1. Dementia with grains: DG
2. Senile dementia of the neurofibrillary tangle type (SD-NFT)
3. Progressive supranuclear palsy: PSP
4. Corticobasal degeneration: CBS
5. Pick disease
6. Diffuse neurofibrillary tangle with calcification: DNTC
7. Globular glial tauopathies: GGT
Of the 1,212 patients (689 males and 523 females aged 31–104 years) examined by serial autopsy between August 1999 and May 2012 at the Brain Bank in the Tokyo Metropolitan Medical Center of Gerontology and Institute of Gerontology, AD was observed in 19%, DG and NFTD which are senile tauopathies were observed in 18% each, followed by DLB in the group with neurodegenerative dementia with a clinical dementia ratio (CDR) of 0.5. Furthermore, dementia with senile tauopathy in the background including that present as a complication was detected in the majority (57%) of patients (Fig. 4.1) [4, 5]. Among those with a CDR of 1 or higher, the diagnosis was AD in 35% and DLB in 19%, while senile tauopathy including that exhibiting complex pathological features was noted in 33%. These findings indicated that senile tauopathy is important as the background pathology of dementia in older people and needs to be considered in clinical and imaging diagnoses.
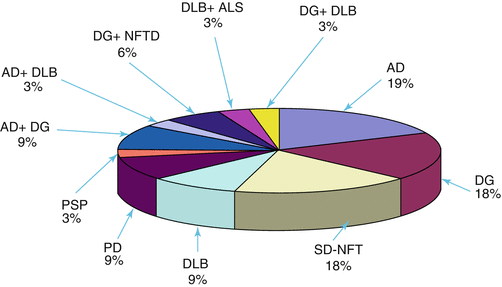
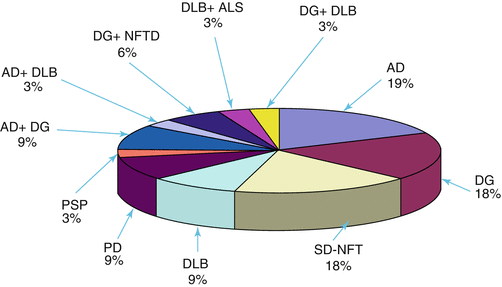
Fig. 4.1
CDR = 0.5 Background pathology of degenerative dementia. AD Alzheimer’s disease, DG Dementia with grains, SD-NFT Senile dementia of the neurofibrillary tangle type, DLB dementia with Lewy body, PD Parkinson’s disease, PSP Progressive supranuclear palsy, ALS Amyotrophic lateral sclerosis. (From [5] with permission)
4.2 Dementia with Grains
4.2.1 Disease Concept
Dementia with grains (DG) is a late-onset dementia morphologically characterized by the presence of abundant spindle-shaped argyrophilic grains in neuronal processes and coiled bodies in oligodendrocytes proposed by Braak and Saito et al. [1–4]. It is an important disease to be considered, in addition to AD and DLB, as a causative disorder for senile dementia (Table 4.1, Fig. 4.1). Neuropathologically, the disease is characterized by the deposition of argyrophilic grains, which are granules that are stained by the Gallyas–Braak silver impregnation method. Deposits first appear in the ambient gyrus, and pathological changes gradually spread to the cortex of the frontal and temporal lobes. Neuropathological staging of the disease into I–III according to the area of pathological changes has been proposed by Saito et al., with dementia occurring in stage III [4]. Figure 4.2 shows the progression of pathological changes in DG. The affected region is close to the areas in which early pathological changes have been reported to appear in AD and, with progression of the disease, overlaps the areas affected in AD.
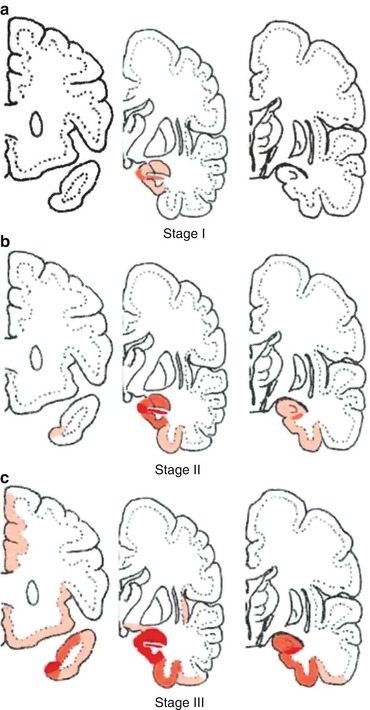
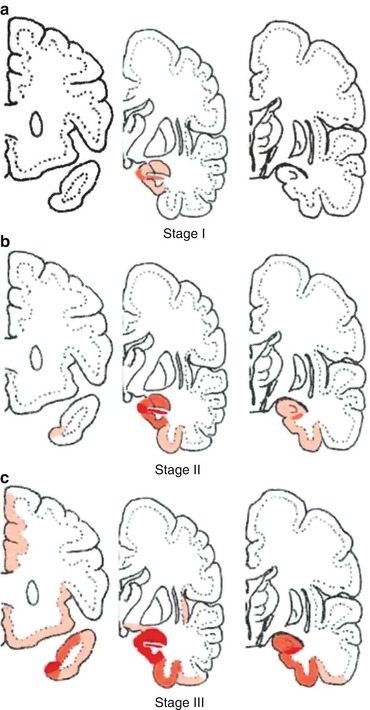
Fig. 4.2
Neuropathological staging of DG [2]. The topographical distribution of argyrophilic grains (AGs) in each grain stage. Three coronal sections through the genu of corpus callosum, the mammillary body, and the lateral geniculate body. Stage I: AGs are localized to the ambient gyrus, the anterior CA1 of hippocampus, the anterior entorhinal area, and the amygdala. Stage II: AGs are more apparent in the medial temporal pole, in the posterior subiculum, and the entorhinal and transentorhinal cortex. Stage III: AGs involve the anterior cingulate gyrus, septum, accumbens, gyri recti, insular cortex, and hypothalamus in addition to the medial temporal lobe. It is highly probable that the pathology in argyrophilic grain stage III contributed to cognitive decline. (From [2] with permission)
4.2.2 Problems with a Clinical Diagnosis
Clinical diagnostic criteria for dementia with grains (DG) have not yet been established. Therefore, DG is diagnosed as diseases with another background pathology including vascular dementia and AD in many patients. The establishment of clinical diagnostic criteria based on the background pathology is considered to lead to appropriate treatments, care, and nursing [4–7].
An analysis of the history of DG definitively diagnosed with neuropathological evidence indicates the following as characteristics of the disease: (1) slow progression, (2) symptoms suggestive of frontotemporal dementia such as personality changes, irritability, and poor personal contact, (3) forgetfulness as a frequent initial symptom, and (4) the older age of patients than those with other degenerative dementias [5–7]. The frequent complications of DG by diseases including AD, SD-NFT, and vascular dementia in older individuals also make its clinical diagnosis difficult. Therefore, although clinical diagnostic criteria for DG are difficult to formulate, their establishment by relating clinical, imaging, and pathological findings in this important disease, which was recognized due to neuropathological evidence, is an urgent requirement.
The development of radical treatments for AD such as Aβ-vaccine therapy based on the amyloid cascade hypothesis, tau targeting drug, and the development of secretase inhibitors further increases the necessity of an accurate diagnosis of mild cognitive impairment (MCI), which is attracting attention as a precursor of AD. Radical treatments for AD are not expected to be effective for DG without β-amyloid deposition, and an accurate diagnosis in the stage of MCI or even earlier is essential. The clinical symptoms of DG overlap those described in the international diagnostic criteria for AD, and difficulties are also associated with their morphological differentiation. The development of evaluation methods that integrate neuroimaging findings as objective data in addition to clinical and neuropsychiatric diagnoses is awaited.
4.2.3 MRI Findings in DG
In typical DG, MRI shows atrophy near the ambient gyrus and uncus [5, 6] (Figs. 4.2, 4.3, 4.4, and 4.5). Asymmetric atrophy is shown in many cases of DG; however, there are also some cases that the difference between right and left is not clear. By the progress of the stage of dementia, atrophic area progresses extensively toward the temporal and frontal lobe widely.
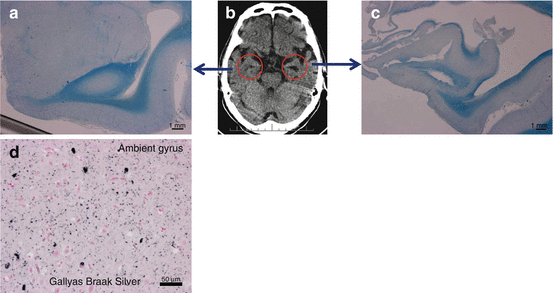
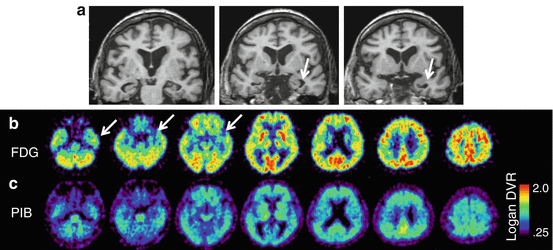
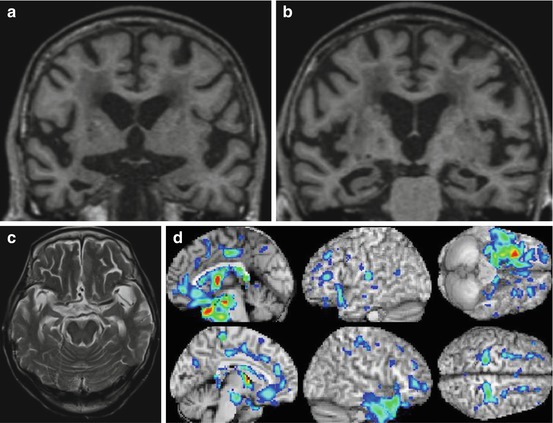
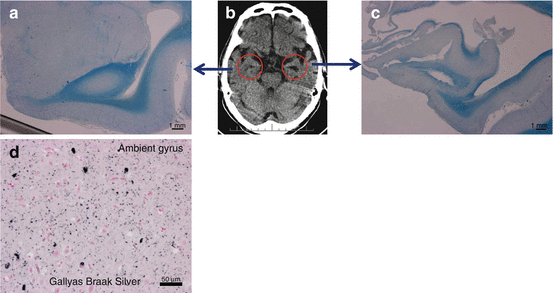
Fig. 4.3
90s male, CDR = 1 Dementia case. [5] (a) (right side medial temporal lobe): Microscopic specimen (KB stain) shows no obvious atrophy. (b) Brain CT shows dilatation of left interior horn of the lateral ventricle suggesting atrophy of the medial temporal lobes including the ambient and parahippocampal gyri (c) (left side medial temporal lobe): Microscopic specimen (KB stain) shows atrophy in the amygdala, anterior side of hippocampus, ambient gyrus to entorhinal cortex. (d) A large number of Gallyas–Braak argyrophilic grains exist in the left medial temporal lobe especially in the ambient gyrus. (From [5] with permission)
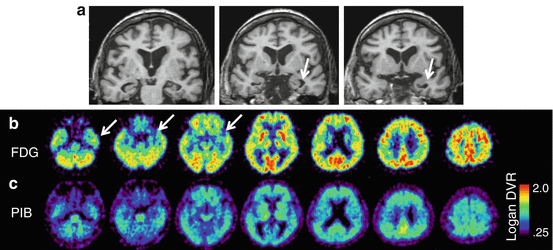
Fig. 4.4
70s female. (a) MRI 3D T1-weighted image shows dilatation of left inferior horn of the lateral ventricle (white arrows) suggesting left dominant atrophy of anteromedial temporal lobe. (b) FDG-PET shows left dominant decrease of glucose metabolism in the frontotemporal lobe (white arrows). (c) 11C PIB-PET study was negative suggesting no accumulation of Aβ in this patient. (From [5] with permission) Courtesy of Dr. Kenji Ishii
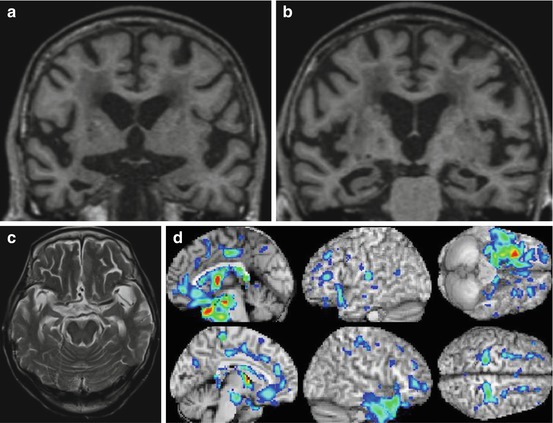
Fig. 4.5
Dementia with grains, MRI of an 80-year-old female patient with pathologically proven dementia with grains. She had a six-year history of cognitive impairment with an antemortem diagnosis of Alzheimer’s disease. (a, b) Coronal three-dimensional (3D) T1-weighted images reveal the right dominant asymmetric atrophy of the medial temporal lobes including the ambient and parahippocampal gyri. (c) Additionally, T2-weighted image reveals right dominant bilateral atrophy of the amygdala. (d) Voxel-based morphometry on Voxel-based Specific Regional analysis system for Alzheimer’s disease (VSRAD) software can objectively display this right dominant asymmetric atrophy of the temporal lobes and limbic system
The structures of the medial temporal lobe near the parahippocampal gyrus, such as the entorhinal cortex and ambient gyrus, in which the earliest changes occur, need to be examined in MRI of the early stage of AD and DG; however, these structures are extremely small, and a visual evaluation of atrophy in such small structures is difficult. MRI has many advantages, the greatest of which are its non-invasiveness and repeatability. Since objectivity is insufficient by visual evaluations alone, a statistical image analysis was recently introduced to overcome this limitation. In the diagnosis of dementia, the usefulness of objective evaluations of the gray matter volume determined by voxel-based morphometry (VBM) as a statistical image analysis technique has also been reported. In the future, problems such as the differentiation of DG from other degenerative dementias including AD by a statistical assessment of ambient gyrus atrophy, which is an initial pathological change in DG, in patients with a confirmed background pathology, the setting of a region of interest for the extraction of DG, and the development of software for the differentiation of DG from AD need to be addressed. In the patient shown in Fig. 4.2, neuropathological findings show clear asymmetry in atrophy of the medial temporal lobe, reflecting CT findings. A large number of Gallyas–Braak argyrophilic grains exist in this site, and the diagnosis was confirmed to be DG. However, the ambient gyrus, which shows deposits of argyrophilic grains and atrophy in the early stage of the disease, is very close to the entorhinal cortex, which is atrophied in an early stage of AD, and the degree of atrophy may also be asymmetric in AD, making differentiation difficult.
