[11C]Clorgyline
[11C]Harmine
[11C]Befloxatone
Selectivity
Excellent (Fowler et al. 1987)
Excellent (Bergstrom et al. 1997b)
Excellent (Dolle et al. 2003)
Reversibility
Not reversible (Fowler et al. 1987)
Highly reversible (Ginovart et al. 2006)
Highly reversible (Bottlaender et al. 2010)
Modeling
2-tissue compartment (Fowler et al. 1987)
2-tissue compartment (human) (Ginovart et al. 2006)
2-tissue compartment (baboon) (Bottlaender et al. 2010)
Reliability
Very good (Fowler et al. 1996)
Excellent (Sacher et al. 2012)
Not reported
Metabolites crossing the blood-brain barrier?
Unlikely
No brain penetrant metabolites (Wilson et al. 2003)
Unlikely
[11C]Harmine demonstrates the properties of an excellent PET radiotracer for an index of available MAO-A density in humans. [11C]Harmine has high affinity for the MAO-A enzyme (K i = 2 nM) with high brain uptake in humans and is selective for MAO-A (Bergstrom et al. 1997b; Ginovart et al. 2006; Meyer et al. 2006). The affinity of [11C]harmine is three orders of magnitude higher for MAO-A than MAO-B (Bergstrom et al. 1997a). Displacement studies in baboons using MAO-A selective inhibitors show complete displacement of [11C]harmine in regions with high density of MAO-A (Bergstrom et al 1997a). The uptake is specific to the parent compound as metabolites of harmine are polar and do not cross the blood-brain barrier (Tweedie and Burke 1987). The uptake of [11C]harmine is highest in brain regions with greater MAO-A density such as the cortex and lowest in regions with low MAO-A density such as white matter (Bergstrom et al. 1997c). The MAO-A V T (MAO-A distribution volume) is primarily an index of specifically bound [11C]harmine at equilibrium since 80–85 % represents specific binding and the levels of free and nonspecific binding are similar among different people (Ginovart et al. 2006; Sacher et al. 2011). The modeling of this compound in humans best fits a two-tissue-compartment model, and negligible bias is found when the Logan model is applied (Ginovart et al. 2006).
25.1.2 Major Depressive Disorder
The in vivo aspect of neuroimaging offers the ability to readily sample medication-free subjects and to selectively choose early-onset major depressive disorder (MDD) which is the most common form (WHO 2008). Late-onset MDD is often attributable to neurodegenerative diseases such as Parkinson’s disease and Alzheimer’s disease (Krishnan 2002), which would be expected to have very different pathologies from early-onset MDD. Since postmortem studies often preferentially sample older subjects, sometimes they are not targeted towards early-onset MDD. The first three postmortem studies of MAO-A levels and activity had not selectively targeted early-onset MDD, and the early perspective of the field was that there was no abnormality of MAO-A level or activity in MDD (Mann and Stanley 1984; Sherif et al. 1991; Ordway et al. 1999).
In 2006, MAO-A V S, an index of MAO-A density, was measured using [11C]harmine PET in medication-free major depressive episodes secondary to early-onset MDD (Meyer et al. 2006). Subjects with major depression (MDE) were drug-free for at least 5 months, and most were antidepressant naive. All MDE subjects and controls were otherwise healthy. The MAO-A V S was highly significantly elevated (p < 0.001 each region, average magnitude 34 % (or two standard deviations)) during MDE (see Fig. 25.1). This was the first definitive study of MAO-A binding in MDE from early-onset MDD: The samples excluded comorbidity, thereby focusing on the differences between this common, early-onset type of MDD and health. The effect size was large, and the radiotracer has outstanding qualities for measuring MAO-A binding. In 2008, Barton et al. (2008) reported a consistent finding of elevated brain serotonin turnover in unmedicated depressed patients. In 2009, the finding of greater MAO-A binding in MDE was replicated with [11C]harmine PET, and in 2011 the finding was replicated in antidepressant-free MDE subjects in postmortem study of orbitofrontal cortex applying Western blot (Johnson et al. 2011; Meyer et al. 2009).
