgenesis of the corpus callosum) (Figure 7–4), other brain anomalies (cerebellar or brainstem dysgenesis), and non–central nervous system (CNS) anomalies. Non-CNS abnormalities may involve any body system.
Figure 7–4.
A fetus with microcephaly, lissencephaly, and dysgenesis of the corpus callosum at 33 weeks, 4 days. (A) Midsagittal plane shows a thin corpus callosum of irregular thickness (arrows) with a large cavum septi pellucidi (CSP) and cavum verga (CV); the vermis is not clearly defined (arrowheads). (B) Parasagittal plane at the level of the insula shows complete lack of sulcation. (C) Coronal plane at the level of the frontal lobes showing similar findings. Note the wide interhemispheric fissure with increased amount of cerebrospinal fluid (CSF) between the lobes (arrows). (D) In a more posterior coronal plane, the CSP is abnormally large.
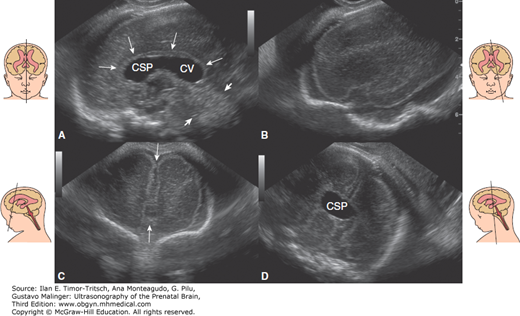
Genetic counseling for families with known single-gene defects is straightforward. The genetic counseling recommendations for primary microcephaly are not yet clearly established. Based on the growing number of autosomal recessive microcephaly loci being reported, Dobyns recommends giving a 25% recurrence risk to parents of any child with microcephaly with simplified gyral pattern or primary microcephaly.7 He believes that the lower risks suggested by older studies is probably due to inclusion of children with nonspecific mild microcephaly.
The recurrence risk may be as high as 50% when one of the parents is microcephalic with normal intelligence,20,21 in these cases, the child will have normal or near-normal intelligence.
The diagnosis of fetal microcephaly is mainly based on biometric measurements. An accurate diagnosis can be difficult, especially in borderline cases due to various factors, including gestational age uncertainties; lack of appropriate HC charts specific for gender, ethnic background, and fetal weight; influence of parental head circumference; and late development of microcephaly during the third trimester or postnatal period.22,23,24,25
Generally, suspicion should be aroused by any head size that is significantly smaller than expected for dates or in comparison to other body parts. Suspected cases should undergo detailed evaluation of the brain and all other body systems. Most significant cases will have additional findings beyond small head measurements, and these can help to confirm the diagnosis. Often intracranial brain structures are very difficult to see because the cranial sutures are very narrow (Figure 7–5). This limited visibility itself is a clue to failure of brain growth because skull growth depends on brain growth.
Chervenak et al6 published in 1984 a paper on the diagnosis of fetal microcephaly. More than 25 years later, it continues to be the main reference for calculating abnormal HC size and counseling. According to their data, microcephaly was diagnosed when the HC is found to be <–3 SD. Using this cutoff, they had no false-negative diagnoses; an HC <–4 SD was a specific cutoff without false-positive patients. An HC/abdominal circumference ratio <3 SD and a femur length/head circumference ratio >3 SD were also helpful in the diagnosis26 (see Chapter 3).
Other measurements have been proposed in an effort to improve diagnostic accuracy,27,28 but these have not been independently verified.23
Patients with extreme microcephaly have been diagnosed as early as 15 postmenstrual weeks of pregnancy, but this is the exception.29 These patients usually have multiple malformations, and the diagnosis is straightforward (Figure 7–6). It is of paramount importance to remember that prenatal diagnosis consistently fails to diagnose most cases of primary microcephaly mainly because head growth is normal until late pregnancy. In a study of 9600 low-risk pregnancies evaluated using axial planes that included HC measurements, Reece and Goldstein30 failed to diagnose all 5 cases with microcephaly. Bromely and Benacerraf31 found that 6 out of 7 microcephalic children had a normal HC before 22 postmenstrual weeks of pregnancy, and microcephaly was detected between 27 and 33 postmenstrual weeks of pregnancy. Even in high-risk cases with recurrence risk of 25% to 50%, the likelihood of reaching a correct diagnosis during the last weeks of pregnancy may be impossible.23,32
Figure 7–6.
