© Springer Science+Business Media New York 2014
Daniel J. Mollura and Matthew P. Lungren (eds.)Radiology in Global Health10.1007/978-1-4614-0604-4_77. Medical Imaging Safety in the Developing World
James T. DobbinsIII1 , Donald P. Frush2 , Christopher J. N. Kigongo3 , James R. MacFall4 , Robert E. ReimanJr.5 and Gregg E. Trahey6
(1)
Medical Physics Graduate Program, Departments of Radiology, Biomedical Engineering, and Physics, and Carl E. Ravin Advanced Imaging Laboratories, Duke University Medical Center, 2424 Erwin Road, Suite 302, Durham, NC 27705, USA
(2)
Department of Radiology, Medical Physics, Duke University Medical Center, 3808, Radiology, Durham, NC 27710, USA
(3)
Department of Medicine-Gastroenterology, Duke University Medical Center, 200 Trent Drive, Durham, NC 27710, USA
(4)
Department of Radiology, Duke University Medical Center, Erwin Road, Durham, NC 27710, USA
(5)
Radiation Safety Division, Duke University Medical Center, 3155, Durham, NC 27710, USA
(6)
Department of Biomedical Engineering, Duke University Medical Center, Room 136 Hudson Hall, 100 Science Drive, Durham, NC 27708, USA
Introduction
Advances in medical imaging have led to substantial improvements in medical diagnostics in the past several decades. From simple applications such as X-ray and ultrasound imaging to more complex procedures such as magnetic resonance (MR) imaging, positron-emission tomography (PET), computed tomography (CT) angiography, and the burgeoning field of molecular imaging, the ability to record anatomical and functional information in viewable form has greatly improved the ability of physicians to diagnose disease and monitor effectiveness of treatments. While medical imaging is generally quite safe, there are important safeguards that need to be observed in order to minimize risk from these procedures to patients, staff, and the general public. This chapter will provide an introduction to the types of safety concerns that may be relevant to imaging in a resource-limited region. This chapter will not cover all possible hazards, nor will it provide a “cook-book” approach to discovering and mitigating safety concerns; rather, it will provide the reader with an idea of the types of issues that may be of importance in a developing world context. The reader must engage the services of qualified safety experts to assess and address the specific safety issues in a given imaging installation.
As discussed elsewhere in this book, there is an unfortunate disparity in access to many types of medical imaging procedures in resource-limited regions of the world. As a result, many of the more advanced medical imaging techniques and devices may not be available in a given location. Recognizing that there may be a wide disparity in the types of devices available, a general description of both elementary and advanced imaging procedures will be given. The term elementary procedures refers to techniques that are generally available in most locations and includes projection X-ray imaging and ultrasound. Advanced procedures include everything else, such as CT, MR, and nuclear medicine. In many settings in the developing world, only elementary procedures are available, and thus they will receive the most attention in this chapter.
Context for Considering Medical Imaging Safety in the Developing World
There are several features of operating a healthcare facility in the developing world that present particular challenges regarding safety that might not be experienced in more developed regions. While this list is not exhaustive, such challenges include the following: (1) lack of adequately trained personnel to install and service equipment, (2) lack of required test equipment to verify safe operation, (3) difficult access to parts for service or maintenance of equipment, (4) lack of adequately trained personnel to properly operate equipment, (5) lack of properly trained personnel to identify issues that need attention (e.g., image quality issues that are indications of equipment malfunction or need for service), (6) lack of equipment that is up to contemporary standards, (7) self-referral by patients without consulting a qualified healthcare provider, and (8) lack of adequate regulatory or governmental oversight bodies to monitor proper installation and use of equipment. As one can see, many of these issues relate to the need for adequate infrastructure of trained staff.