An evaluation using multiple modalities in combination, particularly amyloid imaging using PIB [8, 9] which is considered to reflect the accumulation of β-amyloid, is regarded as a useful tool for differentiating between AD and DG. In the patient shown in Fig. 4.4, atrophy of the parahippocampal gyrus was clear, whereas the accumulation of PIB was absent; therefore, it was necessary to suspect a pathological condition different from AD. If, with the progression of a clinical assessment of PET preparations including PIB for the labeling of β-amyloid proteins, which constitute a core pathological feature of AD, reflection of the amyloid accumulation process in images becomes clear, expectations for the use of amyloid imaging to monitor treatment and care as well as early diagnoses will grow. Furthermore, the development of PET preparations that specifically label phosphorylated tau is in progress, and the possibility of a specific diagnosis of tauopathy is broadening.
While this remains controversial, a characteristic of DG observed on FDG-PET is reduced glucose metabolism on the medial temporal lobe. The area that shows this change is relatively limited, and metabolism in the lateral temporal lobe and parietal lobe is typically intact relative to the disease stage. In AD, pathological changes first appear in the posterior cingulate gyrus and precuneus and then spread to the parietal, lateral, and medial temporal and frontal lobes in typical cases. Furthermore, the pattern of progression may differ from that of the decline in metabolism.
On brain perfusion SPECT, a typical pattern in AD is also decreases starting in the parietal lobe and temporal association cortex that spread to the frontal association cortex. The usefulness of brain perfusion SPECT for the diagnosis of DG has not been established; however, a decrease in cerebral blood flow is observed in the medial temporal lobe, and the spread of areas with reduced blood flow are limited for the disease stage. The techniques of a statistical image analysis have been adopted in brain perfusion SPECT without relying on visual evaluations or the highly arbitrary setting of the region of interest. In three-dimensional stereotactic surface projection (3D-SSP), the results of analyses are projected on the brain surface. Using the easy Z-score Imaging System developed by Matsuda et al., 3-D images of a brain phantom are obtained with SPECT systems at various facilities, differences among the systems are corrected, and a database of normal images is shared among facilities [10–12].
4.3 Senile Dementia of The Neurofibrillary Tangle Type (SD-NFT)
4.3.1 Disease Concept
SD-NFT is a new type of dementia proposed by Yamada et al. on the basis of recent neuropathological findings [13–16]. It is an important disease to be considered, in addition to AD and DLB, as a causative disorder of senile dementia (Table 4.1). Neuropathologically, the disease is characterized by the deposition of numerous NFT in the parahippocampal region with the absence or scarcity of senile plaque.
It was proposed that SD-NFT be termed primary age-related tauopathy (PART) in 2014 [17], but an opposing argument was put forward in 2015 that PART is just a subtype of AD [18]. Establishment of its disease concept by accumulating knowledge in various aspects of neuropathology, biochemistry, neuroimaging, and clinical diagnosis is desired. To clarify the disease concept of SD-NFT, knowledge based on the currently established background pathology is described in this report.
4.3.2 Clinical Diagnosis
The clinical characteristics are as follows: (1) it develops with memory disturbance at an elderly age, (2) shows slow progression, (3) atrophy of the hippocampal region is observed, but diffuse cerebral atrophy is mild, and (4) it can be differentiated from Alzheimer’s disease and other degenerative dementias [13–15].
4.3.3 MRI Findings
There is no well-organized report on the characteristics of imaging diagnosis, but it is important to accumulate slowly progressing dementia cases in the advanced elderly, taking the possibility of differentiation from Alzheimer’s disease and argyrophilic grain dementia into consideration [19], to develop fundamental therapeutic agents, appropriately proceed with clinical trials, and plan precise treatment, care, and nursing plans.
Figure 4.6 shows a female in her 90s who developed dementia with forgetfulness in her 80s, and dementia relatively slowly progressed. An MR image with an MMSE score of 23 at 6 years after the onset is presented. Amygdala atrophy with laterality, such as that observed in DG, could not be confirmed, but atrophy extending to the posterior hippocampus was suggested by visual examination and voxel-based morphometry on Voxel-based Specific Regional analysis system for Alzheimer’s disease (VSRAD) software [20]. Uptake was negative on amyloid imaging, based on which SD-NFT was considered.
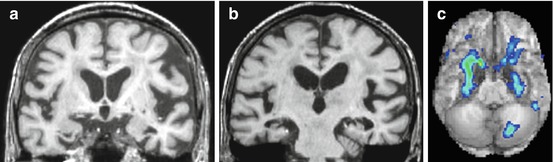
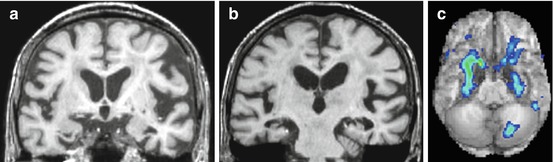
Fig. 4.6
Senile dementia of the neurofibrillary tangle type (tangle only dementia): SD-NFT. MRI of an 80-year-old female in her 90s who developed dementia with forgetfulness in her 80s, and dementia relatively slowly progressed. An MR image with an MMSE score of 23 at 6 years after the onset is presented. (a, b) Coronal three-dimensional (3D) T1-weighted images reveal atrophy of the hippocampus. Severe amygdala atrophy with laterality, such as that observed in DG, could not be confirmed. (From [79] with permission). (c) Voxel-based morphometry on Voxel-based Specific Regional analysis system for Alzheimer’s disease (VSRAD) software can objectively display atrophy of the hippocampus extending to the posterior hippocampus
In SD-NFT, neurofibrillary tangles are widely observed in the parahippocampal gyrus and hippocampus and accompanied by neuronal loss and gliosis. Morphological changes correspond to these neuropathological changes, and atrophy of the parahippocampal gyrus and hippocampal region is observed [13–15]. These morphological changes require differentiation from AD and DG. Atrophy extending to the posterior hippocampus is observed in SD-NFT, in contrast to DG in which atrophy starts in the ambient gyrus and is noticeable on the ventral side of the medial temporal lobe.
Progression of whole-brain atrophy with the disease stage is clear in AD, whereas extensive cerebral cortical atrophy in SD-NFT has been suggested to be mild. When the progression of cognitive disorder is very mild despite morphological atrophy progressing, it is necessary to consider the differentiation of SD-NFT and DG. Since atrophy extending to the posterior hippocampus is present from an early stage, unlike DG, differentiation from AD by visual assessment of MRI alone is difficult at present. The grade of whole-brain atrophy following the disease stage is suggested to be milder than that in AD.
There is no organized report on cerebral blood flow SPECT. Cases showing reduced blood flow localized in the medial temporal lobe and those showing a pattern similar to that observed in Alzheimer’s disease have been suggested. Negativity on amyloid imaging using PIB, which reflects Aβ accumulation, and the development and application of a PET preparation specifically labeling phosphorylated tau may expand the possibility of the specific diagnosis of tauopathy and become a useful tool to differentiate between AD and DG and SD-NFT. Accurate early diagnosis is essential for early and preclinical diagnoses and clinical trials of fundamental therapeutic agents, for which the preparation of diagnostic criteria by accumulating background pathology-based clinical and imaging courses and findings is urgently needed [14, 15].
Although it is hard to say that the evaluation has been established, the characteristic of argyrophilic grain dementia on FDG-PET is reduced glucose metabolism in the medial temporal lobe. It is relatively localized, and metabolism is retained in the lateral temporal lobe and parietal lobe compared with the disease stage progression. SD-NFT has also been suggested to show a tendency similar to DG or be difficult to differentiate from AD in some cases. The accumulation of background pathology-based findings is necessary. The presence of a concomitant pathology of AD, DG, or DLB in SF-NFT has also been reported [4, 16]. It is necessary to be aware of the presence of a complex background pathology at a high rate in advanced elderly patients with dementia.
4.4 Progressive Supranuclear Palsy
4.4.1 Disease Concept
Progressive supranuclear palsy (PSP) is a neurodegenerative disease established as a disease entity by Steel et al., who reported 9 patients including autopsied cases in 1964 [21]. It is a type of tauopathy that exhibits atrophy of the midbrain and superior cerebellar peduncle, depigmentation of the substantia nigra and coeruleus nucleus, and pathological changes in wide areas including the globus pallidus, subthalamic nucleus, and dentate nucleus of the cerebellum, and is histologically characterized by tufted astrocytes.
4.4.2 Clinical Profile
The disease occurs after the age of 40, progresses slowly, and is characterized by supranuclear vertical gaze palsy, frequent falling due to impaired postural maintenance, Parkinsonism, and gait disorders from early after its onset [22].
In studies investigating clinical symptoms of PSP definitely diagnosed pathologically, clinical symptoms suggesting dementia were noted at a high rate, such as changes in personality/frontal sign, psychological symptoms, and memory impairment/cognitive disorder, being present in 33, 18, and 32%, respectively, showing that PSP is an important background disease of cognitive impairment [23, 24]. Since head injury caused by falling affects the prognosis, and an accurate imaging-based diagnosis directly facilitates precise assistance, nursing, and medical care.
Clinical and pathological diversities of PSP have recently been clarified. Classic PSP showing a clinical course of early postural instability, falls, and supranuclear gaze palsy, so-called Richardson’s syndrome (PSP-RS) reported by Richardson et al. [21], accounts for about 50% of all cases. In addition, the presence of PSP-subtypes showing diverse clinical and pathological spectra [25], such as PSP-parkinsonism (PSP-P) difficult to differentiate from Parkinson’s disease (PD), pure akinesia, PSP-pure akinesia with gate freezing (PSP-PAGF) preceded by gate freezing, PSP-primary non-fluent aphasia (PSP-PNFA) developing non-fluent aphasia, PSP-corticobasal syndrome (PSP-CBS) developing asymmetric cortical symptoms, and rare cases of PSP with cerebellar ataxia (PSP-C) in which cerebellar symptoms precede, has been clarified, and imaging findings reflect each subtype.
Clinicopathologically, the pathology of PSP overlaps with those of AD, PD, and DLB, and it is difficult to make clinical and neuroimaging diagnoses of patients with these complications. The suggestion of the presence of an overlapping background pathology while carefully observing changes in the medical history and imaging findings of individual cases is an important role of neuroradiologists.