Discordant twin pregnancy at 18 postmenstrual weeks. Twin A, female with microcephaly, occipital encephalocele, ventriculomegaly, and facial dysmorphism; twin B, normal male (not shown). (A) Lemon-shaped calvarium with bilateral ventriculomegaly. Biparietal diameter (BPD) = 30 mm, well below the fifth percentile, HC = 117 mm, between –2 and –3 SD. (B) Ventriculomegaly with abnormal brain parenchyma and indentation of the ventricular wall (arrow). (C) Fetal profile shows an abnormal nose with mild micrognathia. (D, E) Occipital encephalocele (arrow).
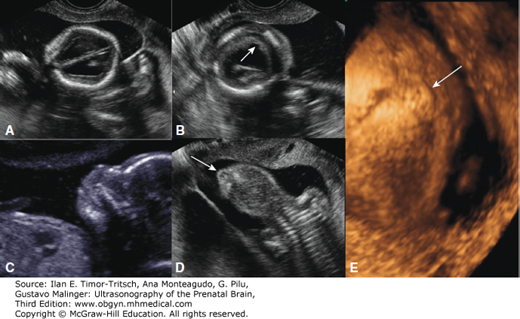
Pilu and colleagues33 reported on the prenatal diagnosis of microcephaly in two fetuses assisted by transvaginal sonography (TVS) and color Doppler. In these two cases, TVS revealed aberrant findings, including large subarachnoid spaces and a rudimentary shape of the lateral ventricles. In one of these fetuses, a sloping forehead was present; and in the other, power Doppler ultrasound (US) demonstrated a discrepancy in the size of the signals generated by the intracranial arteries branching from the internal carotid arteries and those branching from the vertebral arteries. This was interpreted as the consequence of a reduced blood supply to the undersized cerebral hemispheres.33 The authors suggested that evaluation of intracranial anatomy by TVS and power Doppler examination of the cerebral vessels may be of value in the diagnosis of fetal microcephaly. In fetuses with a small HC, the presence of an increased amount of CSF surrounding the brain, particularly when this fluid is prominent around the frontal horns and in the interhemispheric fissure, can be an indicator of congenital microcephaly (see Figure 7–3).
The accuracy of US in the diagnosis of fetal microcephaly has not been studied prospectively, but two retrospective analyses have been published. In the first study, den Hollander et al34 reported on 30 fetuses referred at a mean gestational age of 27 postmenstrual weeks due to reduced head size or suspected intrauterine growth retardation (IUGR) or intra- or extracranial anomalies. Associated anomalies were present in 83.3% of the patients: holoprosencephaly (16.7%), chromosomal anomalies (23.3%), genetic syndromes (20%), and multiple anomalies (23.3%). Only five patients were considered as representing “isolated microcephaly,” but a careful analysis of these cases showed that three of them had other anomalies, and only two represented patients with autosomal recessive primary microcephaly. The authors did not describe the number of fetuses with microcephaly diagnosed after delivery in their center.34
Dahlgren and Wilson35 reviewed all cases of microcephaly diagnosed during a 10-year period at British Columbia Women’s Hospital. They found 45 cases; in 21, the diagnosis was made prenatally and confirmed postnatally. In 15 patients, the second-trimester US was available, and 12 of these patients had a normal scan between 15 and 20 postmenstrual weeks of gestation. In nine patients (43%), the etiology of microcephaly remained unclear: possible viral infection based on placental signs of villitis or chorioamnionitis (four), multiple malformations (one), constitutional (one), and no specific etiology identified (one).
Our diagnostic approach to patients with suspected microcephaly is presented in Figure 7–7.
Magnetic resonance imaging (MRI) can be very helpful and may add information regarding associated malformations and subtle differences in the gyration pattern that may be difficult to visualize by US. The characteristic receding forehead, increased amount of extra-axial fluid, and presence of a simplified gyral pattern have been reported using MRI19,36 (Figure 7–8). It is important to remember that in all these patients, the MRI was performed following the measurement of an HC <–3 SD.
Figure 7–8.
T2-weighted magnetic resonance imaging (MRI) at 31 postmenstrual weeks in a fetus with microcephaly and simplified cortical pattern. Axial (A), coronal (B), and sagittal (C) planes show sloping forehead and increased extra-axial fluid with abnormal, simplified sulcation pattern. (Courtesy of Dr. Atil Yuksel and Dr. Arda Lembet, Istanbul, Turkey.)