Another concern is the effect of limited resources on the ability of a healthcare facility to obtain equipment that is in good working order. The authors are aware of several anecdotal cases where imaging equipment was donated to a hospital or healthcare facility in a resource-limited region without adequate finances to install or maintain the equipment. In one case, in a hospital associated with a medical school, there were several pieces of X-ray equipment that had been donated but were not operable due to lack of parts. Some of these devices were not even unpacked from their shipping crates. In other cases, X-ray equipment had been installed but was not functional due to lack of parts to repair the equipment. In still other settings, ultrasound equipment had been donated but stood unused due to inadequate training on operating the probes or even a lack of acoustic gel to use with the device. Thus, in certain settings in the developing world, a lack of financial resources makes it virtually impossible to install or use imaging equipment properly, which can have ramifications for safety as well as optimal clinical utilization. Clearly, a lack of resources is a major issue confronting imaging in the developing world, and there are no easy answers to the problem of limited resources.
In many developing countries, medical services are funded and set up by governments, which unfortunately are often stretched thin by multiple demands for resources and are unable to meet the full need for medical services in the population. This situation has paved the way for competing private medical outlets to emerge. Private imaging centers compete with physicians regarding who sees a patient first, resulting in unnecessary exposure of the patients and staff to risks from exams that are not appropriately authorized based on medical need. Furthermore, the increasing penetration of the Internet in resource-limited regions has resulted in the public accessing more information on medical issues, some of which has been used to demand particular services without referral by a physician or credentialed healthcare provider. Some independent private centers are under pressure by patients to provide imaging services without appropriate medical supervision, thus causing concerns for safety due to overutilization.
In the developing world context, there is more likely to be a wide disparity in the types of imaging utilization between large cities and rural environments than in more developed areas. For example, in the USA, Europe, and in many parts of Asia, if a particular imaging device (such as CT or MR) is not available in a rural region, then patients are referred to imaging centers generally not far away where such devices are available. Transportation to such advanced imaging facilities is generally not prohibitive in cost to the patient. However, in the developing world context, there can be a substantial difference in the type and quality of equipment in more distant regions than in the larger cities. It is not uncommon for there to be no advanced imaging (CT, MR, nuclear medicine) in regional medical centers serving up to a million people in under-resourced regions. At such locations, the only imaging may be an X-ray or ultrasound machine, and some of these may be of substantially lower quality or more antiquated than is common in the larger cities. Also, it can be a significant hardship on patients or families to travel to larger cities to have more extensive imaging performed. Thus, there tends to be a wider disparity between the imaging devices available in rural and urban settings in the developing world, making it more difficult to generalize about the types of imaging safety requirements needed in these countries.
In the developed world, there are usually well-defined standards by which devices are used and maintained that do not vary substantially from region to region. Thus, if one has an X-ray, CT, or MRI scan done in one region, the risk is likely to be similar to that in other regions. In the developing world, one may find that regulations, training, and utilization may vary substantially from place to place, further making it difficult to establish appropriate safety standards and practices. All of these considerations make it difficult to implement a “one-size-fits-all” approach to imaging safety in the developing world.
One then faces the ethical dilemma of whether it is better to provide some medical imaging in a place where all safety issues may not be addressable or whether it is better to ensure that all imaging be done under the highest accepted standards of safety, realizing that such constraints may mean that some places will not have adequate imaging devices available. Resolving this ethical debate is important but beyond the scope of this chapter. We would simply point out that the reader may face a situation where limited resources will impact upon the ability to perform some recommended safety procedures. It will be up to the reader to consult qualified safety experts to determine what safety procedures are feasible and essential in a given location. The authors emphasize, however, the importance of addressing all safety issues adequately. While providing access to medical care is a matter of ethical concern, it is also a matter of ethics to ensure that one does not jeopardize the safety of one’s patients, staff, or the general public in the process.
Importance of Minimizing Dose from Ionizing Radiation
While the use of ionizing radiation in medical imaging has led to vast improvements in diagnostic capabilities and is generally safe, there are two factors that have led to increasing concern over the level of radiation dose delivered to patients. These factors are (1) cases of radiation injury from improperly used equipment and (2) an upward trend in the overall dose to the population from increased utilization of techniques such as CT imaging. These two factors highlight different ways in which ionizing radiation can pose a hazard. The first factor (radiation injury) causes a hazard to individual patients through damage to tissues. The second factor (stochastic effects) causes risk by increasing the statistical likelihood of inducing cancer in an individual patient or producing genetic mutations in the population at large from imaging studies. Risks from both of these must be minimized.