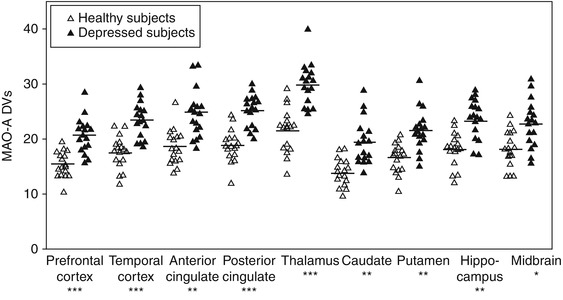
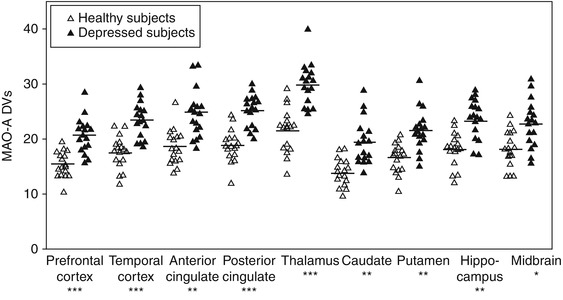
Fig. 25.1
Comparison of MAO-A DVs between depressed and healthy subjects. On average, MAO-A DVs was elevated by 34 %, or two standard deviations, in depressed individuals. Differences between groups were highly significant in each region: *p = 0.001, **p = 1:1.0001, ***p < 0.00001 (Reprinted from Meyer et al. (2006))
Greater MAO-A binding in affect-modulating regions has important implications for understanding the pathophysiology of MDD. A key long-standing theory of MDD is that monoamines were low, but no clear mechanism of monoamine loss had been previously identified, and this finding represents the clearest mechanism to support this theory (Meyer et al. 2006). In addition, since MAO-A has multiple functions, metabolizing serotonin, norepinephrine, and dopamine, oxidizing, and facilitating apoptosis (Youdim et al. 2006), it suggests that new antidepressants should optimally target these additional functions rather than solely raise extracellular serotonin. Such approaches have notable potential to address the issue that at least 40 % of MDE does not respond to the common first-line treatment of selective serotonin reuptake inhibitors (Trivedi et al. 2006).
Investigations of MAO-A V T, or monoamine oxidase distribution volume, were subsequently extended into different states of MDD, an approach which is readily feasible with positron emission tomography. While it might be expected that MAO-A levels would normalize with euthymic mood, the recovered state of MDD is also a state of high risk for another MDE. The risk for a recurrent MDE over 2 years is 20–50 % depending upon treatment conditions (Frank et al. 1990; Fava et al. 2004). Elevated MAO-A V T may be considered an index of a monoamine lowering process, and in the 1950s during treatment with reserpine-based antihypertensives, it was discovered that chronic monoamine lowering is associated with subsequent onset of MDEs which typically occurred 2 weeks to 4 months later (Freis 1954). In recovered MDD, MAO-A V T was significantly elevated in prefrontal cortex, anterior cingulate cortex, striatum, hippocampus, thalamus, and midbrain in a sample of 18 medication-free recovered MDD subjects compared to 28 healthy controls (Meyer et al. 2009). Recovered MDD subjects who had recurrence of their MDE in the subsequent 6 months had the highest levels of MAO-A binding in the prefrontal and anterior cingulate cortex at the time of scanning (Meyer et al. 2009). The prefrontal cortex and anterior cingulate cortex were of particular interest because these regions (and/or subregions of these structures) are activated in mood induction studies (Liotti et al. 2002) and during cognitive functions like pessimistic perspective that generate sad mood (Sharot et al. 2007; Tom et al. 2007). To address the possibility that other factors related to risk of recurrence did not contribute to the finding, subjects were medication-free for at least a year, had no cognitive behavioral therapy within 3 years, were currently asymptomatic, and had no comorbid medical, psychiatric, or substance abuse illnesses. Given the link between elevated MAO-A binding in prefrontal and anterior cingulate cortex and subsequent MDE, this argues that new interventions are needed to decrease MAO-A levels in these brain regions even beyond the duration of a therapeutic intervention so as to prevent recurrence.