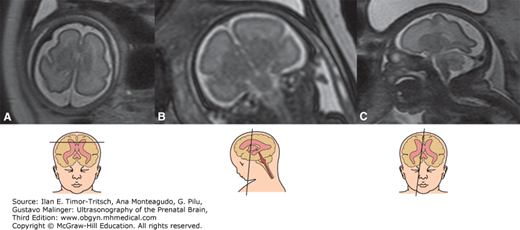
Frustratingly, most children suffering from primary microcephaly will have normal HC when examined during the second trimester and even later on in pregnancy or at birth. The only chance to reach a diagnosis in at least some of the patients will be to perform follow-up examination of those fetuses with an HC in the low-normal range, but we doubt that such an approach is warranted. Another possibility should be rescreening at 32 to 34 postmenstrual weeks. Fetuses with syndromic microcephaly may be detected during routine second-trimester US based on the presence of associated anomalies.
In families at risk of microcephaly following the diagnosis of the disease in a sibling or when one of the parents has microcephaly, it is important to obtain exact dating using first-trimester US measurements. An early second-trimester anatomical US examination performed between 14 and 16 postmenstrual weeks of gestation may be used to rule out the presence of associated anomalies. Fetal measurements should be performed at 4-week intervals until term to evaluate the HC growth curve. A detailed neurosonogram or MRI is indicated at around 32 postmenstrual weeks if the HC is small.
The use of gender-specific charts37 may be helpful in improving diagnostic accuracy.
The fate of fetuses with an HC between –2 SD and –3 SD during pregnancy is still not clear. In a recent study,38 we found that when excluding fetuses with associated malformations, there were no significant differences in neurodevelopmental performance at the ages of 2 to 4 years between children that had small HC (between –2 SD and –3 SD) in the prenatal period and controls. Studies have shown that children with an HC between –2 SD and –3 SD are more likely to have learning disabilities with near-normal intelligence.38
Arvey et al39 found that in children with microcephaly, diagnosed during the first year of life, the risk of moderate to severe mental retardation increased from 33% to 62% when comparing children with a HC between –2 SD and –3 SD and those with an HC <–3 SD.
Outcomes in those syndromes and associated anomalies can depend on the associated abnormalities.
Syndromic and autosomal recessive primary microcephalies are conditions associated with a high risk of moderate to severe mental retardation, and termination of pregnancy, when legally possible, should be offered. Milder cases will continue to be very difficult to manage.
Careful examination of the parents and family history is important because some normal families have members with small-appearing heads.
Macrocrania. Following exclusion of ventriculomegaly and enlarged subarachnoidal spaces, megalencephaly, megacephaly, megalocephaly may be used as synonyms.
Macrocephaly is defined in children and adults as increased brain weight and an HC >2 SD above the mean or above the 98th percentile. The diagnosis excludes head enlargement that is secondary due to other causes, such as hydrocephaly, subdural hematoma, and tumors. As in microcephaly, the diagnosis of fetal macrocephaly may carry a grave prognosis, but the possibility of a false-positive diagnosis should be considered. Common pitfalls may be due to measurement errors and lack of normograms based on fetal gender, ethnic background, and parental HC. It should be remembered that using +2 SD as the upper limit automatically categorizes 2% of the population as macrocephalic. There is no consensus during fetal life regarding the exact definition of an abnormally large HC as there is no available literature on this issue.32 The U.S. Centers for Disease Control and Prevention (CDC) growth charts for HC have recently defined the 97th percentile at birth as 39 cm for boys and 38 cm for girls (http://www.cdc.gov/growthcharts/). These charts are not concordant with the fetal charts.
Statistical data regarding the prevalence or incidence of macrocephaly at birth in the general population are scant. In a study performed in Sweden only in boys, it was found that the prevalence was 1 in 198.40 In autopsy series, the rates of macrocephaly range from 1 in 1146 to 1 in 50,000.41 Macrocephaly is more prevalent in selected populations, such as children with learning disorders,42 developmental disabilities,43 or autism.
Macrocephaly is considered a defect of cell proliferation due to either a more rapid or prolonged time of cell replication or a reduced rate of cell apoptosis.5 Although the total number of neurons is increased, the cells are morphologically normal.
In a mouse model, postnatal progressive megalencephaly has recently been linked to potassium channel dysfunction. The authors propose that a potassium ion channelopathy may be the cause of idiopathic megalencephaly and early-onset epilepsy in a group of infants or young children with or without cognitive impairments.44
Macrocephaly may be isolated or part of a syndrome. There is an isolated form known as familial benign macrocephaly which is due to enlargement of the subarachnoid space and may be transmitted as a dominant or recessive trait or be sporadic.