Several recent high-profile cases of radiation injury have demonstrated the importance of using proper imaging protocols and having adequately trained staff. In one such case, erythema and hair loss were caused by an improper protocol for using CT in brain perfusion studies. Over 200 patients were affected, and this case made news in the popular press [1]. It was discovered that the imaging center was delivering about eight times the correct radiation dose for this type of exam. As a result of this and other cases of overexposure, a new law was passed in California that requires CT scanners to be accredited, radiation dose to be recorded for CT exams (if the scanner is capable of such recording), and that radiation doses exceeding 20 % above what was intended be reported to the state Department of Public Health, among other provisions [2]. In other reported cases, there have been instances of skin toxicities (including necrosis) due to excessively long exposures to fluoroscopically guided interventional procedures such as cardiac catheterization [3]. While these events are rare, they point out the possible hazard from using exams with the potential for high radiation doses.
In cases of radiation injury, harm is caused to specific patients. More difficult to track are the effects from increased utilization that has led to an increase in the overall radiation dose to the population at large. In the 1980s, the per capita average radiation dose to a person living in the USA was 3.7 mSv/year, of which 14 % was due to medical imaging. By 2006, the average annual radiation dose to a person in the USA was 6.2 mSv, with 48 % due to medical imaging. The vast majority of this increase in population-wide radiation exposure was from increased utilization of CT imaging and nuclear cardiology procedures [4]. Devices such as dual-source, dual-energy CT machines with 0.3-s rotation time have opened the possibility for uses of CT in new and expanded applications such as cardiac angiography. Such high-tech imaging is often unfortunately not as readily available in the developing world, and as such, there will not likely be as rapid an increase in CT utilization as has been experienced in developed regions. While the overall increase in population dose from CT in developing regions is likely to trail that of the developed world, there is still a global concern for dose control from increasing utilization of CT.
The concern for patient dose from medical imaging is particularly important in pediatric patients. The use of imaging, especially in the emergency department, has increased in both adults and children. Overall, about 3.5 billion examinations using ionizing radiation have been performed per year globally. Approximately 10 % of all imaging examinations in the USA are performed in the pediatric age group [5]. The use of CT has increased over the past two decades in the emergency setting [6], and in one large population, nearly 43 % of children underwent evaluation using ionizing radiation in a 3-year period, and nearly 8 % of the study population had a CT over the same period [7]. Doses for some modalities, particularly CT, can be quite high when compared to exams such as chest radiography.
The pediatric population is a relatively more sensitive group than the adult population due to more vulnerable tissues and a longer lifetime in which to manifest radiation-induced cancer. Pediatric imaging evaluation may be more complicated than that for adults for several reasons. First, the clinician who does not regularly care for children may be unfamiliar with the spectrum of pediatric diseases, and inappropriate imaging evaluations may be requested. Second, there is often a great deal of anxiety in the setting of an acutely injured or ill child that may prompt more aggressive imaging evaluation. Third, the actual imaging evaluation of the child may be carried out by individuals who are relatively less experienced with technique and technical factors, which may result in an increased number of images being obtained, suboptimal projections or technical factors, or inappropriate CT parameter selection that may provide excessive radiation exposure. Recent data on global CT practices indicated a great deal of variability in justification and utilization [8, 9]. Finally, there is a recognized elevated responsibility for the protection of children. Of note, recently, the first connection between CT examinations and increased risk for both leukemia and brain cancer was reported, and this was discovered in children [10]. These factors contribute to increased public scrutiny and regulatory surveillance of medical imaging in children, and mandate a responsibility to manage radiation dose by all involved with imaging practice.