4.4.3 MRI Findings
4.4.3.1 PSP-RS
Structural MRI findings are useful to diagnose PSP-RS. Examination of the sagittal view is essential, and the characteristic midbrain atrophy with relative preservation of the pons on a sagittal image, termed a “hummingbird sign” or “penguin-silhouette sign,” is the most important finding [26, 27] (Figs. 4.7 and 4.8). The axial image also reflects midbrain atrophy, and reduction of the anteroposterior diameter of the midbrain at the superior colliculi level is observed referred as “Morning glory sign” or “Mickey Mouse sign” (Fig. 4.7) [28].
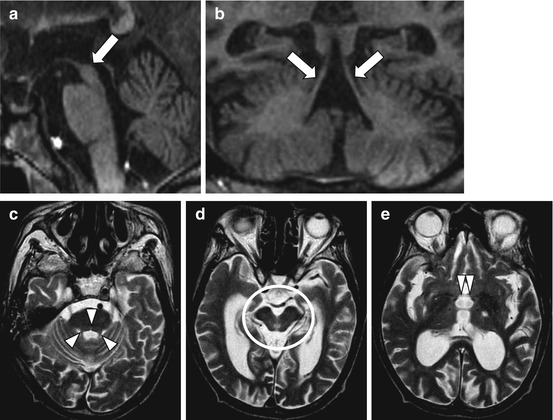
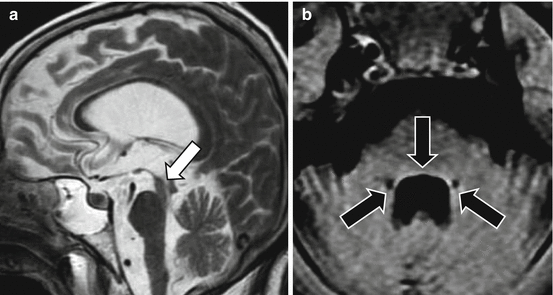
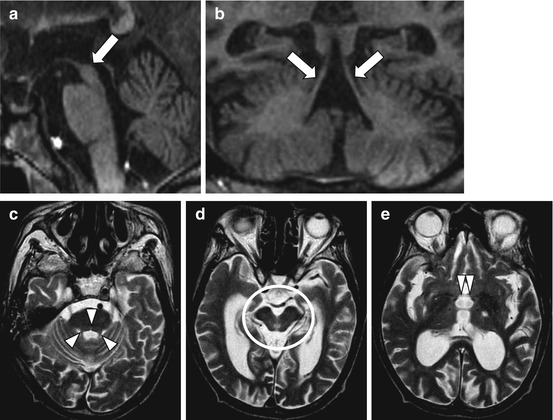
Fig. 4.7
PSP-RS. 80s male. Pathologically proven PSP (PSP-RS). (a, b) Eight years after symptoms onset, reconstructed sagittal and oblique coronal three-dimensional (3D) T1-weighted images (T1WI) reveal the severe atrophy of the midbrain tegmentum and superior cerebellar peduncles (arrows). This characteristic shape of the brainstem including the small midbrain with a concave profile and large pons indicative of “hummingbird sign” or “penguin-silhouette sign” is a typical imaging finding of PSP. (c–e) The atrophy of the pontine tegmentum, bilateral superior cerebellar peduncles (c, arrowheads), the reduced anteroposterior midbrain diameter referred as “Mickey mouse sign” (d, circle), and thalami, and dilatation of the third ventricles (e, double arrowhead), which are also typical imaging findings, are apparent on axial T2-weighted images (T2WI). Small round high signal intensity in the left thalamus indicates an old infarction
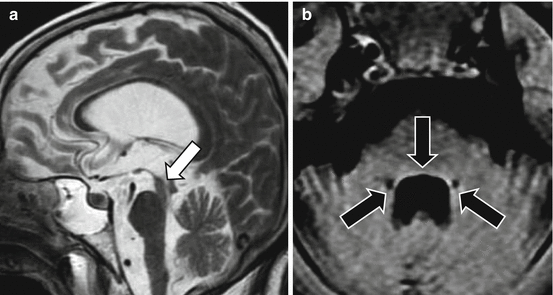
Fig. 4.8
PSP-RS. 70s female. Pathologically proven PSP (PSP-RS). (a) Nine years after symptoms onset, in addition to the typical midbrain atrophy (arrow), sagittal T2WI reveals the atrophy of the corpus callosum and dilatation of frontal sulcus suggestive of the frontal lobe atrophy. (b) Subtle high signal intensity of the superior cerebellar peduncles and brainstem tegmentum on fluid attenuated inversion recovery (FLAIR) images (arrowheads) is also a characteristic finding of PSP. (Courtesy of Dr. Fuji Yokoi, Narita Memorial Hospital, Meiyokai Medical Corporation)
Atrophy of the superior cerebellar peduncle reflects secondary changes of the efferent pathway caused by degeneration of the dentate nucleus of the cerebellum, and it has a high diagnostic value (Figs. 4.7 and 4.8) [29]. In addition, dilatation of the 3rd and 4th ventricles and atrophy of the pontine tegmentum, anterior cingulate gyrus, and medial frontal lobe may also contribute to making a diagnosis [30]. A high intensity may be observed in the globus pallidus, periaqueductal region, and superior cerebellar peduncle on T2-weighted imaging (Fig. 4.8) [31]. These atrophic changes can be objectively evaluated using area measurement with a medial sagittal view and voxel-based morphometry (VBM). Regarding VBM, in addition to the midbrain tegmentum, atrophy of the frontal lobe including the region near the precentral gyrus, caudate nucleus, thalamus, and cerebellum has been reported [32–35]. However, neuroradiologists should understand that even PSP-RS may not exhibit midbrain atrophy in the early disease process (Fig. 4.9).
Regarding evaluation of the microstructural changes based on water diffusivity using diffusion tensor imaging (DTI), it has been reported that differentiations not only between PSP and other parkinsonism but also between PSP-RS and PSP-P are necessary [36]. Greater importance is attached to the superior cerebellar peduncle in evaluation using DTI as well as conventional morphological imaging [37, 38].
Susceptibility weighted imaging (SWI) is a technique with a long echo time high-resolution fully flow-compensated 3D GRE sequence, which has higher sensitivity to the brain mineralization, including iron depositions and blood products, than conventional T2*-weighted imaging [39, 40]. Additionally, this sequence can create better anatomic images in which the basal ganglia structures are easily visible. Previous studies utilizing SWI have reported that the iron deposition in the red nucleus is useful to differentiate PSP from other parkinsonian syndromes [41, 42].
4.4.3.2 PSP-Subtypes
Milder atrophy of the midbrain and superior cerebellar peduncle in PSP-subtypes than in PSP-RS has been reported, to which attention should be paid when making a diagnosis. To make a correct diagnosis of these subtypes, radiologists should focus on the different regions, including the globus pallidus, frontal lobe, and cerebral peduncle, other than the midbrain [42]. Evaluations of the atrophied regions by disease types using statistical imaging analysis, such as VBM analysis, may be accumulated in the future [34, 42–47].
4.4.3.3 PSP-P
PSP-P is the second most frequent subtype following PSP-RS, accounting for 20–35% of PSP variant cases. The patients with PSP-P had less severe tau pathology than those with PSP-RS, although the pattern of distribution between both groups was similar: the subthalamic nucleus and substantia nigra were the regions that were most severely affected. Although the substantia nigra is severely affected in PSP-P, the clinical features that distinguish PSP-P from PSP-RS, including tremor and moderate levo-dopa responsiveness, might be due to less severe depletion of dopamine in the extranigral midbrain in PSP-P [25, 48]. Its response to levo-dopa may be favorable in the early stage in some cases, but the disease stage progresses more rapidly compared with PD. Ophthalmoplegia and postural instability appear and the response to levo-dopa becomes poor as the disease stage progresses. Compared with typical PSP-RS, the degree of midbrain atrophy is less severe in this subtype (Fig. 4.10). Another important point is that atrophy of the superior cerebellar peduncles is less severe in PSP-P patients. Therefore, only the pons/midbrain ratio is useful to distinguish PSP-P from PD patients, but with a relatively lower diagnostic accuracy (sensitivity = 60%, specificity = 96%, accuracy = 86%) [49]. In reports on quantitative analysis, midbrain atrophy is not as noticeable as that in PSP-RS, and atrophy of the midbrain, superior cerebellar peduncle, thalamus, and frontal white matter was also reported to be milder than that in PSP-RS [34].
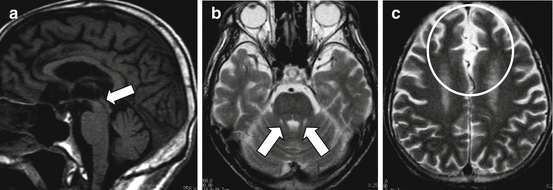
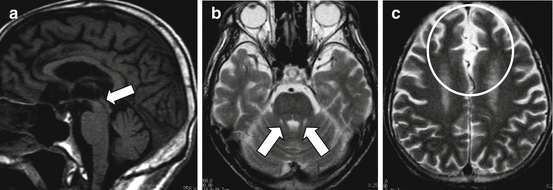
Fig. 4.10
PSP-P. 90s male. Pathologically proven PSP-P. (a, b) Compared with typical PSP-RS cases, there are no obvious atrophic changes of the midbrain tegmentum (a: arrow), and superior cerebellar peduncles (b: arrows) on sagittal and axial image. (c) T2WI reveals atrophy in the anterior cingulate gyrus (circle)
4.4.3.4 PSP-PAGF
PSP-PAGF is characterized by early difficulties in initiating gait and freezing during walking, writing, and speaking, without rigidity, tremor, cognitive impairment, or eye movement abnormality during the first 5 years of the disease. Levo-dopa is not effective. No characteristic finding is observed on visual inspection [50]. However, T2 prolongation of the globus pallidus can be the diagnostic clue in this subtype [51] (Fig. 4.11). On VBM analysis, atrophy of the thalamus, globus pallidus, frontal, inferior parietal, temporal lobes, and limbic lobes including the hippocampal region and anterior cingulate gyrus, compared with normal controls has been reported [52] (Fig. 4.11). It is necessary to accumulate cases to investigate morphological differences from those of PSP-RS and other PSP-subtypes and associations with clinical findings and the background pathology.
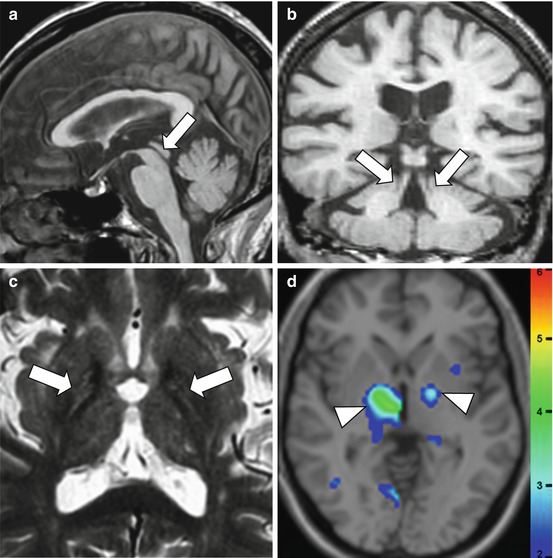
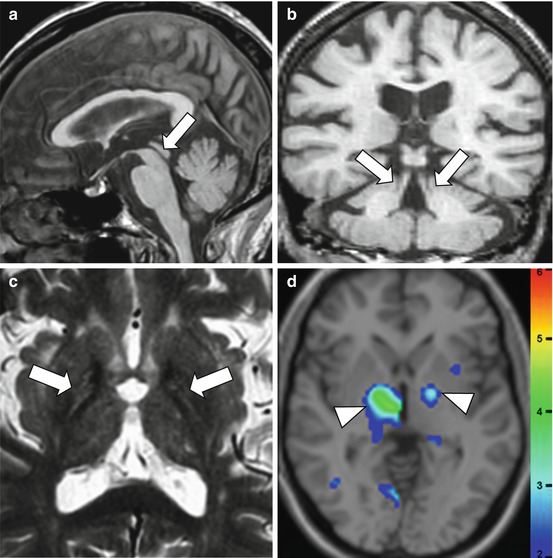
Fig. 4.11
PSP-PAGF. 80s female. Pathologically proven PSP (PSP-PAGF). (a, b) Compared with typical PSP-RS cases, there are no obvious atrophic changes of the midbrain tegmentum (a: arrow), corpus callosum and superior cerebellar peduncles (b: arrows) on sagittal and reconstructed oblique coronal 3DT1WIs. (c) On the other hand, T2WI reveals right dominant bilateral triangle-shaped high signal intensities of the globus pallidus (arrows). (d) Of note, voxel-based morphometry (VBM) on Voxel-based Specific Regional analysis system for Alzheimer’s disease (VSRAD) software can objectively display right dominant bilateral atrophy of the globus pallidus and right thalamus (arrowheads), which are prediction sites of pathological changes in PSP patients. The degree of atrophy is represented using Z-scores on the rainbow scale color bar
4.4.3.5 PSP-PNFA
There is a rare subtype of PSP which causes marked progressive speech and language dysfunction as a progressive non-fluent aphasia. PNFA is known as a disease type of frontotemporal lobar degeneration (FTLD), and PSP may be present as a background pathology in some cases. Accurate diagnosis of the pathology is also needed with regard to care and nursing, such as the necessity of considering the risk of falls due to background PSP. Predominant frontal lobe atrophy, particularly on the left side, in PSP-PNFA has been reported, and atrophy of the ipsilateral cerebral peduncle is also observed [53–55] (Fig. 4.13).