Macrocephaly associated with other malformations is usually due to specific syndromes, some of which may be diagnosed prenatally45–69 (Table 7–3). Others, such as Weaver, Sturge-Weber, Alexander, and fragile X, may not manifest macrocephaly during fetal life.
Earliest Reported Diagnosis | Method | References | |
---|---|---|---|
Syndromes | |||
PTEN-related syndromes | Birth | Mutation analysis | Tekin et al45 |
Neurofibromatosis type 1 | 27 weeks | US, MRI | McEwing et al46 |
Linear epidermal nevus syndrome | 30 weeks | US | Neis et al 47 |
Hemimegalencephaly | 25 weeks 32 weeks | US MRI | Malinger et al69 Agid et al48 |
Frontal macrocephaly, polymicrogyria | 21 weeks | US, MRI | Parazzini et al49 |
Macrocephaly with thick corpus callosum | 23 weeks | US, MRI | Lerman-Sagie et al68 |
Overgrowth syndromes | |||
Macrocephaly, capillary malformation syndrome | 31 weeks | MRI, US | Gripp et al50, Nyberg et al51 |
Sotos syndrome | 31 weeks | US | Thomas et al52, Chen et al53 |
Simpson-Golabi-Behemel syndrome | Birth (34 weeks) | Clinical presentation | Yamashita et al54, Hughes-Benzite et al55 |
Perlman syndrome | Birth | Clinical presentation | Schilke et al56, Alessandri et al57 |
Megalencephaly, polymicrogyria, and hydrocephaly (MPPH) syndrome | 31 weeks | MRI, US | Gripp et al50 |
Neuro cardio facio cutaneous syndromes | |||
Costello syndrome | 27 weeks | US | Lin et al58 |
Metabolic diseases | |||
Glutaric aciduria, type 1 (GA-1) | 33 weeks | US, MRI | Mellerio et al59 |
D-2-hydroxyglutaric aciduria | 40 weeks | US, Mutation analysis | Zafeiriou et al60 Augoustides-Savvopoulou et al67 |
Canavan disease | Birth | Clinical presentation | Traeger et al61 |
Megalencephalic leukodystrophy with cysts (MLWC) | First trimester | Mutation analysis | Shukla et al66 |
Skeletal dysplasias | |||
Achondroplasia | 27 weeks | US | Huggins et al62 |
Thanatophoric dysplasia | 15 weeks 27 weeks | US US | See Figure 7–9 Chen et al63 |
Campomelic acampomelic dysplasia | Birth (33 weeks) | Clinical presentation | Michel-Calemard et al64 |
Greig cephalosyndactyly syndrome | Birth | Clinical presentation | Sobetzko et al65 |
Chromosomal disorders | |||
46XX del [3q]26.1-27.1 | 20 weeks | US | See Figure 7–10 |
There are no available data regarding histopathology of familial benign isolated macrocephaly.
The diagnosis of associated anomalies enables differentiation between syndromic and nonsyndromic cases, and a detailed search should be performed when macrocephaly develops in utero. Family history is important because about half the cases will be benign familial macrocephaly. The search for associated anomalies should be initially oriented toward differentiation between fetuses with abnormal growth (overgrowth syndromes or skeletal dysplasias) and those who only have a large HC (Figure 7–9). As with microcephaly, macrocephalic individuals display craniofacial anomalies that may be directly related to the large brain (frontal bossing and increased amount of extra-axial fluid) (Figures 7–9 and 7–10) or associated with MCD (pachygyria, polymicrogyria, periventricular heterotopia, and dysgenesis of the corpus callosum) (Figures 7–9, 7–10, and 7–11), other brain anomalies (ventriculomegaly and cerebellar or brainstem dysgenesis) (Figure 7–10), or non-CNS anomalies (Figure 7–9).
Figure 7–10.
Macrocephaly (HC = +2 SD) in a fetus at 20 postmenstrual weeks with chromosome 3q deletion. (A) Median section of the brain shows dysgenesis of the corpus callosum (arrows). (B) Paramedian section shows ventriculomegaly; note the irregular ventricular wall (arrow). (C, D) Three-dimensional (3D) imaging of the fetal profile shows frontal bossing (C) and wide open anterior fontanelle (D).