25.1.3 Early Postpartum
Early postpartum is a time of greater risk for three types of mood disturbance (O’Hara and Swain 1996). The first is the common, postpartum blues, present in up to 75 % of women involving mild sadness and can be accompanied by some trouble sleeping, irritability, desire for isolation, and difficulty concentrating (O’Hara and Swain 1996). It is considered within the healthy range of experience, typically starting on day 4 postpartum and finishing within the first week. The second is postpartum depression, which is a MDE that occurs within the first 4 weeks after giving birth, although some definitions include onset within the first 3 months after giving birth (O’Hara and Swain 1996; American Psychiatric Association 1994). MDE in postpartum is the most common complication of childbearing with a 13 % prevalence over the first 3 months (O’Hara and Swain 1996). The third is a rare, serious condition of postpartum psychosis (which may be accompanied by a MDE) occurring in 0.2 % of deliveries.
In contrast to postmortem investigations, MAO-A neuroimaging is well suited to postpartum study, even though it is challenging for subjects to organize their time to come for an imaging scan. While several studies reported an inverse relationship between decline in estrogen and rise in MAO-A levels, mRNA, and activity (Luine et al. 1975; Ma et al. 1993, 1995; Holschneider et al. 1998), prior to 2010, MAO-A binding, mRNA, or activity had never been studied in any species in early postpartum. Given that estrogen levels are more than 100-fold over the first several days in humans (O’Hara et al. 1991), a [11C]harmine PET study measured MAO-A binding during early postpartum (days 4–6) (Sacher et al. 2010). A highly significant elevation of MAO-A binding was found, which was, on average, 43 % greater across the brain regions assayed (prefrontal cortex, anterior cingulate cortex, striatum, thalamus, hippocampus, midbrain) as compared to women not recently pregnant (Sacher et al. 2010). A voxel-based analysis demonstrated that the elevation in MAO-A binding was present throughout the gray matter of the brain (see Fig. 25.2) (Sacher et al. 2010).
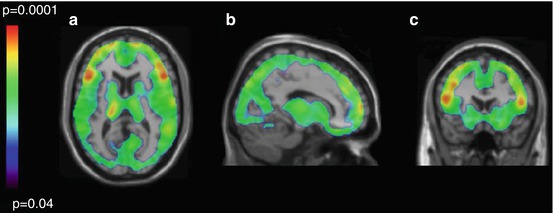
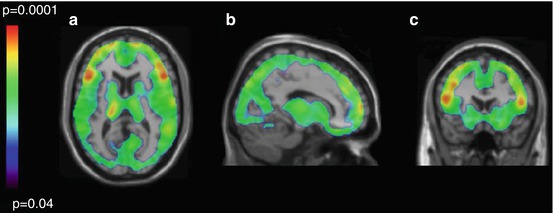
Fig. 25.2
Regional distribution of p-values reflecting elevated MAO-A binding in immediate postpartum period. Parametric maps of elevated monoamine oxidase A binding in the postpartum group versus the control group. Maps are superimposed on a T1-weighted magnetic resonance image that is normalized to the T1-weighted template (SPM2; Department of Cognitive Neurology, Wellcome Trust Centre for Neuroimaging, London, UK). (a) Transverse. (b) Sagittal. (c) Coronal. Individual voxel threshold was set at p < 0.05; 86 412 voxels comprised a single cluster, which had a cluster-corrected significance of p = 0.03. Mean regional difference was 43 % (Reprinted from Sacher et al. (2010))
This study has several major important implications. The first is that this provides a new neurobiological explanation for postpartum blues, involving a rapid decline in estrogen, followed by a rapid rise in MAO-A levels in affect-modulating structures in the brain, with subsequent sad mood and symptoms of postpartum blues (Sacher et al. 2010). The second is that since elevated MAO-A V T is associated with both MDE and subsequent onset of MDE, elevated MAO-A V T in early postpartum provides a mechanistic explanation for the high risk of MDE in early postpartum. The third is that there may be an opportunity to develop strategies to prevent postpartum depression, based upon these neurobiological models. Greater severity of postpartum blues, which typically occurs before postpartum depression, is associated with greater risk for postpartum depression (PPD) (O’Hara et al. 1991). Thus, reducing the intensity of postpartum blues might reduce the risk for PPD, and this may be done by specifically targeting the pathway of estrogen decline, rise in MAO-A levels, and excessive metabolism of monoamines. For example, it is possible that amino acid supplementation with monoamine precursors might compensate for monoamine loss and reduce severity of postpartum blues.
