Assessment of the hepatic vasculature is essential for tumor staging, surgical planning, and understanding of liver disease. Technological advances have made contrast-enhanced magnetic resonance (MR) imaging comparable to multidetector-row computed tomography for diagnostic vascular imaging with respect to spatial resolution. Unenhanced MR angiographic sequences enable reasonable clinical assessment of vessels without contrast agents in patients with contraindications or renal insufficiency. Furthermore, MR angiography may be used to provide directional information through manipulation of the signal intensity of flowing blood. A major limitation to consistent contrast-enhanced MR angiography is the timing of MR image acquisition with arrival of the contrast bolus in the structures of interest. In this article, the authors discuss currently available techniques for imaging of the hepatic vasculature.
Routine hepatic magnetic resonance (MR) imaging provides an excellent assessment of the hepatic vasculature. Automated contrast detection methods in combination with fast breath-hold sequences allow reproducible capture of the arterial, portal venous, and delayed phases of enhancement. This reproducible capture enables improved vascular lesion detection and characterization. Sequences can also be dedicated to focus on specific aspects of the hepatic blood vessels, including the demonstration of flow physiology. High-resolution, 3-dimensional, gadolinium-enhanced imaging, including fat suppression and multiphasic reconstructions, can be used routinely without exposing patients to ionizing radiation. MR imaging is able to provide an accurate assessment of the hepatic vascular anatomy. This accurate assessment is essential for patients who are being considered for surgery, such as liver resection or transplantation. In addition, MR imaging can also provide a road map to guide radiological intervention, which can reduce procedural times and improve safety ( Fig. 1 ). For diagnostic evaluation, relatively noninvasive techniques such as MR angiography and computed tomography (CT) angiography have largely replaced digital subtraction angiography (DSA).
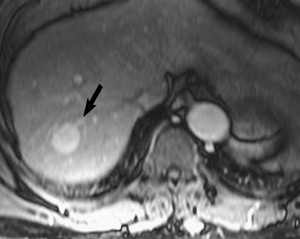
Multidetector row CT (MDCT) angiography provides excellent detail of the hepatic arteries and their branches and is a robust, quick method for vascular assessment before surgery. MDCT also has the advantage of being readily accessible in most institutions. However, a significant advantage of contrast-enhanced MR angiography is the ability to assess the liver without irradiation. Both CT and MR imaging contrast agents have some associated risk of contrast-induced nephropathy as well as other adverse effects, including rare but potentially lethal reactions such as anaphylactic shock. More recently, there has been increased awareness of nephrogenic systemic fibrosis as a potential complication in patients with severe chronic renal insufficiency or acute renal failure who undergo gadolinium-enhanced MR imaging. A further advantage of MR imaging compared with MDCT is the ability to selectively visualize the hepatic vasculature using noncontrast-enhanced MR angiography. Doppler ultrasonography also allows for such noninvasive imaging of vessels without ionizing radiation or contrast agents, but visualization may be limited for various reasons such as overlying bowel gas or large body habitus.
Historically, an important limitation of MR angiography compared with DSA and CT angiography has been lower spatial resolution. However, there have been recent significant improvements in the achievable spatial resolution owing to the introduction of newer image reconstruction algorithms and dedicated contrast agents. These developments have enabled MR angiography to achieve higher spatial resolution and better image quality. The combination of multichannel, high field-strength MR systems, and the development of multielement angiography receiver coils has also helped to substantially improve the clinical utility of MR angiography.
Hepatic arteries
Contrast-Enhanced MR Angiography
Contrast-enhanced MR angiography is the preferred technique for MR imaging of the arterial vasculature. Contrast-enhanced MR angiography relies on timing scan acquisitions after contrast administration such that T1 shortening is maximal during the period when the center of k-space is acquired. Injection of conventional gadolinium contrast agents results in only a transient T1 shortening of the intra-arterial blood during which time data must be acquired, necessitating accurate timing of the scan with the injection of contrast material. Most routine MR imaging contrast agents rapidly diffuse from the vessels into the extracellular space. The intravascular half-life of such extracellular gadolinium-based contrast agents is approximately 90 seconds. Consequently, to optimize results, a sufficient volume of contrast needs to be injected in a short period to decrease the T1 of blood to values substantially less than those of adjacent tissues.