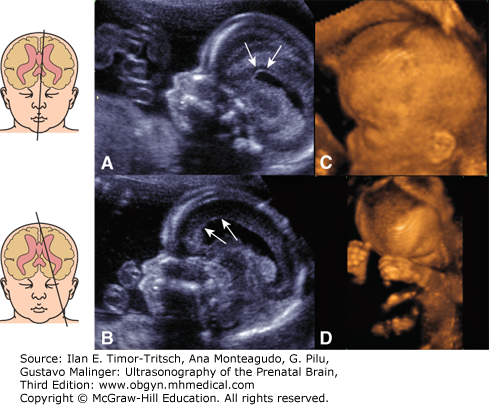
When searching for non-CNS anomalies, particular attention should be given to the extremities (polydactyly and vascular malformations)50 (Figure 7–11).
Figure 7–11.
Macrocephaly/vascular malformations syndrome. Prenatal at 32 weeks of gestation (A) and postnatal at 2 months of age (B) axial T2-weighted MRI show an abnormally open operculum lined with an abnormal cortex (polymicrogyria) (arrows). (C) Ultrasound (US) at 33 postmenstrual weeks shows syndactyly of foot fingers 5 and 6 (arrows). (D) Fetal MRI shows a hemangioma of the elbow (arrow).
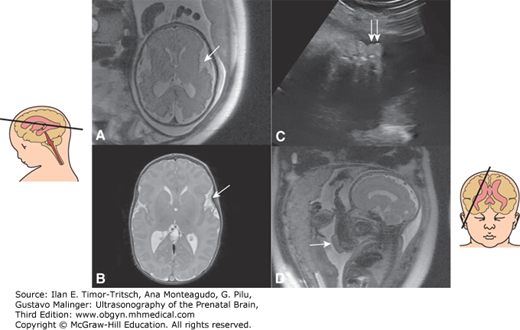
Genetic counseling for families with known single-gene defects is straightforward. Familial isolated macrocephaly may have a recurrence risk of up to 50%, but this is usually a relatively benign condition. According to Arbour et al,70 the inheritance pattern of nonsyndromic macrocephaly is considerably lower than expected for an autosomal dominant trait and should be considered multifactorial and not dominant. Similar findings were found in the Swedish study previously mentioned.40
Due to its development in late pregnancy or after birth, macrocephaly is usually not diagnosed during pregnancy, and when suspected during the third trimester, biometric and anatomical evaluation are usually difficult. Accurate diagnosis can be limited due to gestational age uncertainties, lack of appropriate gender-specific fetal HC charts, ethnic background, weight, and influence of parental HC.
According to our experience, the vast majority of fetuses with apparently isolated macrocephaly with HC between 2.0 and 2.5 SD are males and most of them have a normal HC at birth.
Exceptionally, macrocephaly may be diagnosed during the second trimester. These patients are at increased risk of having one of the syndromic conditions (see Figures 7–9 and 7–10; Table 7–3). The prenatal diagnosis of familial isolated benign macrocephaly may be made when there is a family history and no associated malformations71,72 (Figure 7–12).
Figure 7–12.
Prenatal US (A, B) and MRI (C, D) at 32 weeks’ gestation and postnatal MRI (E) in a patient with apparently isolated macrocephaly showing subarachnoid enlarged spaces. A subdural hematoma was suspected by neurosonography due to the presence of floating echogenic material (arrows in A and B). MRI shows asymmetric amounts of CSF overlying the right hemisphere with residual dural thickening (arrow). The father is also macrocephalic; the child is developing normally at the age of 3 years. The subdural hematoma resolved shortly after birth.
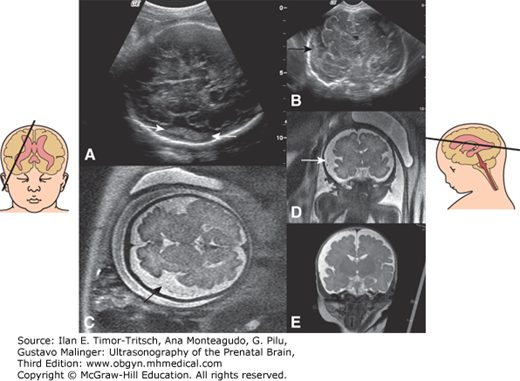
Our diagnostic approach to patients with suspected macrocephaly is presented in Figure 7–13.
MRI may add information regarding associated malformations and subtle differences in the gyration pattern that may be difficult to visualize by US. The characteristic frontal bossing, increased amount of extra-axial fluid, and presence of abnormal sulcation have been reported using MRI19,36 (Figure 7–11).When evaluating patients with suspected macrocephaly, caution must be taken because increased extra-axial fluid may give a false impression of an abnormal oversulcation pattern (see Figure 7–12).