Midbrain atrophy is not as noticeable as that in PSP-RS, but it is atrophied compared with normal controls, and the disease may be diagnosed based on this. On VBM analysis, it has been reported that the main cortical regions with atrophy were the premotor and supplemental motor areas in cases of apraxia of speech, and the anterior periSylvian area in PNFA cases [54, 56] (Figs. 4.12 and 4.13). The accumulation of analyses connecting the clinical, pathological, and imaging findings is desired.
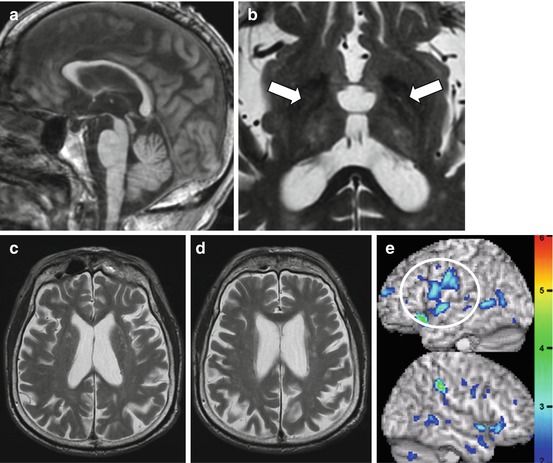
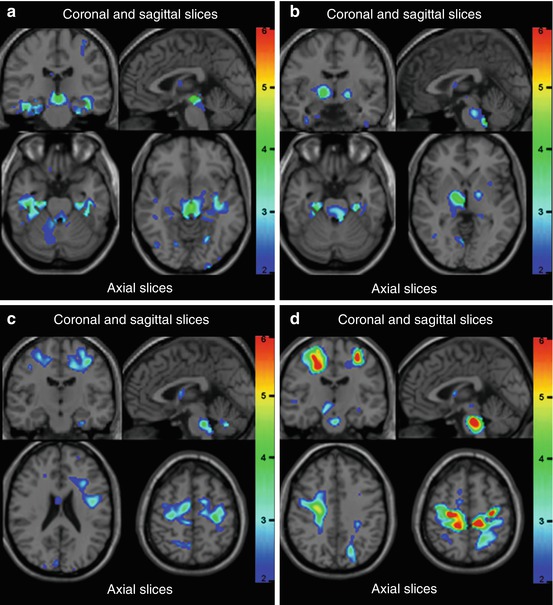
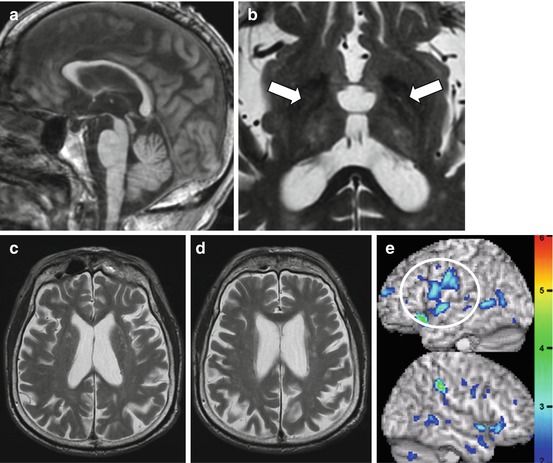
Fig. 4.12
PSP-PNFA. 80s male. Pathologically proven PSP (PSP-PNFA). (a) The degree of the midbrain atrophy is relatively mild on sagittal 3DT1WI. (b):T2WI reveals bilateral club-shaped high signal intensity of the globus pallidus (arrows). (c–e) Although it is difficult to evaluate the left dominant frontal atrophy on visual inspection of T2WIs (c, d), VBM on VSRAD software can display the left dominant frontal gray and white matter atrophy. 3D render views emphasize the left frontal lobe gray matter atrophy, especially in the Broca’s area (e, circle)
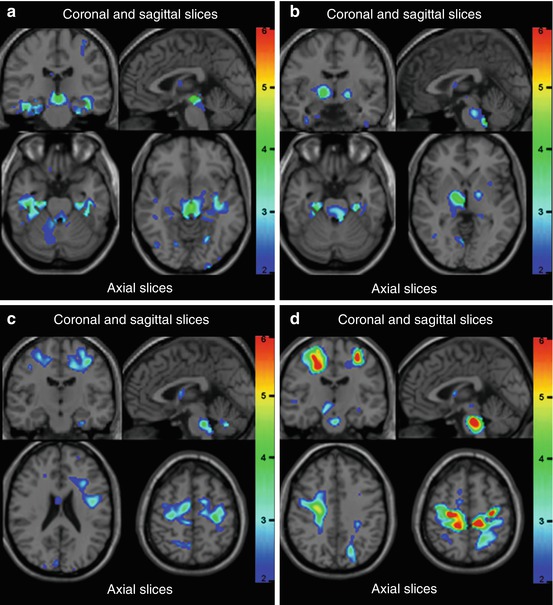
Fig. 4.13
Axial views of VBM result in pathologically proven PSP-RS (a), PSP-PAGF (b), PSP-PNFA (c), and PSP-CBS (d) patients. VBM analyses can reveal the characteristic white matter atrophy of PSP clinical subtypes indicative of the midbrain and superior cerebellar peduncles of PSP-RS, globus pallidus of PSP-PAGF, left frontal lobe including the inferior frontal gyrus of PSP-PNFA, and asymmetric frontoparietal lobes around the central sulcus of PSP-CBS. Additionally, most subtypes reveal the atrophy of the pontine tegmentum
4.4.4 Differential Diagnosis
Midbrain tegmentum atrophy in the median sagittal view on MRI is an important finding based on which PSP may be diagnosed. However, PSP is not the only disease causing midbrain tegmentum atrophy, and corticobasal degeneration (CBD) is the most important disease to be differentiated. In CBD, cortical atrophy with laterality and laterality of accompanying cerebral peduncle atrophy are observed, but differentiation is difficult because laterality is also observed in PSP-PNFA and PSP-CBS. It is known that pathological changes in the white matter are marked in CBD compared with those in PSP, which is useful to differentiate the disease in many cases, and evaluation using VBM has been reported, in addition to visual examination. Machado–Joseph disease and dentatorubral-pallidoluysian atrophy, which are forms of autosomal dominant spinocerebellar ataxias, can also exhibit the atrophy of the midbrain and superior cerebellar peduncle. Midbrain atrophy may also develop in idiopathic normal pressure hydrocephalus (i-NPH). Tight high convexity, medial subarachnoid spaces, and enlarged Sylvian fissures with ventriculomegaly can be defined as disproportionately enlarged subarachnoid-space hydrocephalus (DESH), which is a characteristic of iNPH. Patients with DESH can exhibit midbrain atrophy indistinguishable from that of PSP.
4.5 Corticobasal Degeneration and Corticobasal Syndrome
4.5.1 Disease Concept
Corticobasal degeneration (CBD) is a progressive neurodegenerative disorder first described by Rebeiz et al. [57] and characterized by progressive, asymmetric, cortical dysfunction (e.g., apraxia, alien limb sign, cortical sensory loss, and myoclonus), and extrapyramidal dysfunctions (e.g., akinesia, dystonia) [57]. As a pathologic diagnosis, CBD is characterized by widespread deposition of hyperphosphorylated 4-repeat tau in neurons and glia in specific topographic areas [58]. When first described, CBD was considered a distinct clinicopathologic entity, however, CBD has much clinicopathological heterogeneity [59–61], the term corticobasal syndrome (CBS) has been used as a clinical diagnosis, and CBD is used to refer to the pathological diagnosis [59, 60].
CBS can be the clinical syndrome of a host of different background pathologies, including corticobasal degeneration (CBS-CBD), progressive supranuclear palsy (CBS-PSP), Alzheimer’s disease (CBS-AD), and frontotemporal lobar degeneration (CBS-FTLD).
4.5.2 Clinical Diagnosis
Definition and standardization of clinical diagnostic criteria for CBD are critical as potential development of curative therapeutic drugs for tauopathies. Current criteria for the clinical diagnosis of pathologically confirmed CBD no longer reflect the expanding understanding of this disease and its clinicopathological correlations. An international consortium of behavioral neurology, neuropsychology, and movement disorders specialists developed new criteria based on consensus and a systemic literature review [62]. Combined with consensus, four CBD phenotypes emerged: corticobasal syndrome (CBS), frontal behavioral-spatial syndrome (FBS), non-fluent/agrammatic variant of primary progressive aphasia (naPPA), and progressive supranuclear palsy syndrome (PSPS). They propose two sets of criteria: more specific clinical research criteria for probable CBD (diagnostic criteria excluding background pathology other than cr-CBD) and broader criteria for possible CBD (p-CBD) that has less specificity for CBD pathology while representing other tau-based pathology [62].
4.5.3 MRI Findings
Although neuroimaging is not described in the latest diagnostic criteria described above, these criteria will need continued revisions as understanding of CBD and as neuroimaging studies advance, and are validated for distinguishing different phenotypes and diagnoses.
At present, there are two main roles of MRI in diagnosing CBD. The first role is clarification of MRI findings useful for cr-CBD, for which tenacious investigation connecting the clinical findings-imaging-background pathology is necessary. Previous MRI studies have reported distinct neuroimaging findings of pathologically diagnosed CBD, including asymmetric atrophy in the cerebral cortex and peduncle with dominance contralateral to the more clinically affected side, atrophy of the midbrain tegmentum and corpus callosum, and abnormal T2 prolongation in the subcortical white matter [55, 66] (Figs. 4.14 and 4.15). At sites where MRI showed white matter lesions, although neuropathological examination revealed some secondary degeneration, tauopathy in the white matter, particularly the subcortical white matter, was clearly evident [66] (Fig. 4.14). Visually, the grade of changes in the intensity of the white matter varies among cases from widely extending to unclear changes. Atrophy of the midbrain tegmentum is present in both CBD and PSP, reflecting the pathology, and their differentiation may be difficult clinically or on imaging in some cases, but white matter degeneration impairing U-fibers is pathologically severer in CBD than PSP, and changes in the intensity of the white matter may serve as a point of differentiation. However, its judgment by visual evaluation alone may be difficult, for which the usefulness of objective evaluation by Voxel-based morphometry (VBM) analysis has been reported. VBM analysis using SPM8 plus DARTEL demonstrated the diagnostic value of significant atrophy in the bilateral frontal subcortical WM for diagnosing CBS [67]. Thus, the VBM approach can be useful for discriminating between CBS and PSP-RS. However, considering the broad clinical spectrum of CBD, more pathologically proven cases of CBD are required to establish the diagnostic value of WM volume reduction on VBM analysis. It is difficult to differentiate CBD from PSP-CBS, PSP-PNFA even using VBM analysis of WM volume reduction.