Until recent developments, breath-hold scan times exceeding 30 seconds were often required for standard 3-dimensional gradient-recalled echo (GRE) sequences. Such prolonged breath-hold times limit the quality of images obtainable in debilitated patients who have compromised respiratory function and are not capable of the multiple breath-holds required for dynamic GRE MR imaging. Several approaches have been developed to reduce the breath-hold times in dynamic GRE MR imaging, such as decreasing the matrix size and the number of partitions. However, the introduction of such parameter changes to reduce acquisition time results in trade-offs including reduced spatial resolution or limited z-axis coverage.
Parallel Imaging Techniques
Another approach that is presently applied to reduce breath-hold times is to use parallel imaging techniques. These methods use multiple radiofrequency receiver coils to acquire multiple data points simultaneously within a single phase-encoding gradient. In this approach, k-space is systematically undersampled by each coil, which conventionally would lead to aliasing or wraparound artifacts. However, parallel imaging uses the redundant spatial information available from the multicoil configuration to restore the spatial resolution and avoid the aliasing artifact. Techniques that have been developed to correct for aliasing vary, depending on whether they work on image domain data, such as the sensitivity encoding method, or on the raw (k-space) data, such as the GeneRalized Autocalibrating Partially Parallel Acquisitions method.
With parallel imaging, the maximum increase in speed is proportional to the number of coils. However, the signal-to-noise ratio (SNR) is reduced by shorter acquisition times and is further decreased by the amplification of noise that occurs in regions in which the geometry of coil sensitivities is suboptimal. This reduction in SNR can be partially overcome by faster rates of injection of the contrast bolus to achieve higher contrast concentrations during sampling.
Shortened Scan Times
Advances in gradient hardware, pulse sequences, and computational power have allowed for greatly shortened scan times. By shortening the scan time to several seconds, it is possible to image arterial, portal, and venous contrast phases during individual breath-holds. The resultant images provide dynamic information on contrast arrival and rates of enhancement of vascular structures and organs. Regardless of the technique used, there is always a trade-off between spatial resolution and temporal resolution. Investigators have compared the diagnostic accuracy of dynamic parallel GRE MR imaging with contrast-enhanced 64-detector MDCT, which served as the noninvasive reference standard. Comprehensive hepatic vascular mapping of the hepatic arteries and the portal and hepatic veins was included. Image quality for depiction of most of the hepatic vessels was rated as high with the use of parallel GRE MR techniques. Furthermore, image quality was rated as good or excellent for 91.1% to 100% of vessels. The readers for this study were able to confidently diagnose variant hepatic vessels and arterial stenosis with 94% to 100% accuracy ( Fig. 2 ). All portal and hepatic veins were visible for diagnostic purposes, and image quality was considered to be nondiagnostic in only 2.1% of the hepatic arteries evaluated.
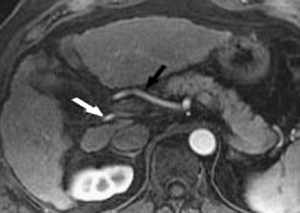
Noncontrast MR Angiography Techniques
It is technically feasible to image the hepatic arteries using specific sequences without the administration of MR contrast agents. The noncontrast technique, which is likely to be most effective in evaluating the hepatic arteries, is the respiratory-triggered, 3-dimensional, steady-state free-precession (SSFP) sequence, which is a fast MR technique that has been widely used in coronary MR angiography. SSFP can be used to rapidly produce images of acceptable resolution, and when time spatial labeling inversion pulse (T-SLIP) is combined with SSFP there is further improvement in the visualization of the hepatic arteries. The T-SLIP sequence is a form of spin labeling that can provide quantitative and selective inflow information by placing the inversion pulse before data acquisition and suppressing the background tissue. The time-of-flight (TOF) technique, which can also be used to visualize vessel, is likely to be less effective because of in-plane saturation effects. The SSFP sequence is a type of gradient-echo sequence applied with very short echo times, which allows imaging of blood vessels without the use of a contrast agent. SSFP depends on the ratio of T2 to T1. This ratio is relatively high for blood compared with the surrounding tissues and the short echo time renders the sequence flow insensitive. T-SLIP is a type of arterial spin labeling technique, which can be used to further enhance the signal from the selected vessel in combination with SSFP by signal suppression of the background tissue and other vessels.
These techniques are presently limited for routine clinical use because of the long acquisition times of up to 10 minutes. New methods to shorten acquisition times include the combination of this technique with parallel imaging and using techniques to counteract the reduction in signal that would occur. The combination of SSFP with short-tau inversion recovery (STIR) and T-SLIP enables greater background signal suppression to compensate for the signal reduction in the blood vessel induced by 2-dimensional parallel imaging. The combination of 2-dimensional-PI, T-SLIP, and STIR has been shown to successfully shorten the acquisition time from 10 minutes to 6 minutes while allowing for selective hepatic artery visualization and maintaining diagnostic image quality.