Safety for Patients
There are several key components to ensuring a safe imaging environment for patients. First, it is important to ensure that a proper examination has been requested, so that patients do not undergo needless radiation or other exposures that do not contribute meaningfully to proper medical diagnostic evaluation. Ordering proper examinations depends heavily on having a well-trained staff of healthcare providers, both referring physicians and radiologists. In remote settings where there may not be a full complement of medical specialties, there should be some mechanism for physicians who recommend imaging examinations to consult with radiologists by phone, email, Skype, or other electronic means if there is uncertainty about the most appropriate diagnostic imaging procedure to order. Proprietors of imaging centers should advise their patients of the need to get a physician’s referral prior to seeking imaging tests and should not offer procedures without such referrals. Second, there needs to be a thorough review of the imaging equipment by trained and qualified medical physicists, radiation safety personnel, and service engineers to ensure that equipment has been properly installed, maintained, and evaluated for safe operation. A medical physicist should carefully check to ensure that appropriate filtration, shielding, and imaging protocols are established. Third, it is important that the staff who acquire the images be adequately trained on how to use the equipment properly and safely, and that they understand the proper imaging protocol for a given exam. It is important for each institution to have properly vetted and posted imaging protocols for all exams that will be performed. Often, individuals taking diagnostic images will be trained in radiologic technology, but the specific requirements for a given jurisdiction should be followed. Fourth, it is important to ensure mechanical and electrical safety for all equipment that is used on patients. Some X-ray imaging equipment, for example, is quite heavy, and proper engineering must be done to ensure that overhead equipment is properly mounted. Electrical safety should be evaluated on all equipment as well. Properly trained installation or service staff should be consulted. Governments and professional bodies should ensure regular inspections of imaging facilities. Fifth, in the case of magnetic resonance imaging, it is important that patients be evaluated for metallic implants or objects that could give rise to local heating or other deleterious effects. Also, patients undergoing magnetic resonance imaging should be evaluated for the potential for claustrophobia and appropriate remediation measures taken if the patient suffers from that condition.
Another important feature regarding imaging safety is ensuring good image quality. While image quality may not initially be considered a safety issue, it has a potential impact on safety because images of low quality may need to be repeated or may provide inadequate information or may lead to erroneous clinical decision-making. Covering all of the issues related to image quality is beyond the scope of this chapter, but some issues in X-ray image quality include having proper beam filtration, choosing appropriate kVp, having adequate tube current (mA) in order to use short exposure times that do not result in motion blur, and using anti-scatter grids (when appropriate) to minimize scattered radiation. With digital imaging devices, it is also important to properly select image-processing parameters. It is important to have a qualified medical physicist to evaluate a given imaging installation for proper image quality and to work with the radiologist on staff to select image-processing parameters that suit the clinical need and the preferences of the radiologist.
The above list of safety factors for patients is not exhaustive, and there may be additional safety issues in a given healthcare setting that require attention. It is important to engage appropriately trained individuals to evaluate these issues related to patient safety before any imaging is performed at a new facility, and also periodically afterwards to ensure continued safety. There may also be regulatory issues in a given location that must be understood and followed. It would be important to communicate with the Ministry of Health or appropriate professional bodies in a given country to learn about requisite regulatory issues.
Safety for Staff
Many of the same safety concerns listed above for patients also apply to staff. For example, it is important to ensure the mechanical and electrical safety of all equipment. There are other safety issues related to staff, however, that go beyond those for patients. One of the primary concerns for safety of staff is ensuring the appropriate level of understanding regarding how to properly use the equipment. In addition to putting patients at risk, if the imaging staff is not properly trained in equipment operation then it is possible that staff may expose themselves to unnecessary risk. One key way in which staff are subject to risk is through repeated exposure to ionizing radiation. There are standards in the USA and other countries for the amount of radiation exposure an occupationally employed person is allowed to experience as part of their work duties. These levels of radiation are specific to work-related exposures and do not include any medically related exposures for the individual’s own medical care. The limit for occupational exposure in the USA is 50 mSv/year. Staff should be continuously monitored for their occupational exposure to radiation, through the use of “radiation badges” or equivalent monitoring devices. It is especially important to set up proper safety equipment and training for staff that will be involved in high-exposure procedures such as fluoroscopy, nuclear medicine, and CT. In many of these cases, staff must wear approved radiation-blocking aprons. In the case of fluoroscopy, there should be adequate hanging leaded barriers to shield the operator from scattered radiation from the patient. It is important for the staff to understand the proper location for wearing radiation-monitoring badges when wearing leaded aprons.