25.1.4 Cigarette Smoking
Most biological descriptions of early cigarette withdrawal focus upon abnormal modulation by nicotine of dopamine-releasing neurons projecting from the ventral tegmental area to ventral striatum (Laviolette and Van der Kooy 2004; Brody et al. 2006; Cosgrove et al. 2009). This model has been successful in clinical applications since therapeutics targeting this pathway, when combined with therapy, achieve 6-month abstinence rates of up to 40 % (Law and Tang 1995; Piper et al. 2009). However, there are other central nervous system targets affected by cigarette smoke that may be important in the mechanism of cigarette withdrawal, and several substances in cigarette smoking have been reported to binding with moderate affinity to monoamine oxidase A (MAO-A) (Hauptmann and Shih 2001; Herraiz and Chaparro 2005). Harman is the chemical in cigarette smoke that has the highest affinity for MAO-A (Hauptmann and Shih 2001; Herraiz and Chaparro 2005).
The first PET study of MAO-A binding applying [11C]clorgyline reported reduced available MAO-A binding in those who smoke cigarettes in the active smoking state (Fowler et al. 1996). This finding was confirmed in a recent PET study with the tracer [11C]befloxatone (Leroy et al. 2009). Given that the plasma half-life of the key MAO-A binding substances found in cigarette smoke (harman and norharman) is only an hour (Rommelspacher et al. 2002), there was reason to specifically assess MAO-A V T during both active smoking and withdrawal conditions. When MAO-A V T was assessed in both conditions, it was discovered that prefrontal and anterior cingulate cortex MAO-A V T (as well the other brain regions assessed) rose during withdrawal in those who smoke heavily, that is, more than one pack of cigarettes per day, but not in those who smoke more moderately at less than one pack per day (see Fig. 25.3) (Bacher et al. 2011) and that during withdrawal from heavy smoking, MAO-A V T was greater than in health in all regions assessed (see Fig. 25.4) (Bacher et al. 2011).
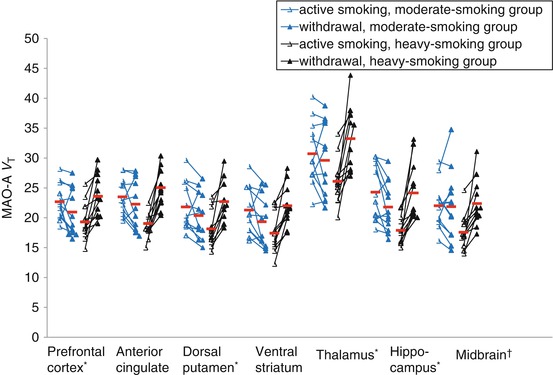
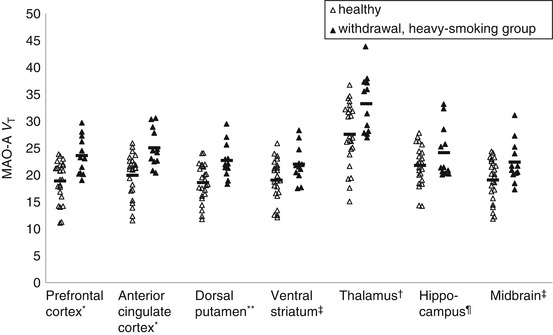
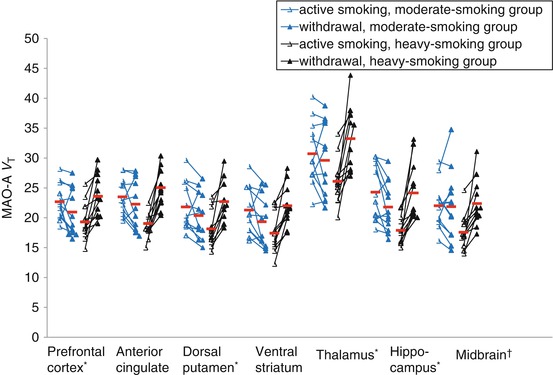
Fig. 25.3
Monoamine Oxidase-A binding in cigarette smoking subjects during active smoking and withdrawal. Repeated measures MANOVA found a highly significant effect between smoking severity and change in MAO-A V T in the prefrontal cortex and anterior cingulate cortex regions (F 1, 22 = 25.58, p < 0.001). Repeated measures MANOVA also found a significant effect between smoking severity and change in MAO-A V T for all the regions assayed (F 1, 22 = 28.24, p < 0.001). The effect was confirmed in each region with a repeated measures ANOVA (interaction between smoking severity and change in MAO-A V T, repeated measures ANOVA, F 1, 22 = 11.16 to 28.87, p = 0.003 to p<0.001), * p<0.001, † p = 0.003 (Reprinted from Bacher et al. (2011))