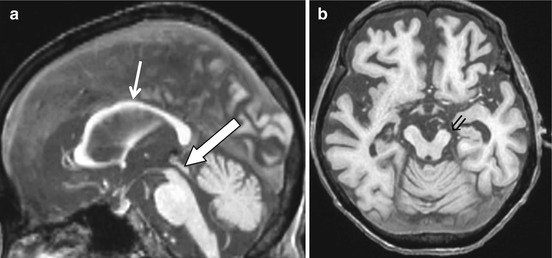
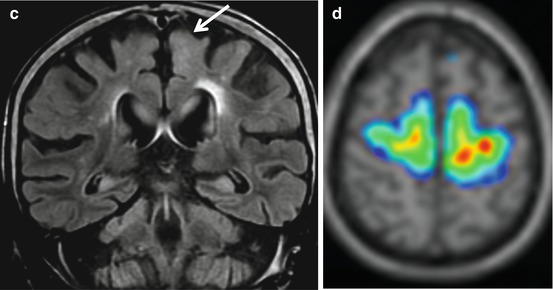
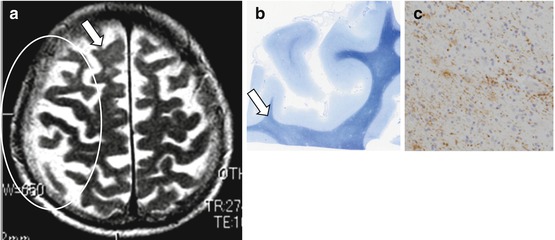
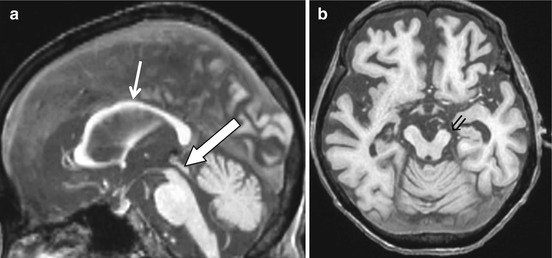
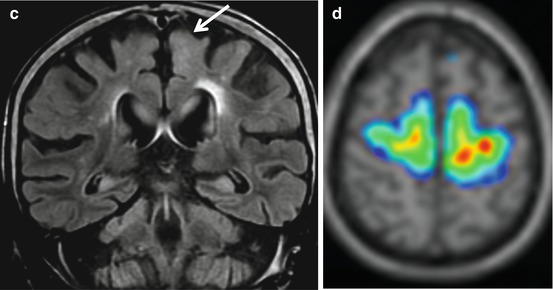
Fig. 4.14
80s female. CBD. (a) Sagittal T1-weighted image shows severe atrophy of midbrain (bold arrow) and thinning of corpus callosum (arrow). (b) T1-weighted axial image shows left dominant atrophy of cerebral peduncle (arrow). (c) FLAIR coronal image shows hyperintensity in the subcortical white matter (arrow). (d) Axial views of VBM reveal left dominant white matter atrophy
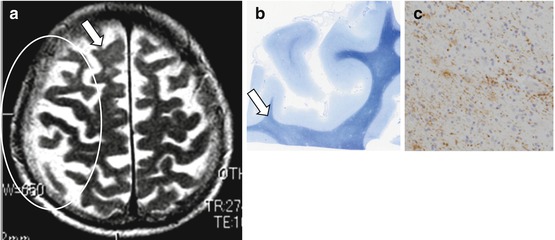
Fig. 4.15
Pathologically proven CBD:80s female. (a) Axial T2-weighted image shows right dominant atorophy (circle)and subcortical hyperintensity in the right frontal lobe (arrow). (b) In a microscopic specimen, myelin sheath staining is decreased in the white matter (arrow). (c) There is positive staining for antiphosphorylated tau antibody on AT8 staining, which is compatible with the primary changes in CBD
The second role of MRI is differentiation of diseases with background pathology included in p-CBD other than CBD [68, 69]. Although there are diverse pathological backgrounds, lesions in the cortex of the frontal and parietal lobes and regions involved in- and output are present in common, suggesting that clinical symptoms are determined by localization of the lesions, but not by background pathology of the lesions, i.e., apraxia and cortical sensory loss may be due to lesions in the parietal lobe and alien limb signs may be due to lesions in the frontal or parietal lobe. It is essential to realize that AD, FTLD, PSP, and Creutzfeldt-Jakob disease (CJD) are present as the background pathology of CBS. In CJD, a high intensity in the cortex on diffusion-weighted MRI is the characteristic, and MRI performed at an appropriate timing is useful for exclusion diagnosis. There are still only a few reports on comparison between pathologically diagnosed CBS and images, VBM analysis has been reported. Imaging patterns of atrophy in CBS vary according to pathologic diagnosis. Widespread atrophy points toward a pathologic diagnosis of FTLD-TDP or AD, with frontotemporal atrophy suggesting FTLD-TDP and temporoparietal atrophy suggesting AD. On the contrary, more focal atrophy predominantly involving the premotor and supplemental motor area suggests CBD or PSP pathology. These atrophy patterns in CBS are promising but require prospective validation prior to inclusion in diagnostic criteria.
Diffusion tensor imaging (DTI) measures the translational movement of water molecules in biologic tissues. It measures fractional anisotropy (FA), an index of white matter coherence and axonal packing, and apparent diffusion coefficient average (ADC ave), an index of the average magnitude of the rate of diffusion within each voxel. DTI has a possibility to sharply detect different distributions of microstructural tissue damage, and its usefulness for differentiation between PS and CBD has been reported.
Detections of atrophy on MRI and microstructure damage on DTI are expected to serve as biomarkers which may correspond to the molecular pathology [70]. To attempt treatment targeting βamyloid, tau, and TDP-43, accumulation of investigations connecting clinical-neuroimaging-pathology is necessary to play a specific role as objective criteria.
4.6 Hippocampal Sclerosis of Aging
4.6.1 Disease Concept
Hippocampal sclerosis of aging (HS-Aging) is a relatively common neuropathological finding characterized by cell loss, gliosis, and atrophy in the hippocampus that is not explained by AD [71–79]. HS-Aging pathology is observed in up to 20% of individuals over the age of 85 years [73, 77]. The presence of HS-Aging pathology is associated with cognitive impairment [72–76]. HS-Aging is known as a complication of AD, DLB, and FTLD, but, independently from these pathologies, pathological reports of HS-Aging, in which neuronal loss and gliosis are detected in the CA1 area of the hippocampus and subiculum, have recently been accumulating [71–79]. A high frequency of the TAR DNA-binding protein 43 (TDP-43) pathology has also been reported, but investigation of its association with clinical and imaging findings is insufficient [71, 73, 76]. While investigation is proceeding from the viewpoint that it is an independent neurodegenerative disease, changes accompanying posticteric encephalopathy, hypoxic-ischemic encephalopathy, encephalitis, and vascular disorder, or superimposition of these are also assumed, and its pathology may be diverse.
HS-Aging is present in the background pathology at a relatively high frequency in the elderly. Its importance as a factor of dementia has been clarified by investigation of the background pathology; however, establishment of consensus guidelines for clinical diagnosis is needed, for which development of objective biomarkers including neuroimaging is an urgent task.
4.6.2 Neuroimaging
Only a few studies on neuroimaging based on the background pathology of HS-Aging have been reported [71, 76, 77], but MRI is expected to be an important tool as a biomarker to diagnose HS-Aging clinically. To acquire images of the hippocampus, observation of the coronal view vertical to the long axis of the hippocampus is necessary. The sagittal plane was acquired, and the coronal section vertical to the hippocampus was set. The basic sequences of evaluation of hippocampal sclerosis are fast spin echo T2-weight imaging, and fluid attenuated inversion recovery (FLAIR).
The MRI findings of hippocampal sclerosis are atrophy and a high intensity on T2-weighted imaging and FLAIR (Figs. 4.16, 4.17, and 4.18). It develops on the unilateral side in many cases, but there are bilateral cases. Differentiation from AD is essential because hippocampal atrophy is observed. When clinical progress of dementia is slow despite a clear high intensity and atrophy being detected on T2-weighted imaging and FLAIR of the hippocampal formation, the possibility of hippocampal sclerosis with relatively preserved verbal fluency despite impaired word list delayed recall performance (which is informative because the former relies more on neocortical function and the latter on brain function referent more directly to the hippocampal formation) is considered [75]. Clinically, many cases were diagnosed as AD or clinically diagnosed as FTLD [73–78], but improvement of the sensitivity and specificity of differential diagnosis by further accumulating definite pathologically diagnosed cases is necessary.
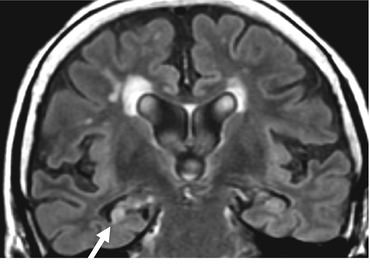
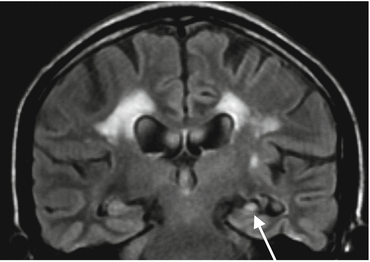
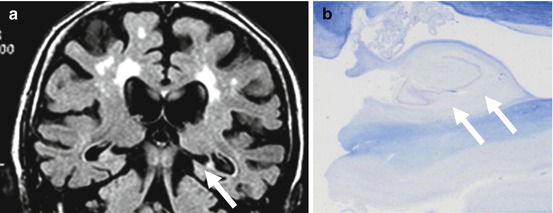
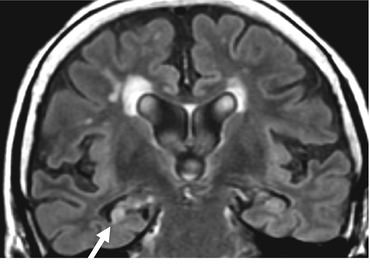
Fig. 4.16
70s female, memory disturbance. On the coronal view of FLAIR, local atrophy and a high intensity of the right hippocampus were clear, suggesting hippocampal sclerosis
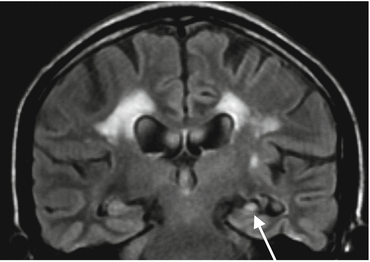
Fig. 4.17
70s male with dementia without marked progression for 12 years. He had past history of epilepsy. Left-side dominant local atrophy of the hippocampus and an increased intensity on FLAIR were clear (arrow). Left-side dominant hippocampal sclerosis was suggested
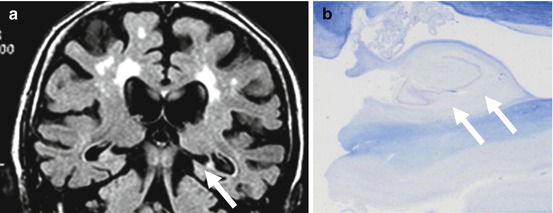
Fig. 4.18
80s male. (a) Left hippocampal atrophy and an increased intensity were noted in the coronal view on FLAIR (arrow). Morphologically, differentiation of hippocampal sclerosis was necessary. (b) The background pathology was neuronal loss in the left hippocampus CA1-2 (arrows), corresponding to hippocampal sclerosis. Accumulation of TDP-43 was also noted
The severity of dementia may be different due to the presence of hippocampal sclerosis on the right or left side. Attention should be paid to whether or not hippocampal sclerosis is located in the dominant hemisphere of the patient.
Atrophy and an abnormal intensity of the hippocampus suggest hippocampal sclerosis, but at present, attention should be paid to the possible heterogeneity of its background pathology, and attitude to diagnose along the clinical course of individual cases is essential.
In studies on HS-Aging, dementia is the main symptom and the frequency of epilepsy is low. Based on experience of the elderly at an emergency site, when the morphology suggests hippocampal sclerosis, similarly to early-onset cases, careful investigation of a past medical history of epilepsy, present illness, infection, vascular disorder, a past medical history of trauma, and the course may be significant to prepare a treatment plan [79–81]. Epilepsy symptoms may be recognized as dementia in elderly patients because many seizure episodes are non-convulsive, making diagnosis difficult in some cases. In this aging society, rapid elevation of the incidence of epilepsy in the elderly aged 70 years or older should be kept in mind [80, 81]. Attention should be paid to whether or not cases showing hippocampal sclerosis on MRI are included in the case group.
It has been reported that TDP-43 was negative on brain sections from younger individuals after hippocampectomy due to seizures, who had pathologically confirmed hippocampal sclerosis, but further accumulation of cases is necessary to investigate differences and overlapping between HS-Aging with foreground dementia in the elderly and early-onset hippocampal sclerosis manifesting epilepsy as a clinical symptom.
4.6.3 Differential Diagnosis
Alzheimer’s disease, dementia with grains should be included in clinical and imaging differential diagnoses. For neuroimaging, reliably diagnosing encephalitis (Fig. 4.19), posticteric encephalopathy (Fig. 4.20), hypoxic-ischemic encephalopathy, and cerebral infarction during the course is also important.