Special attention must be paid to the protection of the developing embryo and fetus in pregnant staff members, based upon the principles that rapidly dividing, undifferentiated tissues are highly sensitive to the adverse effects of ionizing radiation. In the USA, pregnant women who have declared their pregnancy to their employer, and are exposed to ionizing radiation as a consequence of their employment, are subject to a dose limit of 5 mSv during the period of gestation. It is also recommended that dose be limited to 0.5 mSv during each month of gestation. Compliance with dose limits may be documented by having the woman wear a passive radiation dosimeter at waist level, on the front of the body, which is changed monthly. If the employee wears a shielding garment, the dosimeter is generally placed underneath the garment in order to get the best estimate of fetal dose. In addition to appropriate shielding, other methods of limiting fetal dose should be undertaken. These include minimizing the amount of time spent in radiation fields and maintaining the maximum practicable distance from radiation sources.
Safety for the General Public
In addition to considerations of safety for patients and staff, steps must also be taken to minimize risk to the general public when setting up an imaging facility. Certain principles apply to providing proper shielding for ionizing radiation in X-ray facilities and protection from harm due to the magnetic field in MR installations. In certain jurisdictions, there are regulatory considerations for public safety that must be met.
One of the main types of protection for the public is providing shielding from high-energy photon radiation in X-ray, CT, or nuclear medicine. In some health facilities in the developing world, old rooms have been repurposed to house X-ray machines but without regard for appropriate shielding based on activities in surrounding parts of the building. In one facility familiar to one of the authors, an X-ray room was placed adjacent to a postnatal room and another high-traffic clinical area. The room originally served as a storeroom for the facility, but after acquiring an X-ray machine by donation, the room’s role was changed to that of an X-ray facility without adequate attention to shielding. This example indicates how easily the public could be exposed to the risk of ionizing radiation from imaging equipment.
The number of considerations in shielding design is too extensive to be covered in detail in this chapter, but a qualified medical health physicist can work with a given imaging installation to provide a suitable shielding design. The basic principles of shielding include providing a barrier in walls, ceilings, and floors where the public (or employees) may be present; shielding of scattered radiation, such as from the patient and imaging hardware; and shielding of leakage radiation from the X-ray tube housing [11]. Structural shielding is typically in the form of lead that is constructed into the wall. In buildings constructed of concrete, the concrete barrier in the floor and ceiling may be able to serve as part of the barrier, if thick enough. Doors can be purchased that have lead lining. For places with windows that separate workers or the public from a radiation area, leaded glass is available.
The general considerations for shielding calculations include the type of ionizing radiation, the distance from the point of emanation to the location of a person who could be exposed, the “workload factor” (the approximate amount of time per week that the radiation is present), the anticipated average intensity of radiation emitted, the fraction of time that a radiation beam will be directed at the intended barrier, and the anticipated occupancy of the area beyond the barrier. If a barrier separates an X-ray room from a closet that is rarely occupied, for example, less shielding may be needed than for a barrier facing a waiting room. Thus, it is not enough to merely consider that an X-ray machine may be present; it must also be taken into consideration what types of X-ray exams may be performed, and what the environment is like surrounding the X-ray room. Safety for nuclear medicine facilities also includes proper shielding for holding the sources and adequate safeguards for securing the radionuclides (e.g., locked cabinets with access to the key limited to authorized personnel).
In magnetic resonance facilities, it is important to keep the general public outside of the strong magnetic field of the magnet. Barriers should be provided that limit access to regions with strong magnetic field; these precautions should be taken with regard to the general public and to staff members (such as cleaning staff) who may not be adequately informed of safety hazards.