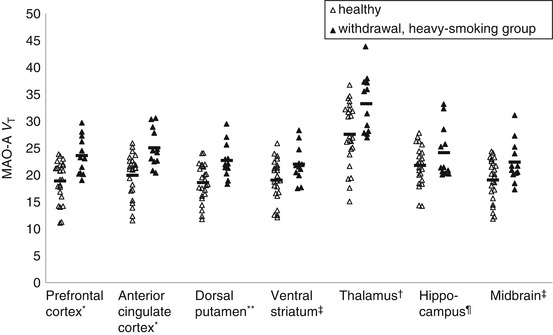
Fig. 25.4
Greater MAO-A binding during acute cigarette withdrawal in heavy-smoking group compared with healthy subjects. MAO-A V T was significantly greater in the prefrontal and anterior cingulate cortex in the withdrawal state of those in the heavy-smoking subgroup as compared to healthy controls (MANOVA, F 2,33 = 6.72, p = 0.004). Most other individual regions were also significantly different (independent t-test) (Reprinted from Bacher et al. (2011)). * p ≤ 0.001, ** p ≤ 0.005, † p ≤ 0.01, ‡ p ≤ 0.1, ¶ p ≤ 0.05
The elevation in MAO-A V T has important implications for the problem of sad mood in people who smoke cigarettes. There is a very high comorbidity between cigarette smoking and major depressive disorder with 50 % of people with MDD also smoking cigarettes, and the pattern is such that cigarette smoking predisposes to MDD and vice versa (Anda et al. 1990; Breslau et al. 1998). It is the heavy cigarette smoking group who are at much greater risk for MDD (Pratt and Brody 2010), and this group had a 25 % elevated MAO-A V T in the prefrontal and anterior cingulate cortex during withdrawal as compared to healthy controls, arguing for a process of elevated MAO-A level during withdrawal as a mechanism to create risk for MDD (Bacher et al. 2011). Hence, repeated exposure of elevated MAO-A level, in the prefrontal and anterior cingulate cortex, a mechanism associated with onset and maintenance of MDE state, occurring after a short period of 8-h withdrawal could explain the predisposition to MDE in people who smoke cigarettes heavily.
A second problem with mood in people who smoke cigarettes is the depressed mood of acute withdrawal (Carey et al. 1993; Kenford et al. 2002). In the imaging study of MAO-A during cigarette withdrawal, in those who smoked heavily, the magnitude of rise in MAO-A V T in prefrontal and anterior cingulate cortex during withdrawal was significantly correlated with the shift in visual analogue scales towards depressed mood (Bacher et al. 2011). This rise in MAO-A V T also correlated with the decline in harman in those who smoke heavily. These results suggest that rapid removal of harman from occupying MAO-A sites leaves a high level of available MAO-A for metabolizing monoamines in prefrontal and anterior cingulate cortex resulting in depressed mood. This argues for testing of MAO-A inhibitor treatments in people who experience sad mood during early cigarette withdrawal as a strategy to assist in quitting, an important issue since 50 % of people tend to relapse in the first few days of trying to quit cigarette smoking (Law and Tang 1995; Garvey et al. 1992).