Portal vein
Contrast-Enhanced MR Portography
Several modalities are available to image the portal veins, and each modality has its strengths and limitations. Doppler ultrasonography (DUS) is particularly useful, as it is noninvasive, inexpensive, and provides information regarding portal flow. However, DUS is highly operator and subject dependent and, therefore, may be adversely affected by factors such as patient obesity, intestinal gas, and excessive abdominal ascites.
Any technique used to image the portal vein must be able to provide an accurate assessment of portal venous anatomy, which is essential for patients being evaluated for liver resection or transplantation. Studies have demonstrated the superiority of 3-dimensional MR portography over DUS. The normal portal vein bifurcates into right and left branches in 89% of individuals. The right portal vein divides into anterior and posterior branches, whereas the left divides into superior and inferior branches. Common anatomic variants are trifurcation of the main portal vein, in which the left portal vein arises at the bifurcation of the right portal vein. Other common variants are a left portal vein origin from the right anterior portal vein branch or an early origin of the right posterior portal vein branch seen in 4% and 6% of individuals, respectively. Unfavorable congenital portal venous anomalies may preclude individuals from liver donation. It is also essential to evaluate the size and patency of the recipient portal vein prior to liver transplantation because the donor portal vein should optimally be no more than 3 to 4 mm different in size relative to that of the recipient’s portal vein.
Portal Vein Thrombosis
Portal vein thrombosis is another important clinical indication for portal venous evaluation. The diagnostic sensitivity of DUS for detecting portal vein thrombosis is reported to range from 66% to 100%. Portal vein thrombosis may be associated with a variety of different disease processes such as severe intra-abdominal infections, hypercoagulable states, and various malignancies. Portal vein thrombosis may also be a complication of pregnancy, oral contraceptive use, or trauma. In such cases, portal vein thrombi appear as well-defined, nonenhancing, intraluminal filling defects on contrast-enhanced MR imaging, and may be associated with transient hepatic intensity differences caused by compensatory increased arterial flow to the thrombosed hepatic segments. Distinction between acute and chronic portal vein thrombosis is often of clinical value. In the acute setting, portal vein thrombus may resolve with anticoagulation therapy. However, once the occlusion is long-standing, the likelihood of therapeutic recanalization is greatly reduced. Acute thrombi may show increased signal on T2-weighted sequences relative to chronic thrombi. Chronic portal venous occlusion leads to cavernous transformation of the portal vein, which may preclude future transplantation or liver resections.
Although bland thrombus is the most common filling defect to be seen within the portal vein, it is prudent to consider alternative diagnoses. In particular thrombophlebitis, which is caused by infected venous clot, should be excluded because treatment requires antibiotics in addition to anticoagulation. At MR imaging, thrombophlebitis may be suggested when periportal edema, venous mural thickening, and venous mural enhancement is seen in a febrile patient or patient with sepsis. Another critical diagnosis to consider is tumor thrombus, which typically presents as portal vein expansion with enhancing tissue. The most common malignancy to present with portal venous tumor thrombus is hepatocellular carcinoma, which frequently exhibits direct tumor extension into the portal vein ( Fig. 3 ). The enhancement of tumor thrombus may be subtle, and for such cases subtraction images may be of benefit. A notable pitfall is that tumor thrombus may show increased T2 signal, much like an acute thrombus, and so evaluation for enhancement, which is seen only with tumor thrombus, is important.
Portal Vein Angiography Advantages
MR portography (portal vein angiography) compares favorably to other imaging modalities using contrast agents for the diagnosis of portal vein thrombus. Kreft and colleagues described an overall sensitivity, specificity, and accuracy for the detection of thrombosis of 100%, 98%, and 99%, respectively, for 3-dimensional gadolinium-enhanced MR angiography and 91%, 100%, and 96%, respectively, for standard intra-arterial DSA. A 3-dimensional gadolinium-enhanced MR angiography also shows an efficacy similar to a 64-slice MDCT for portal vein thrombus detection, and is able to identify portal vein anatomic variants with sensitivities and specificities of 100%. In this regard, MR imaging has a clear advantage when compared with MDCT or catheter-based angiography regarding concerns of irradiation and, in patients without renal insufficiency, the safety profile of the contrast agent.