In all of the considerations for protections of patients, staff, and the general public, trained and authorized safety personnel should provide recommendations, design, and evaluation of safety features for any proposed facilities.
Specific Issues of Importance in Various Imaging Modalities
X-Ray and CT
The most important safety consideration in X-ray and CT imaging is protection from excessive radiation exposure. An excess of radiation exposure can come from a number of different sources, including inadequate equipment, improper imaging protocols, staff inexperience, clinical overutilization, and perhaps surprisingly, from inadequate attention to image quality. Each of these will be discussed briefly.
There are several components to ensuring proper equipment to reduce radiation exposure in radiography. First, there should be adequate beam filtration. Regulations concerning filtration requirements may vary by jurisdiction, but typically the recommended beam filtration amounts in the USA are 0.5 mm Al (or equivalent) for <50 kVp, 1.5 mm Al equivalent for 50–70 kVp, and 2.5 mm Al equivalent for >70 kVp. This filtration removes the lowest energy photons from the beam that are almost all absorbed in the patient, thereby contributing to patient dose but not to the usable image. Filtration is said to “harden” the beam, meaning that it makes the mean beam energy higher. Special filtration considerations may exist for particular types of imaging such as mammography or dental radiography.
A second equipment-related exposure consideration is the use of beam collimators. It is important that the beam be collimated down to the smallest overall field size that can appropriately image the desired anatomy. A trained technologist will know the proper amount of collimation for a given exam, but an inexperienced user may expose too large of an area. Inadequate collimation can lead to unnecessary exposure to regions of anatomy that do not contribute to answering the clinical question at hand. It is also important to ensure that the light field used for patient positioning be adequately aligned with the actual exposed image area. A medical physicist can do an alignment test to test the fidelity of the light field to the X-ray field. Using appropriate collimators also ensures that the beam is restricted in size so that imaging staff are not unintentionally exposed. Following the same principle, adequate shielding of X-ray tube units must be confirmed to ensure that leakage radiation is not exposing patients or staff.
A third factor to consider is the use of anti-scatter grids. X-ray photons interact with tissue in two predominant ways at the energies used in diagnostic radiography: photoelectric effect and Compton scattering. Compton scattering (along with the less prevalent coherent scattering) contributes some to image contrast but also leads to photons that are spread across the detector that add to image fog but not to usable image content. The scattered photons have the net effect of adding to image noise (mottle) and reducing the net contrast of an image. This added image noise may be compensated for by increasing the overall beam flux, thereby increasing patient dose. The loss of contrast can lead to inadequate visualization and consequently may lead to inadequate clinical usefulness of the image. The main way in which scattered radiation is addressed in radiography is through the use of anti-scatter grids. Grids significantly improve image contrast (and thereby, visibility of anatomy) but can require an increase in tube output. It is important to select grids with appropriate parameters for a particular application. For example, with fixed geometry upright chest radiography, it is common to use grids of 12:1 or 14:1 grid ratio (the grid ratio is one measure of a grid’s effectiveness). However, for angiographic use, a lower grid ratio may be used. For bedside imaging, it is customary to use no grid at all (due to the difficulty of positioning the grid in the variable geometry of bedside application). Reduction in scattered radiation can also be achieved by air gaps (a large distance between the patient and the image receptor), and in some rare cases by scanning slit devices.