25.1.5 Aggression
Aggression has major social costs since 50 % of prisoners have problems with aggression (Fazel and Danesh 2002). Substantial evidence has accumulated to suggest that inherited levels of MAO-A may also have influence upon neurodevelopment and lead to impulsive behavior: MAO-A knockout (KO) and wild-type mice given MAO-A inhibitors during development demonstrate elevated brain serotonin during development, which disrupts the organization of somatosensory thalamocortical afferents and abnormalities in the somatosensory cortex barrel fields (Cases et al. 1996; Vitalis et al. 1998; Mejia et al. 2002). It has been proposed that impulsive aggression observed in MAO-A KO mice and mice given MAO-A inhibitors during development is consequent to impairment in organizing sensory input secondary to these abnormalities (Bortolato et al. 2008). Men who are deficient in MAO-A, consequent to an X chromosome mutation that impairs function of the MAO-A gene, demonstrate frequent aggressive behavior (Brunner et al. 1993). Consistent with this, people with a genotype associated with low levels of MAO-A in an in vitro assay have been associated with greater risk of developing antisocial behavior (Caspi et al. 2002; Kim-Cohen et al. 2006).
Two PET imaging studies of MAO-A in relation to aggressive personality traits show consistent results. The first, an [11C]clorgyline PET study, reported a correlation between reduced MAO-A binding in prefrontal cortex and subregions of the prefrontal cortex such as the orbitofrontal cortex, with self-reported aggression in healthy men (Alia-Klein et al. 2008). The second study, which applied [11C]harmine positron emission tomography with the Revised NEO Personality Inventory, found that prefrontal cortex and subregions of the prefrontal cortex (such as the orbitofrontal cortex) correlated strongly with personality facets angry hostility negatively (r = −0.515, p = 0.001) and deliberation facets positively (r = 0.514, p = 0.001) (Soliman et al. 2011). In a 2-factor regression model, these facets explained 38 % of variance in prefrontal cortex MAO-A binding. In both studies, the MAO-A binding measures among different regions were intercorrelated so the correlations of MAO-A binding with aggression-related measures were also present in all brain regions sampled which included both cortical and subcortical regions.
Currently, this neuroimaging direction is still progressing towards creating impactful information for society. Ideally, future studies should investigate MAO-A binding in samples with pathological levels of aggression. Should it be that reduced MAO-A binding is related to pathological aggression, a longer-term strategy could be to identify whether harmful environmental influences predispose to this low-MAO-A-level phenotype in prefrontal cortex so as to develop novel prevention strategies against harmful neurodevelopment.
25.2 Why Image Indices of Serotonin Transporter Density?
The serotonin transporter (5-HTT) is a 630-amino-acid-long receptor with 12 transmembrane domains (Lesch et al. 1993a, b) and is mainly found in soma, dendrites, and terminals of serotonin-releasing neurons (Zhou et al. 1998; Tao-Cheng and Zhou 1999). Most 5-HTT are located on plasma cell membranes, mainly perisynaptically or along axons (Zhou et al. 1998; Tao-Cheng and Zhou 1999). In human brain, there is wide variation in regional 5-HTT density: superior and inferior raphe nuclei > hypothalamus > thalamus (depending upon nucleus) ≈ amygdala > putamen > caudate ≈ hippocampus > insular cortex > prefrontal cortex > white matter > cerebellar cortex (except vermis) (Cortes et al. 1988; Laruelle et al. 1988; Kish et al. 2005). The serotonin transporter is coupled to sodium, chlorine, and potassium transport (Blakely et al. 1997), but the physiological role of greatest interest of 5-HTT in the brain is its influence upon extracellular serotonin levels. Antidepressants which bind to the serotonin transporter raise extracellular serotonin, and 5-HTT knockout mice have elevated extracellular serotonin, confirming the role of the serotonin transporter in modulating extracellular serotonin levels in vivo (Blier and De Montigny 1983; Bel and Artigas 1992, 1993, 1995; Dreshfield et al. 1996; Moret and Briley 1996; Mathews et al. 2000).