Most of the children with isolated macrocephaly will have an age-appropriate HC when examined during the second trimester and even in later pregnancy or at birth. The only chance to reach a diagnosis in at least some of the patients will be to perform follow-up examination of fetuses with an HC in the high-normal range, but we doubt that such an approach is warranted.
Syndromic macrocephaly along with associated anomalies may present during routine second-trimester US.
In families at risk of having syndromic macrocephaly, it is of paramount importance to establish accurate dating in the first trimester. An early second-trimester anatomical US examination performed between 14 and 16 postmenstrual weeks may be performed to rule out the presence of associated anomalies. Fetal measurements can be performed at 4-week intervals until term to evaluate the HC growth curve. A detailed neurosonogram or MRI may provide additional information if the HC is large.
The fate of fetuses with an HC between 2 and 3 SD during pregnancy is not clear. In a recent study we found that when excluding fetuses with associated malformations, there were no significant differences in the neurodevelopmental performance at the ages of 2 to 4 years between children with a large HC (between 2 and 3 SD) in the prenatal period and controls.38
A study in boys has shown that those with an HC above 2 SD have a significantly lower intelligence level (odds ratio, 1.32; 95% confidence interval [CI], 1.11–1.38), but not mental retardation (odds ratio, 1.31; 95% CI, 0.80–2.02).40 Others found that the risk of mental retardation in children without apparent associated malformations was as high as 7% to 10%.73,74
Syndromic macrocephalies are conditions associated with a high risk of moderate to severe mental retardation, and termination of pregnancy, when legally possible, should be offered. Isolated macrocephaly, particularly in patients with a family history and when the HC measurement is close to 2 SD, has a good prognosis.
Hemimegalencephaly describes abnormal hamartomatous enlargement of one hemisphere. It is regarded as an abnormality of neuronal proliferation and migration and may be due to abnormal function of left-right organizer genes. Most cases are sporadic with unknown etiology.
Isolated and syndromic forms are described. A group of syndromes known as neurocutaneous syndromes are frequently associated with hemimegalencephaly. Neurocutaneous syndromes include epidermal nevus syndrome, Proteus syndrome, Kippel-Tréaunay-Weber syndrome, neurofibromatosis type 1, and tuberous sclerosis complex, among others. The cerebral findings are similar in isolated and syndromic cases.75,76 The clinical manifestations vary with the degree of brain abnormality and can include epilepsy, psychomotor retardation, and contralateral hemiparesis. Hemispherectomy may be needed for seizure control.75
It has been detected as early as 18 weeks gestation.77 At prenatal US the affected hemisphere shows increased volume, ventricular enlargement, abnormal texture, and occasional calcifications (Figure 7–14). The smaller hemisphere is compressed and distorted by the enlarged one, and characteristically abnormal findings are also present.78 Note that normal fetuses can have a minimal 2 mm asymmetry at 20 to 22 postmenstrual weeks.79
Figure 7–14.
Hemimegalencephaly diagnosed by US at 28 postmenstrual weeks of gestation (A–C) with MRI confirmation at 29 weeks of gestation (D–F) and following delivery (G–I). The clinical course was complicated by refractory epilepsy. Note the difference in the size of the cerebral hemispheres and lateral ventricles. The corpus callosum is thick (C, I), and the vermis not observed in the midline (E, I). Abnormal sulcation in the left hemisphere is suggested on fetal images and clearly depicted in both hemispheres on postnatal MRI (G, H). (Courtesy of Dr. Mauricio Herrera, Bogotá, Colombia.)
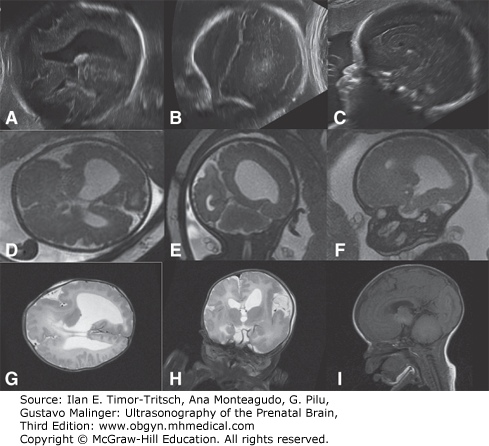
Prenatal US findings can be confirmed by MRI (Figure 7–14). Differential diagnosis includes intracranial tumor or bleed and infections.
Prenatally diagnosed cases are offered pregnancy termination.