Noncontrast MR Imaging of the Portal Vein
Gadolinium-based contrast agents are clinically contraindicated in some patients. In such patients, noncontrast-enhanced MR imaging techniques may help to provide useful diagnostic information. There are other drawbacks to the use of contrast agents, which can be overcome by noncontrast MR angiography techniques. For example, it is occasionally difficult to differentiate the portal vein and the surrounding liver parenchyma during the portal venous phase of enhancement because of insufficient difference in signal. Furthermore, mistiming of image acquisition may result in suboptimal venous opacification relative to surrounding structures.
TOF MR angiography is a well-established, noncontrast technique that can be used to assess patency as well as the direction of flow in the portal vein. TOF MR angiography produces saturation of stationary tissue by multiple repetitive radiofrequency pulses. Nonsaturated blood entering the imaging section is of higher signal than the surrounding saturated tissue. Imaging data can then be acquired as multiple overlapping sections that can be reconstructed to create angiographic images. Presaturation bands can be used to eliminate arterial signal and also provide information on whether flow is hepatopedal or hepatofugal.
Patent blood vessels show high signal intensity on SSFP imaging, whereas thrombus is usually seen as a lower signal-filling defect within vessel. Although slow-moving blood may occasionally result in ambiguous findings, SSFP may be repeated several times until a satisfactory image is obtained. Therefore, SSFP imaging may provide helpful assessment of the portal vein in debilitated patients or those with poor venous access. In a study by Smith and colleagues, SSFP imaging allowed correct diagnosis in 17 out of 17 patients as negative for portal vein thrombosis, achieving a specificity of 100%. In this study 4 of 6 patients were also found to be positive for portal vein thrombosis using SSFP imaging, and the diagnosis was inconclusive in 3 patients, of whom 2 were eventually found to be positive for portal vein thrombosis while 1 had portal vein stenosis. Thus, the sensitivity of SSFP for portal vein thrombus detection was only 67% when compared with contrast-enhanced MR imaging of the portal vein. An important factor to remember is that overlapping hepatic arteries and veins may obscure the portal vein; therefore, the combination of T-SLIP with SSFP is recommended for selective suppression of signal from blood vessels that are of no clinical interest. Furthermore, more than one T-SLIP can be placed in the imaging region, to enable selective visualization of the vessel of interest. Another potential disadvantage of SSFP for evaluation of the portal vein is the insensitivity of SSFP to the direction of blood flow. If the direction of flow is of clinical relevance, TOF or phase contrast (PC) techniques can be used to image the portal veins.
Half-Fourier fast spin echo (FSE) is a newer, T2-weighted, unenhanced MR angiography technique that has potential clinical applications. Half-Fourier FSE allows for a coronal acquisition to enable shorter 3-dimensional acquisition times. The T2-weighting results in relatively low background liver parenchymal signal intensity, resulting in greater portal vein conspicuity. Selective visualization of the portal vein with half-Fourier FSE may also require the addition of T-SLIP to improve results.
Portal Blood Flow Evaluation
The 2 main noninvasive methods for the quantitative assessment of hepatic blood flow are duplex DUS and MR imaging. Regarding MR imaging techniques, PC imaging is ideally suited for flow quantification. PC sequences use the intrinsic ability of MR imaging to detect tissue displacement. Whereas conventional MR imaging sequences represent only the combined magnetization vector amplitude, PC also computes the phase shift of this vector induced by flow direction and velocity. Examples of clinical scenarios in which PC techniques may be helpful include the quantification of increases in portal flow after transhepatic shunting or the noninvasive calculation of total hepatic blood flow rates. Hepatic artery flow rate measurements can also be deduced by subtraction of the portal blood flow rate from that of the hepatic venous flow rate. An alternative method to measure portal venous and hepatic arterial fraction flow is by the use of dynamic contrast-enhanced MR imaging with intravenous gadolinium boluses. Such methods have shown promise for the assessment of diffuse liver disease, in which the relative portal venous flow is reduced compared with control patients.
Hepatic Veins
It is important to assess for anatomic variants of the donor hepatic veins before liver transplantation, as well as for patients who are to undergo partial hepatectomy. The hepatic veins in most individuals are usually composed of a right, middle, and left main hepatic veins. Common anatomic variants are accessory hepatic veins draining segments V and VIII in 43% and 49%, respectively. Another common anatomic variant is a hepatic vein-draining segment VI directly into the inferior vena cava (IVC). The identification of these and other anatomic variants is critical prior to hepatic surgery. MR imaging is able to provide excellent evaluation of the hepatic anatomy for this purpose ( Fig. 4 ).