Neuroimaging studies of the 5-HTT offer the opportunity to measure an index of total 5-HTT density, the 5-HTT BPND. This marker is useful for determining therapeutic occupancy of antidepressants as well as several different models of 5-HTT dysregulation in disease. Most disease investigations focus upon potential mechanisms for lowering brain serotonin, and there are at least four models to explain how 5-HTT BPND could be altered in a disease that lowers brain monoamines (Meyer 2007). The first model is a lesion model in which the density of serotonin-releasing neurons is reduced and reductions in 5-HTT BPND occur. The second model is a secondary change in transporter binding consequent to serotonin lowering via a different process. However, available evidence suggests that the different monoamine transporters do not regulate in the same fashion after chronic depletion of their endogenous monoamine. Acute reductions in serotonin lead to reductions in 5-HTT mRNA (Yu et al. 1995), but long-term reductions or elevations in serotonin typically show no effect upon regional 5-HTT density (Graham et al. 1987; Dewar et al. 1992; Benmansour et al. 1999). The third model is increased clearance of extracellular monoamine via greater serotonin transporter density. In the third model, greater total available 5-HTT BPND is interpreted as representative of greater functional 5-HTT density. The fourth model is endogenous displacement and is dependent upon the properties of the radioligand: Endogenous displacement is the property of a few radioligands to express different binding after short-term manipulations of their endogenous neurotransmitter (Paterson et al. 2010). Available evidence suggests that this fourth model is unlikely to be relevant in humans with [11C]DASB (Talbot et al. 2005; Praschak-Rieder et al. 2005), but the issue has not been investigated for other radioligands. Abnormalities in 5-HTT BPND may be discussed in the context of these models.
25.2.1 Radioligands Available for Imaging Serotonin Transporters
There are at least six different radiotracer imaging protocols for imaging serotonin transporters, and the quality of technique varies widely. Originally, the only two radiotracer techniques were 2-beta-carbomethoxy-3-beta-(4-iodophenyl)-tropane (β-CIT) single-photon emission tomography (SPECT) and [11C](+)McN5652 PET, but both of these methods have significant limitations for quantitation (Kuikka et al. 1993). β-CIT has almost equal affinity for the dopamine transporter as compared to the serotonin transporter (Laruelle et al. 1994). As dopamine transporter density is high in the substantia nigra (Ciliax et al. 1999), the relative contributions of dopamine and serotonin transporter binding to the measure of specific binding cannot be separated in the midbrain, the location where this radiotracer technique is applied. The other early radiotracer, [11C](+)McN5652, has a low ratio of specific binding relative to free and nonspecific binding, which, in combination with modest reversibility, makes valid and reliable quantitation difficult in regions other than the thalamus and impossible in human cortex (Kent et al. 2002; Buck et al. 2000).
The radiotracer [11C]DASB (3-amino-4-(2-dimethylaminomethylphenylsulfanyl)-benzonitrile) was a major advance as a result of its selectivity, reversibility, greater specific binding, and reliability (Wilson et al. 2000, 2002; Ginovart et al. 2001; Meyer et al. 2001, 2004a, b; Praschak-Rieder et al. 2005). This radiotracer was three orders of magnitude more selective for the 5-HTT over other monoamine transporters and highly selective for the 5-HTT in comparison to a number of other targets screened (Wilson et al. 2000, 2002). Selectivity was also demonstrated when 94 % of the specific binding was displaceable by 5-HTT binding medications in animal models (Wilson et al. 2000, 2002). [11C]DASB has very good brain uptake in humans (Ginovart et al. 2001). In humans, its ratio of specific binding relative to free and nonspecific is good, and its free and non-specific binding has low between-subject variability (Ginovart et al. 2001; Ichise et al. 2003). Multiple brain regions may be assessed (Wilson et al. 2000, 2002; Ginovart et al. 2001; Meyer et al. 2001, 2004a; Praschak-Rieder et al. 2005), and reliability of regional 5-HTT BPND measures is very good (Meyer et al. 2001, 2004a; Praschak-Rieder et al. 2005). The 5-HTT BPND measures are low in cortex, but with standardized region-of-interest methods, very good reliability of 5-HTT BPND in human cortex is achievable (Meyer et al. 2001, 2004a; Praschak-Rieder et al. 2005). Thus, the discovery of [11C]DASB PET imaging created a new opportunity for validly quantifying 5-HTT binding in humans.