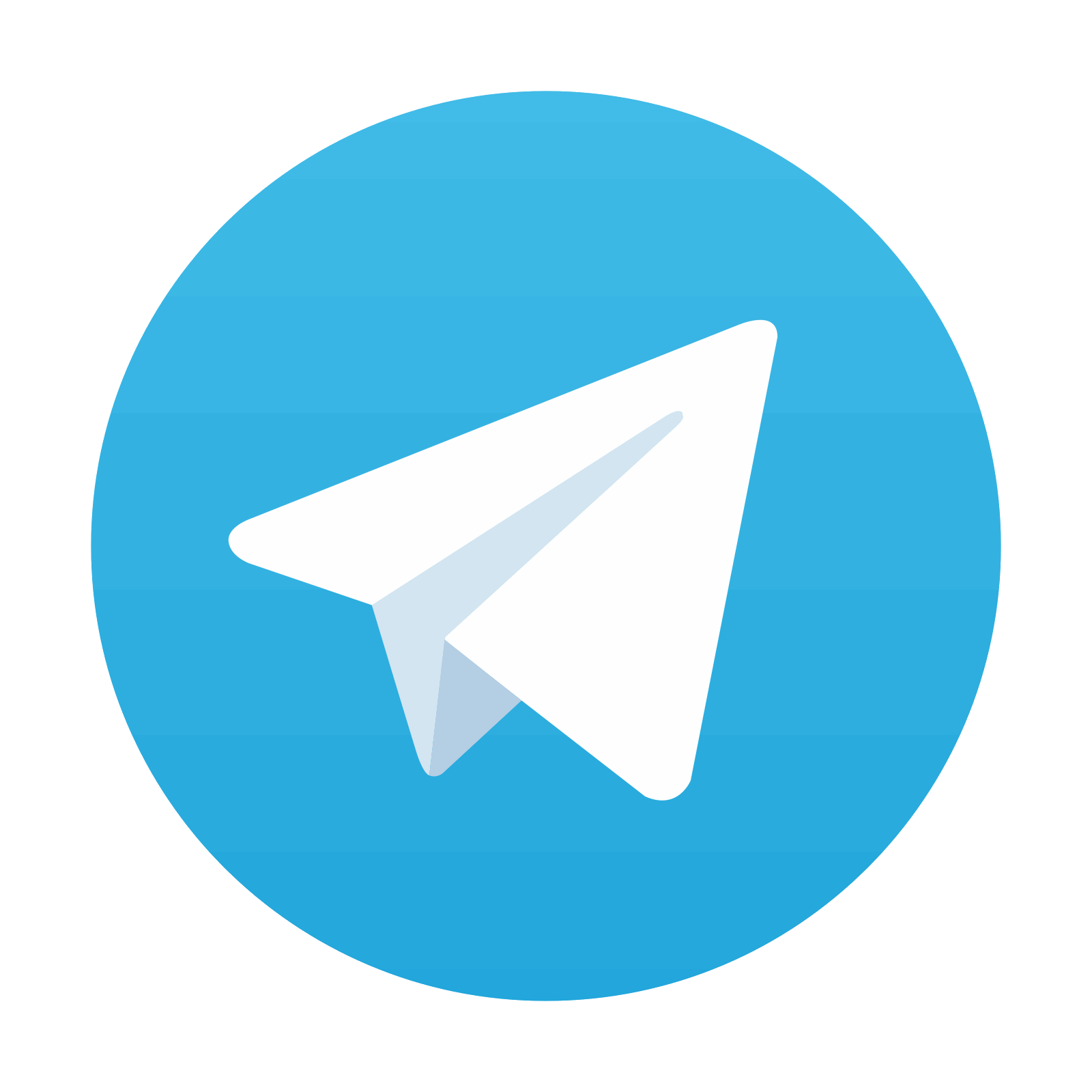
Stay updated, free articles. Join our Telegram channel

Full access? Get Clinical Tree
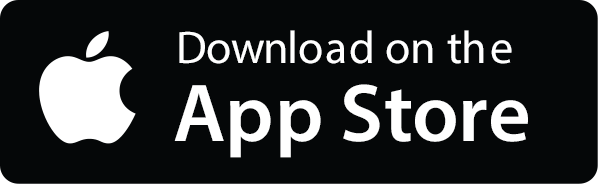
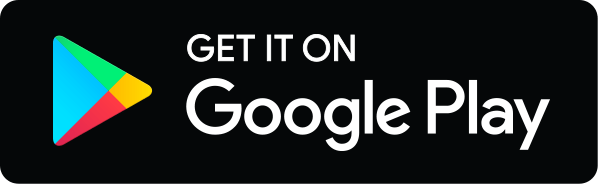