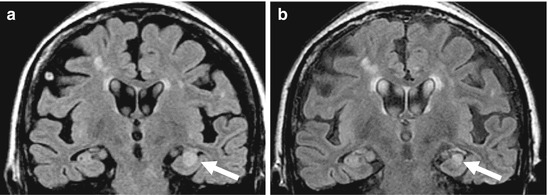
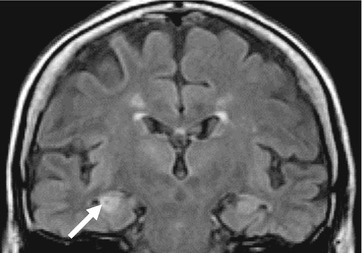
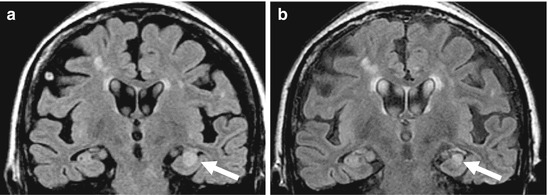
Fig. 4.19
70s female with suspected non-herpetic limbic encephalitis (NHLE). (a) Coronal FLAIR image shows left hippocampal swelling and high intensity at the onset of NHLE and the increased intensity prolonged on FLAIR (arrow). (b) 11 months later of onset, coronal FLAIR image shows hippocampal atrophy with hyperintensity (arrow). The clinical course was clearly followed and imaging findings were also followed as the course of NHLE. However, if the first examination was performed at 11 months after the onset when the MMSE score was 24 showing mild cognitive dysfunction, how do radiologists consider these findings? They may include hippocampal sclerosis in differentiation, and at the same time, careful consideration of the course after inflammation and convulsion and the course of ischemia would be necessary. (From [79] with permission)
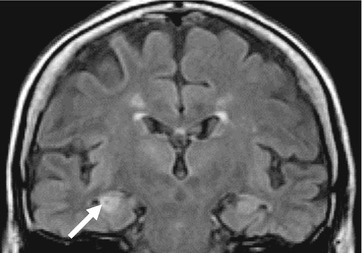
Fig. 4.20
80s female. Coronal FLAIR image was taken a 10 days after convulsive attack. This image shows high intensity in the right hippocampus probably due to posticteric encephalitis. (From [79] with permission)
4.7 Idiopathic Normal Pressure Hydrocephalus (iNPH)
4.7.1 Disease Concept
Normal pressure hydrocephalus (NPH) is well known as a treatable cause of dementia in elderly, and is characterized by the classical clinical triad of gait disturbance, dementia, and urinary incontinence [82–84]. NPH can be classified into idiopathic NPH (iNPH) and secondary NPH (See Table 4.2). International guidelines and Japanese guidelines for the diagnosis and management of iNPH have been published [82–89]. The etiology and pathomechanism of iNPH remain unknown. Clinical improvement by ventricle-peritoneal (VP) and lumbo-peritoneal (LP) shunts based on appropriate diagnostic criteria can be expected, and neuroimaging providing objective information plays a major role.
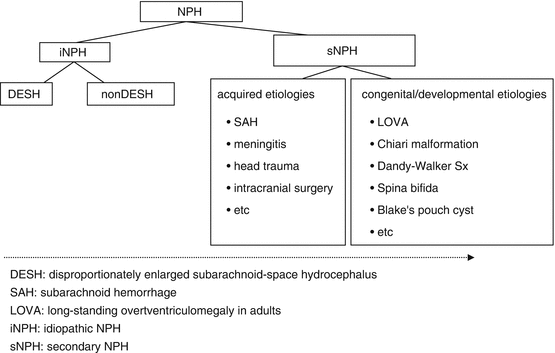
Table 4.2
Classification of normal pressure hydrocephalus
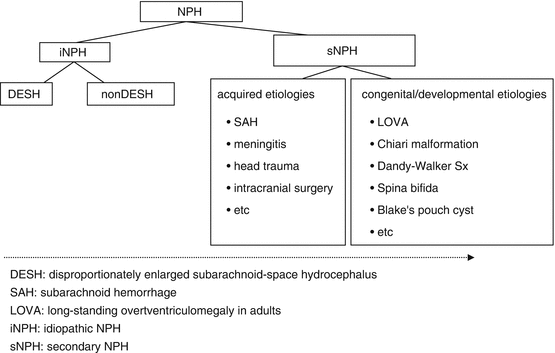
4.7.2 MRI Findings of iNPH
Table 4.3 shows the points of the diagnostic criteria for iNPH proposed in 2011 [86]. MRI plays an important role in making a diagnosis, and acquisition of the coronal and sagittal images, in addition to the transverse image, is recommended. There are 3 main findings of iNPH on MRI, as follows (Figs. 4.21, 4.22, and 4.23):
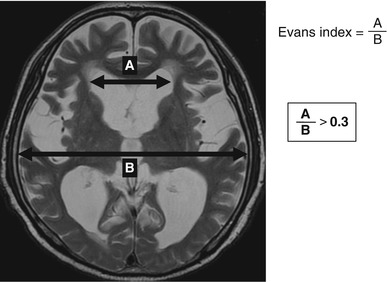
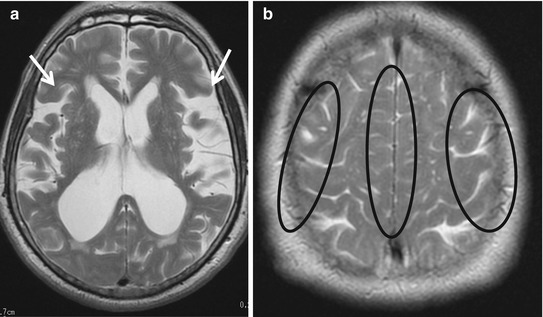
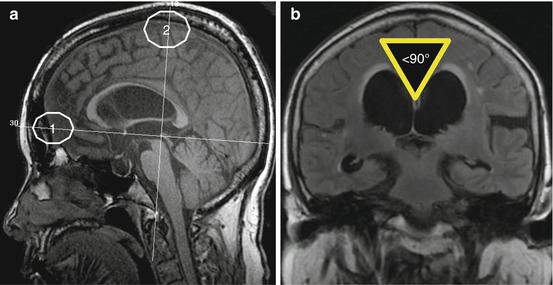
Table 4.3
Diagnostic criteria for idiopathic normal pressure hydrocephalus (iNPH) in these revised guidelines
1. Possible iNPH: meets all of the following five features: |
(1) Individuals who develop the symptoms in their 60s or older |
(2) More than one of the clinical triad: gait disturbance, cognitive impairment, and urinary incontinence |
(3) Ventricular dilatation (Evans index > 0.3) |
(4) Above-mentioned clinical symptoms cannot be completely explained by other neurological or non-neurological diseases |
(5) Preceding diseases possibly causing ventricular dilatation are not obvious, including subarachnoid hemorrhage, meningitis, head injury. congenital hydrocephalus, and aqueductal stenosis |
Possible iNPH supportive features |
(a) Small stride, shuffle, instability during walking, and increase of instability on turning |
(b) Symptoms progress slowly; however, sometimes an undulating course, including temporal discontinuation of development and exacerbation, is observed |
(c) Gait disturbance is the most prevalent feature, followed by cognitive impairment and urinary incontinence |
(d) Cognitive impairment is detected on cognitive tests |
(e) Sylvian fissures and basal cistern are usually enlarged |
(f) Other neurological diseases, including Parkinson’s disease, Alzheimer’s disease, and cerebrovascular diseases, may coexist; however, all such disease should be mild |
(g) Periventricular changes are not essential |
(h) Measurement of CBF is useful for differentiation from other dementias |
Possible iNPH with MRI support |
Possible iNPH with MRI support indicates the condition fulfilling the requirements for possible iNPH, where MRI shows narrowing of the sulci and subarachnoid spaces over the high convexity/midline surface (DESH). This class of diagnosis can be used in circumstances where a CSF examination is not available, for example, in a population-based cohort study |
2. Probable iNPH: meets all of the following three features: |
(1) Meets the requirements for possible iNPH |
(2) CSF pressure of 200mm H2O or less and normal CSF content |
(3) One of the following three investigational features: |
(a) Neuroimaging features of narrowing of the sulci and subarachnoid spaces over the high convexity/midline surface(DESH) under the presence of gait disturbance |
(b) Improvement of symptoms after CSF tap test |
(c) Improvement of symptoms after CSF drainage test |
3. Definite iNPH |
Improvement of symptoms after the shunt procedure |
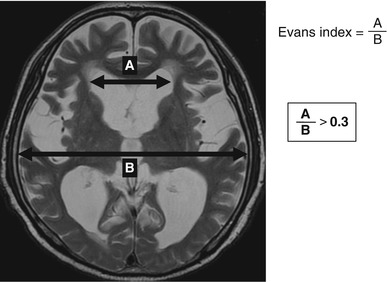
Fig. 4.21
Evans index = A/B. A/B ≥ 0.3. A = the largest diameter of the frontal horns. B = the diameter of the internal skull, on the same plane. (Courtesy of Dr. Mori Harushi, Department of Radiology, The University of Tokyo)
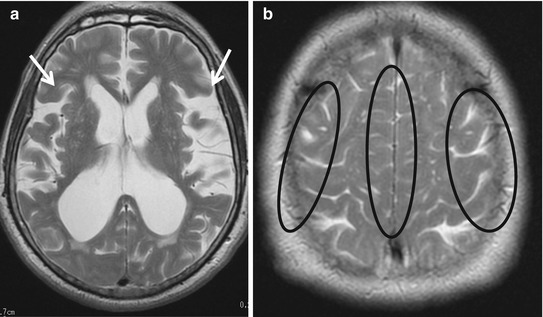
Fig. 4.22
DESH 80s male. He had gait disturbance, urinary incontinence, and cognitive impairment. (a) Axial T2-weighted image shows ventricular enlargement and Sylvian fissure (arrow) (b) Axial T2-weighted image shows tightened high convexity/midline CSF space (circles). (Courtesy of Dr. Mori Harushi, Department of Radiology, The University of Tokyo)
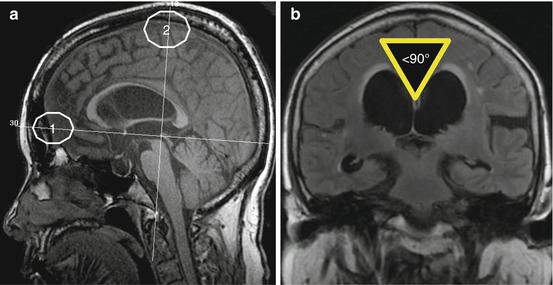
Fig. 4.23
CA (callosal angle): 60s female with gait disturbance. (a) 1 = the anteroposterior commissure line. 2 = coronal plane just on the posterior commissure perpendicular to the anteroposterior commissure line. (b) CA is under 90° in the coronal plane just on the posterior commissure. (Courtesy of Dr. Mori Harushi, Department of Radiology, The University of Tokyo)
(1) Ventricular dilatation: Evans index (determined by the largest diameter of the frontal horns divided by the diameter of the internal skull, on the same plane) >0.3 (Fig. 4.21) [85, 90, 91], (2) enlargement of Sylvian fissure, and (3) basal cistern tightened high convexity/midline CSF space. A pathological state meeting all these conditions and reflecting disproportional distribution of CSF between the superior and inferior subarachnoid spaces is termed disproportionately enlarged subarachnoid-space hydrocephalus (DESH), and many cases of iNPH show this morphology [90–92]. Sylvian fissure dilatation is less likely to be observed in NPH, other than iNPH. DESH is characterized by tight high convexity and medial subarachnoid space, but local cerebral sulcus dilatation is often observed (Fig. 4.24).