Subsequent to the development of [11C]DASB, several new similarly structured radiotracers were created for imaging techniques of [123I]ADAM SPECT, [11C]MADAM PET, and [11C]HOMADAM PET (see Table 25.2 for a comparison). [123I]ADAM SPECT is superior to β-CIT SPECT; however, [123I]ADAM SPECT is not as useful a technique as the other methods because of its limited specific binding to free and nonspecific binding signal and lack of formal modeling development. [11C]MADAM PET approaches the quality of [11C]DASB PET, but [11C]MADAM has substantially less brain uptake which would be expected to increase the variability of the measurement, especially in regions of lower 5-HTT density (Lundberg et al. 2006). However, it is clear that [11C]MADAM is superior to [11C](+)McN5652 in terms of the reversibility of time-activity curves and specific binding signal relative to free and nonspecific binding signal. [11C]HOMADAM PET shows some advantage over [11C]DASB PET (and the other radiotracers) in that it has a more reversible time-activity curve and a somewhat greater specific binding to free and nonspecific binding signal (Ginovart et al. 2001; Nye et al. 2008), but there are still key areas of development for [11C]HOMADAM PET, including a reliability assessment of binding measures and assessment of its sensitivity to endogenous serotonin. Initial attempts have been made to apply a 18F-labeled derivative of McN5652 in humans (Hesse et al. 2012), but data on test-retest reliability and sensitivity to endogenous 5-HT are still lacking.
Table 25.2
Comparison of PET radiotracers for the serotonin transporter
[123I]β-CIT SPECT | [11C]McN5652 PET | [11C]DASB PET | [123I]ADAM SPECT | [11C]MADAM PET | [11C]HOMADAM PET | |
---|---|---|---|---|---|---|
Selectivity | Highly selective (Jarkas et al. 2005) | |||||
Displaceability of specific binding | Highly displaceable (Jarkas et al. 2005) | |||||
Reversibility (in human imaging) | Adequate in midbrain, good to very good in other regions | Very reversible in all regions (Nye et al. 2008) | ||||
Brain uptake | Good (Lundberg et al. 2006) | Very good (Nye et al. 2008) | ||||
Sensitivity to endogenous serotonin | Not known | Not known | Not known | Not known | Not known | |
Specific binding to free and nonspecific binding ratio | Good | Adequate to very good (Nye et al. 2008) | ||||
Reliability of 5-HTT BP | Not measured | Modest (Kent et al. 2002) | Most regions reasonable (Catafau et al. 2005) | Good to very good (Lundberg et al. 2006) | Not yet reported | |
5-HTT BP measurable in multiple regions? | Yes (Lundberg et al. 2006) | Yes (Nye et al. 2008) |
25.2.2 Developing New Antidepressants with Serotonin Transporter Neuroimaging
In two seminal studies published in 2001 and 2004 applying [11C]DASB PET, it was demonstrated across five different selective serotonin reuptake inhibitors (SSRIs) that after 4 weeks of treatment, for doses which clinically distinguish from placebo, an 80 % occupancy occurs in most brain regions (Meyer et al. 2001, 2004b) (see Fig. 25.5 and 25.6). These SSRIs are defined as such by the property of having at least two orders of magnitude preferential affinity for the serotonin transporter over other target sites. Within the datasets, there was increasing occupancy with increasing dose (and plasma level), with plateauing at the higher doses and higher plasma levels. The dose occupancy relationships consistently demonstrated that the threshold of reaching 80 % occupancy was important. Even though these SSRIs have a 100-fold range in affinity for the serotonin transporter, an 80 % striatal 5-HTT occupancy occurs at minimum clinical dose, thereby demonstrating an additional, important predictive value of the 5-HTT occupancy data in relation to clinical trial development. Furthermore, the added information from occupancy measurement is clear because the in vivo EC50 does not correlate with affinity (Meyer et al. 2004b). This association of 80 % occupancy with doses of SSRI that distinguish from placebo in clinical trial is consistently reported (Kent et al. 2002; Suhara et al. 2003). Hence, while affinity is an essential piece of information regarding an antidepressant, it cannot predict occupancy, even when plasma levels are known (Meyer et al. 2004b). Given the association between the clinically relevant dose and 5-HTT occupancy for SSRIs, it is now generally believed that an 80 % 5-HTT occupancy is a therapeutic threshold for new antidepressants. This technique is frequently applied during phase I trials to assess whether new antidepressants in development are adequately brain penetrant and to guide dosing selection for subsequent phase II clinical trials.