Tuberous sclerosis, TSC, Bourneville disease, epiloia, phakomatosis TS
Tuberous sclerosis complex (TSC) is a neurocutaneous syndrome characterized by abnormal proliferation of neurons and glia in the form of hamartomata or low-grade tumors with accompanying anomalies of migration and differentiation. The manifestations of TSC are not limited to the brain but usually involve other systems, particularly the skin, eyes, kidneys, lungs, heart, blood vessels, and bones. In the fetus, the presence of cardiac rhabdoyomata is highly suggestive of TSC (Figure 7–15).
The prevalence is estimated to be between 7 and 12 cases per 100,000 live births, with more than half of these cases undetected prenatally.80 The total population prevalence figures have steadily increased from 1 in 150,000 in 1956, to 1 in 100,000 in 1968, to 1 in 70,000 in 1971, to 1 in 34,200 in 1984, to the present figure of 1 in 12,500 in 1998.
TSC is caused by mutations of the TSC1 gene on chromosome 981 or of the TSC2 gene on chromosome 16.82 This autosomal dominant disease expresses the following two successive mutations: A germ line mutation produces a loss of heterozygocity in one of the alleles, followed by a somatic mutation of the other allele.83 TSC1 and TSC2 encode for two proteins called hamartin and tuberin, respectively. Hamartin and tuberin are tumor suppressors acting as inhibitors in the mTOR cascade pathway. Hyperactivation of this pathway results in abnormal cell growth and proliferation.84 Although most of the cases are due to sporadic mutations in TSC2, TSC1 mutations are more common in familial cases.
TSC may affect the brain in different forms. The name of the disease originates from the presence of firm cortical masses with dimpling resembling potatoes with eyes. Similar nodules may be found in the periventricular zone. The tubers are composed of large, poorly differentiated cells of neuronal and glial origin. Similar cells may be found in the white matter. Abnormal cell differentiation may also produce benign giant cell astrocytomas. In the fetus, the only other organ frequently involved (in up to 50% of cases) is the heart by the development of rhabdomyomata.