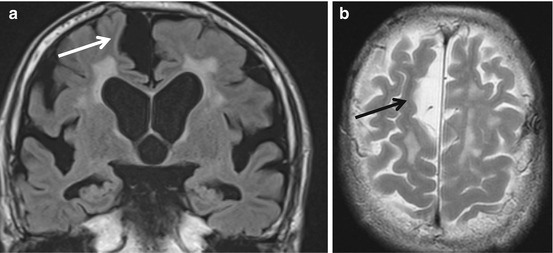
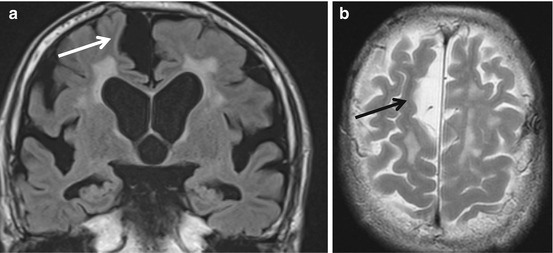
Fig. 4.24
80s M, iNPH. He had cognitive decline and gait disturbance. His background pathology did not show any degenerative diseases. (a) FLAIR coronal image shows dilatation of lateral ventricle and Sylvian fissure. (b) Axial T2-weighted image shows tightened CSF space in the posterior medial side of high convexity. Local cerebral sulcus dilatation is observed in (a and b) (arrows)
Reference findings include dilatation of the anterior cingulate sulci [92, 93] and narrowing of the corpus callosal angle (CA) [94] (Fig. 4.23). CA represents the angle formed by the corpus callosum on the posterior commissure perpendicular to the anteroposterior commissure line (AC-PC line) on coronal MRI (Fig. 4.23). It has been reported that when the angle is 90° or smaller, the angle is useful for differentiation between normal aging and AD at an accuracy of 93%, sensitivity of 97%, and specificity of 88% [91]. Many iNPH cases are not accompanied by periventricular hyperintensity (PVH), and currently, the presence or absence of PVH does not contribute to the differentiation of iNPH.
iNPH cases are mixed with cases of ventricular enlargement dilatation showing no DESH finding, and these are termed non-DESH-type iNPH. Some cases are not accompanied by clinical symptoms despite typical DESH findings being observed on MRI. These are considered at high risk of progressing to iNPH, and termed asymptomatic ventriculomegaly with features of iNPH on MRI (AVIM) [95]. Differentiation between DESH and AVIM on MRI is difficult, and it is judged in consideration of clinical symptoms, but it is necessary to call attention to how the follow-up of clinical and MRI findings is essential.
4.7.3 Differential Diagnosis
(1) The incidence of iNPH is high in the elderly. The disease is accompanied by cognitive impairment, it may be treatable, and its differentiation from Alzheimer’s disease (AD) and Parkinson’s disease (PD) is important. Since the DESH findings were proposed, the accuracy of diagnosing iNPH by MRI improved, but it should be noted that iNPH is frequently complicated by AD, PD, and cerebrovascular disease (CVD) in elderly patients, and the disease is difficult to diagnose by MRI alone. Figures 4.25 and 4.26 show the differentiation points between AD and iNPH on MRI. Ventricular enlargement is observed in both iNPH and AD, but the tightened high convexity/midline CSF space is clear and the callosal angle is 90° or smaller in iNPH, whereas it is unclear in AD. In addition, cingulate sulcus dilatation is detected in the anterior region in iNPH, but it is dominant in the posterior region in AD.
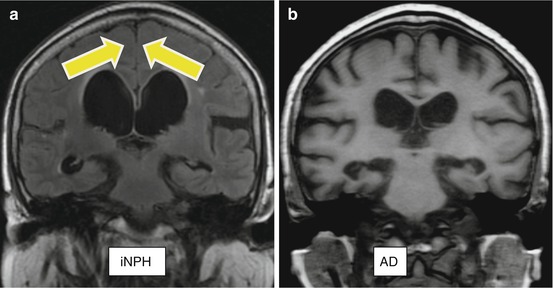
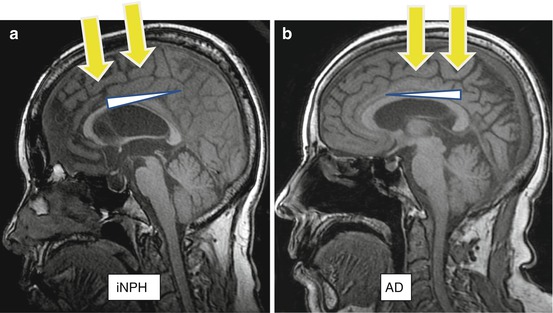
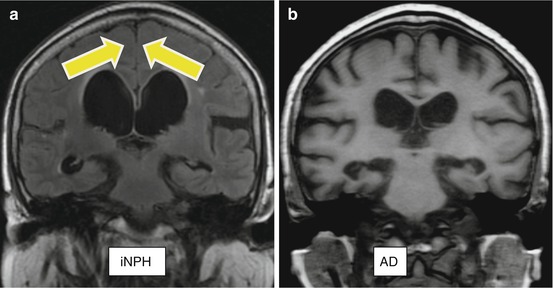
Fig. 4.25
Differential diagnosis between iNPH and AD. (a, b) Ventricular enlargement is observed in both iNPH and AD, but the tightened high convexity/midline CSF space is clear and the callosal angle is 90° or smaller in iNPH (a), whereas it is unclear in AD (b). (Courtesy of Dr. Mori H, Department of Radiology, The University of Tokyo)
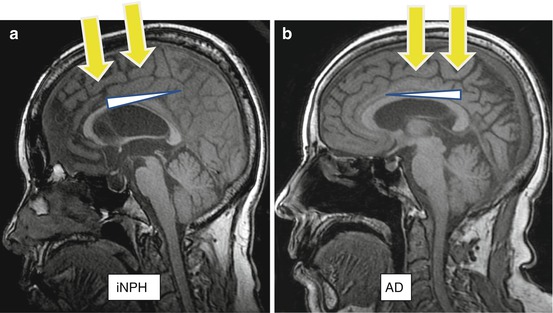
Fig. 4.26
Differential diagnosis between iNPH and AD. (a, b) Cingulate sulcus dilatation is detected in the anterior region in iNPH (a), but it is dominant in the posterior region in AD (b). (Courtesy of Dr. Mori H, Department of Radiology, The University of Tokyo)
Yamashita et al. have been reported recently that the VBM-based CSF space analysis can accurately detect disproportionate changes in the CSF space and differentiate iNPH patients from those with AD or PD and healthy elderlies, for which usefulness is expected [96]. In addition to contributing to early and differential diagnoses, the VBM-based CSF space analysis can be used to predict the onset of diseases and to monitor morphological changes between responders and nonresponders to shunt operations. To apply the VBM-based CSF space analysis as a useful tool and lead it to future multicenter trials, further cross-validation studies are needed.
Midbrain atrophy may also develop in iNPH, and attention should be paid to differentiation of and complication by progressive supranuclear palsy (PSP) and corticobasal degeneration (CBD).
(2) Secondary normal pressure hydrocephalus (sNPH)
sNPH is induced by acquired etiologies, such as subarachnoid hemorrhage (SAH) and meningitis. If the presence of its past medical history is confirmed, the diagnosis can be made without a doubt. Morphologically, ventriculomegaly and a tightened CSF space are observed compared with those in iNPH, but the Sylvian fissure dilatation is mild in many cases.
(3) Long-standing overt ventriculomegaly in adults (LOVA) [97]
In LOVA, ventriculomegaly persists for a prolonged period from infancy. It results in hydrocephalus inducing urinary incontinence and cognitive impairment in adults, and aqueductal stenosis is considered to be the cause (Fig. 4.27) [97]. Long-term ventriculomegaly may be accompanied by sella turcica expansion and destruction. Its incidence is high in the young population, from teens to 60s, compared with that of iNPH. Ventriculomegaly and tightened CSF space are extensively observed, but no Sylvian fissure dilatation is observed (Fig. 4.27).
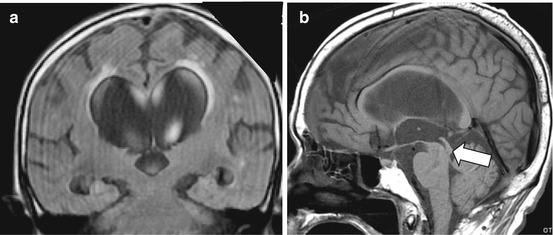
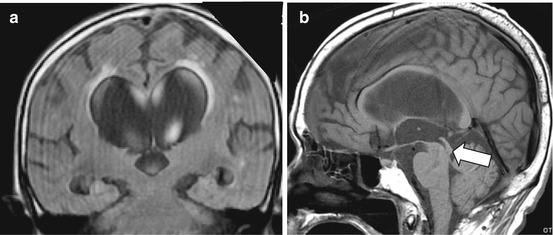
Fig. 4.27
LOVA: 80s M. He had long-standing gait disturbance and easy to fall. (a) There are marked ventriculomegaly without dilatation of Sylvian fissures. (b) There shows stenosis of caudal aqueduct of midbrain (arrow)
4.7.4 Pathophysiology of CSF Dynamics Remains Unknown
The current CSF movement is based on the hypothesis proposed by Dandy and Blackfan [98] and Cushing et al. in the early twentieth century, i.e., there is unidirectional flow in which CSF is produced at the site of the choroid plexus and absorbed in the pacchionian granulations.
Dandy et al. proposed the CSF bulk flow theory: Hydrocephalus is caused by imbalance between CSF formation and absorption. In contrast to the bulk flow theory, Greiz et al. proposed the “hydrodynamic theory” [99]: Chronic hydrocephalus is due to decreased intracranial compliance, causing restricted arterial pulsations and increased capillary pulsations.
However, explanation of the pathology of DESH is difficult with either theory, and at present, the cause of iNPH is unclear. Recently, Yamada et al. investigated CSF movement using the time-spatial labeling inversion pulse (SLIP) technique [100]. According to them, pulsatile CSF flow was observed up to the Sylvian fissure, but no CSF pulsatile flow continuous from the Sylvian fissure to the cerebral convexity was observed, and CSF movement is not limited to steady-state unidirectional flow, suggesting that the previous common wisdom of CSF movement may be changed by the new MR technique.
4.8 Vascular Dementia
4.8.1 Disease Concept
Vascular dementia (VaD) is one of the most common causes of age-associated dementia. In addition, cerebrovascular disease (CVD) may worsen the dementia of neurodegenerative diseases, for example, Alzheimer’s disease (AD), Parkinson’s disease (PD), Lewy body disease (DLB), etc.
There is a diversity in the cause and pathology of VaD, and clinical symptoms and courses are also diverse. When CVD develops, dementia develops at the same time. The causal relationship between these is very clear in some cases, but various pathologies are present in others, such as that dementia progresses during the course of repeated cerebrovascular disorder, small vascular lesions modify dementia, and cortical microinfarcts cause dementia. There are also several clinical diagnostic criteria [101, 102]. This report describes points to remember of imaging diagnosis based on the National Institute of Neurological Disorders and Stroke (NINDS)–Association Internationale pour la Recheche et l’Enseignement en Neurosciences (AIREN) diagnostic criteria including brain imaging, and introduces diverse imaging findings of cortical microinfarcts, cerebral autosomal dominant arteriopathy with subcortical infarcts and leukoencephalopathy (CADASIL), and cerebral amyloid angiopathy.
4.8.2 MRI Diagnosis
4.8.2.1 Multi-Infarct Dementia
Multiple large complete infarcts, usually from large-vessel occlusions involving cortical and subcortical areas, resulting in a clinical syndrome of dementia.
4.8.2.2 Strategic Single-Infarct Dementia
The cognitive impairment resulting from small, localized ischemic damage in functionally important cortical and subcortical areas is well defined [101, 103–106].
Regions in which cognitive decline-inducing strategic infarcts occur are shown in Table 4.4 [101, 103–106]. The Papez circuit is a series of neural circuits starting from the hippocampal formation to the fornix, mammillary body, mammillo-thalamic tract (Vicq d’Azyr bundle), anterior thalamic nucleus, cingulum, entorhinal cortex, and returns to the hippocampal formation, and cerebrovascular disorder impairing the Papez circuit is involved in cognitive decline because the circuit is closely involved in the memory.
Paramedian thalamic infarction occurs on the bilateral sides in many cases, and bilateral paramedian thalamic lesions are often accompanied by rostral midbrain lesions, producing a mesencephalothalamic syndrome of which a triad of symptoms are altered mental status, vertical gaze palsy, and amnesia. The paramedian thalamic area is perfused by the thalamoperforating artery branching from the P1 segment of the posterior cerebral artery (PCA), but there is an anatomic variant termed the artery of Percheron (AOP) branching from the PCA as a single dominant thalamoperforating artery, and many bilateral paramedian thalamic infarcts are caused by obstruction of the AOP [105]. Figure 4.28 shows a male patient in his 50s in whom cognitive decline occurred after sudden development of vertical eye movement. Infarcts were detected in the bilateral paramedian thalami and midbrain.