TSC is transmitted as an autosomal dominant trait with high penetrance but variable expression, which may cause mildly affected individuals to be undiagnosed. The vast majority of cases are sporadic (Table 7–4). Following the prenatal diagnosis or the birth of an affected patient, both parents should be evaluated for the presence of subclinical disease.
Author (Year) | Number of Fetuses | Week of Diagnosis | Single, Multiple | Outcome | Need for Treatment | TSC in Family | Neurodevelopmental Delay |
---|---|---|---|---|---|---|---|
Pipitone et al89 (2002) | 9 | 27–36 Mean 30.0 | 1 single, 8 multiple | 9 delivered (1 NND) | 5 | 7/80/9 | NS |
D’Addario et al90 (2002) | 6 | 23–37 Mean 30.0 | 3 single, 3 multiple | 6 delivered | 1 (died) | 3/60/6 | NS |
Gamzu et al91 (2002) | 18 | 21–33 Mean 27.1 | 12 single, 6 multiple | 12 delivered, 6 TOP | 0 | 7/123/18 | NS |
Bader et al92 (2003) | 20 | 19–37 Mean 28.4 | 2 single, 18 multiple | 18 delivered 2 TOP-IUFD | 7 | 15/194/20 | 6/18 |
Tworetzky et al93 (2003) | 42 | 15–38 Mean 28.5 | 9 single, 33 multiple | 34 delivered, 8 TOP-IUFD | 8 | 33/4210/42 | 85% (of prenatal + postnatal group) |
Fesslova et al94 (2004) | 13 | 21–36 Mean 26.0 | 5 single, 8 multiple | 9 delivered, 4 TOP | 0 | 9/112/13 | 5/9 2 neurosurgery |
Chao et al95 (2008) | 11 | 18–39 Mean 27.5 | 5 single, 6 multiple | 8 delivered (2 NND), 3 TOP-IUFD | 2 | 3/62/11 | NS |
Saada et al96 (2009) | 51 | 21–37 Mean 27.1 | 10 single, 41 multiple | 25 delivered, 26 TOP | 5 (1 died) | 39/518/51 | 9/20 With MRI + 67% With MRI – 33% |
Total | 170 | 15–38 | 47 single, 123 multiple | 121 delivered (3 NND), 49 TOP-IUFD | 28/121 | 116/15529/170 | 20/47 |
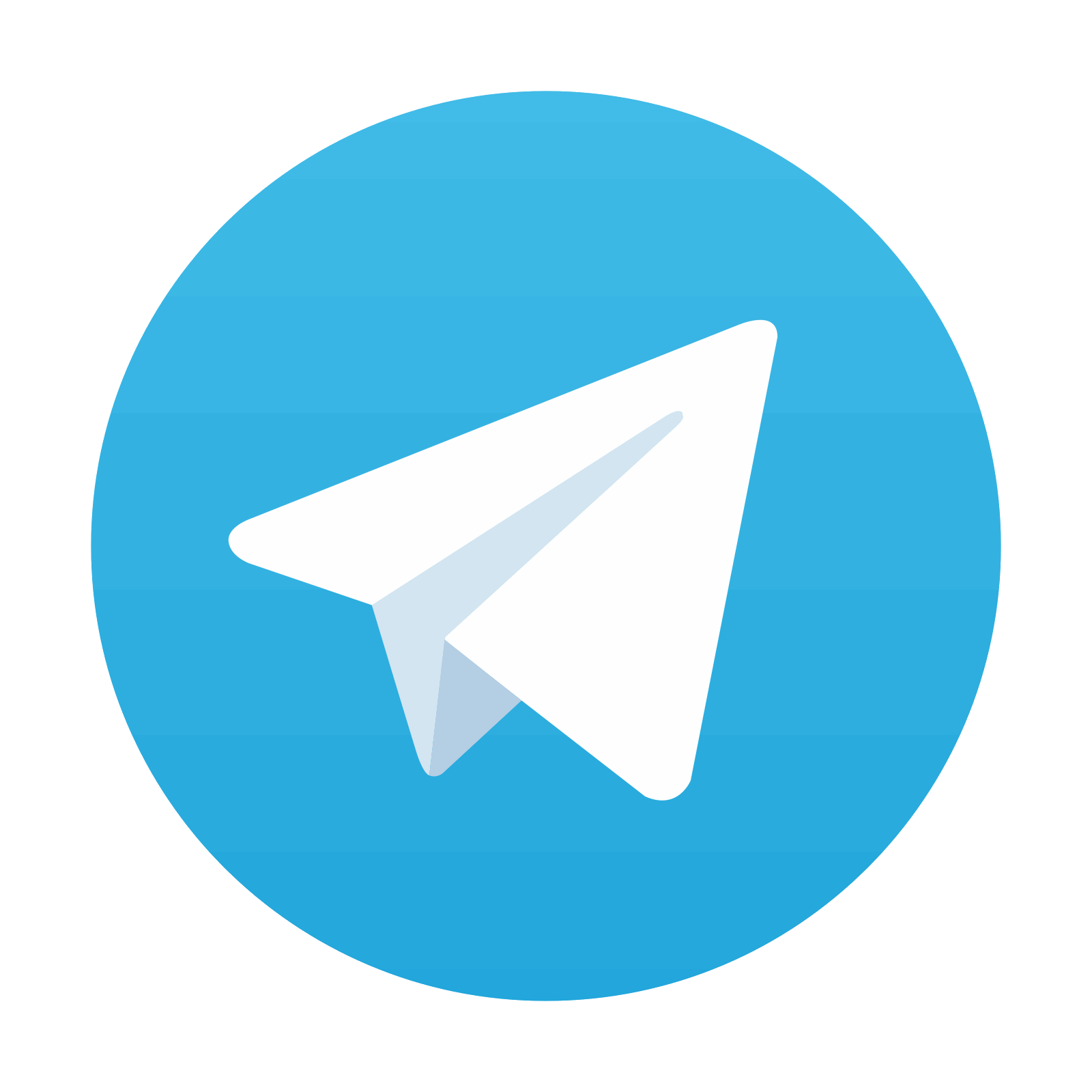
Stay updated, free articles. Join our Telegram channel

Full access? Get Clinical Tree
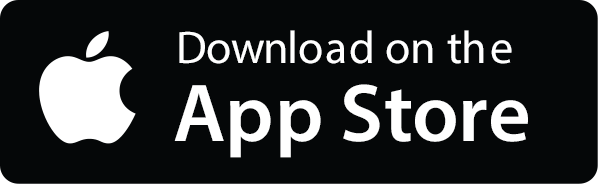
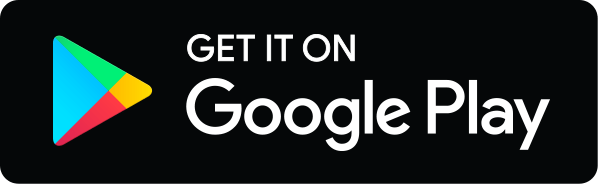