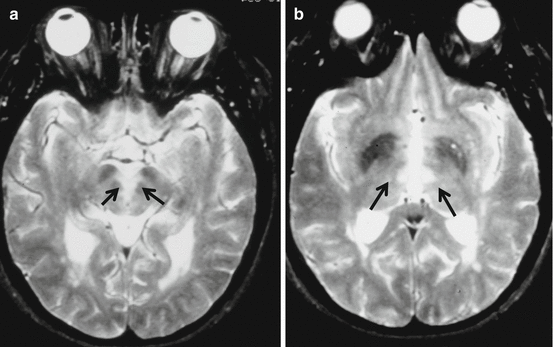
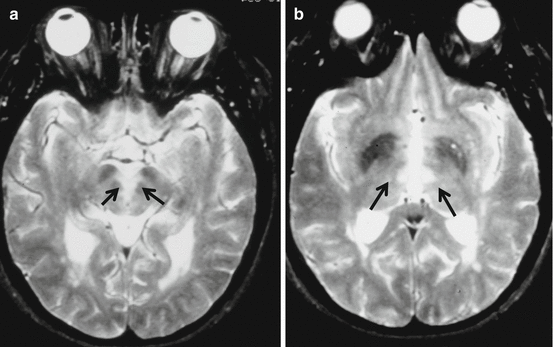
Fig. 4.28
Male patient in his 50s in whom vertical eye movement suddenly occurred. (a, b) T2-weighted transverse image shows infarcts in the bilateral paramedian thalami and midbrain (arrows). (From Tokumaru A et al.: Neuro-Ophthalmology Japan. Vol. 15(3) with permission)
Figure 4.29 shows a male patient in his 60s with amnesic cognitive dysfunction accompanying infarction of the subcallosal artery territory (ScA) which branches from the anterior communicating artery (A-com). High-intensity lesions were detected in the bilateral anterior commissures and columns of the fornix on diffusion-weighted imaging and FLAIR (Fig. 4.29). Stroke in the vascular territory of the ScA leads to a characteristic imaging and clinical pattern. Ischemia involves the anterior columns of the fornix and the genu of the corpus callosum, and patients present with Korsakoff’s syndrome including cognitive disturbances. These regions should also be noted in those involved in cognitive decline after surgery for A-com aneurysm [106].
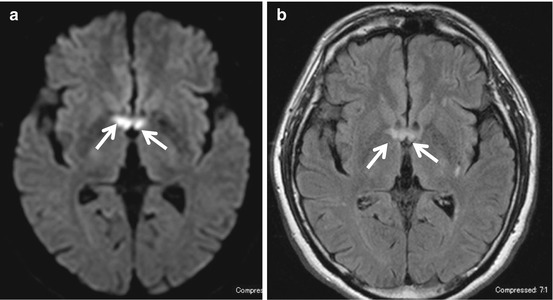
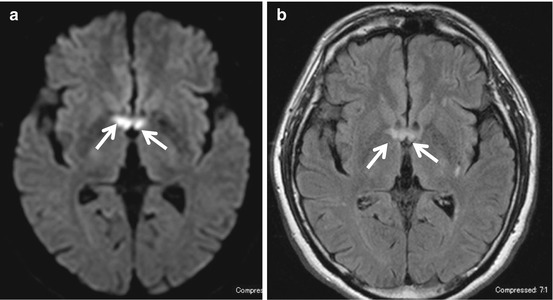
Fig. 4.29
60s M: Amnesia and cognitive disorder suddenly developed several days earlier. (a, b) Amnesia and cognitive disorder (strategic infarct) accompanying infarction of the bilateral columns of the fornix and anterior commissure (region perfused by the subcallosal artery)
Figure 4.30 shows a female patient in her 70s in whom secondary degeneration of the mammillo-thalamic tract occurred after infarction of the left anteromedial thalamus and the ipsilateral mammillary body atrophied, this was the responsible lesion for her memory disturbance.
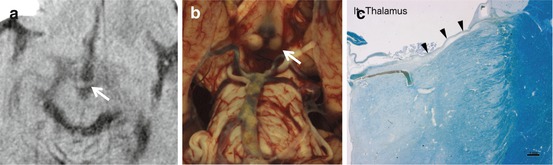
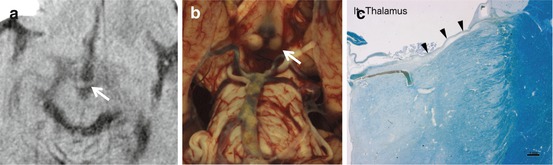
Fig. 4.30
70s female: Memory disturbance. (a, b) Axial CT image shows atrophy of left mammillary body (arrow). Macrospecimen represents atrophy of left mammillary body (arrow). (c) KB stain shows left medial thalamic infarct. After the development of left thalamic infarction (arrowheads), mammillary body atrophy occurred due to secondary degeneration of the mammillo-thalamic tract. (From Tokumaru A et al.: Japanisch-Deutsche Medizinische Berichte. Vol. 52(4) with permission)
4.8.2.3 Small Vessel Disease with Dementia
Lesions caused by small vessel disease may be cortical or subcortical: the latter include lacunes and white matter lesions frequently observed in the brains of elderly people. Lesions in the multiple basal ganglia and white matter lacunes are caused by obstruction of the perforating and medullary arteries. Binswanger’s disease (BD) is characterized clinically by pseudobulbar signs, behavioral changes, memory disturbances, psychomotor retardation, and other subcortical features such as gait disturbances, urinary incontinence, and parkinsonian signs. BD is also termed subcortical arteriosclerotic encephalopathy and forms diffuse ischemic lesions extensively in the cerebral white matter. High-intensities are extensively observed in the white matter on T2-weighted imaging and FLAIR on MRI, and complications by multiple lacunes and small hemorrhages are frequently observed (Fig. 4.31). In BD, in elderly hypertensive patients (particularly, nocturnal hypertension) who survived arteriosclerosis-induced large vascular obstruction and large cardiac vascular events, progression of cerebral hypertensive small vessel disease and chronic hypoperfusion generate lacunes and white matter lesions mainly in the frontal lobe in which the arterial and venous densities are embryologically low [107–109]. In BD, not only ischemia in the region perfused by the medullary artery but also watershed ischemia with the lenticulostriate and medullary arteries and perforating branch of the insular cortex are important causes. In this context, prevention of not only hypertensive small vessel disease but also chronic hypoperfusion is included in the treatment strategy.
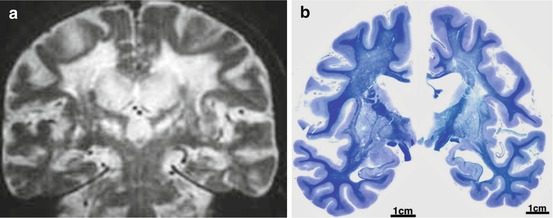
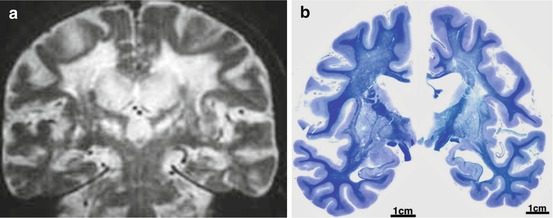
Fig. 4.31
Binswanger’s disease. (a) On coronal T2-weighted imaging, diffuse high-intensities in the white matter, multiple small infarcts in the bilateral thalamic and basal ganglias, and microbleeding were observed. (b) On myelin sheath staining, myelin sheath sustainability decreased corresponding to the high-intensities on T2WI, but U-fibers were relatively retained. Multiple small infarcts and microbleeding were scattered in the basal ganglia and thalamus
4.8.2.4 Cortical Microinfarcts and Cognitive Decline
The involvement of cortical microinfarcts in cognitive impairment has recently been attracting attention from clinical, imaging, and pathological aspects [110, 111]. Reportedly, microinfarcts microscopically observed in the cerebral cortex are involved in cognitive decline. It has been reported that microinfarcts can be detected by carefully observing FLAIR, and cortical microinfarcts can be more clearly visualized using double inversion recovery (DIR) and 3-dimensional fluid attenuated inversion recovery (3D-FLAIR) on 3-Tesla MRI [111]. Mechanisms by which microinfarcts relate to dementia and cognition are currently unknown. Half of those with microinfarcts did not have macroscopic infarcts; in addition, the microinfarct effects were independent of other common pathologies. Association with cerebral amyloid angiopathy and the possibility of artery-to-artery embolic infarcts are considered, for which it may be essential to clearly visualize cortical microinfarcts on MRI and build up investigations of correspondences with clinical and pathological findings and risk factors [110, 111]
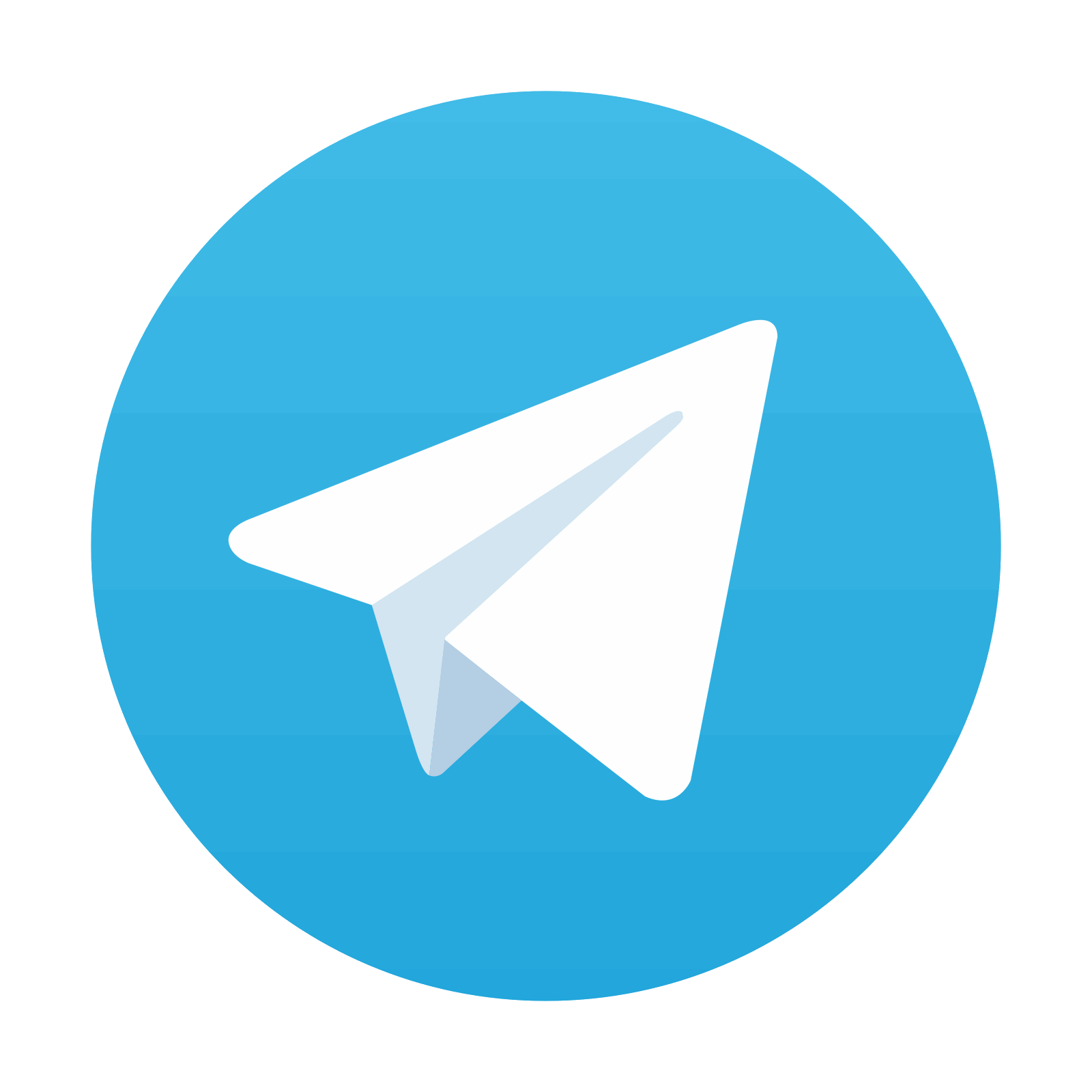
Stay updated, free articles. Join our Telegram channel

Full access? Get Clinical Tree
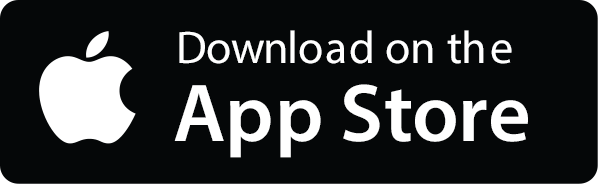
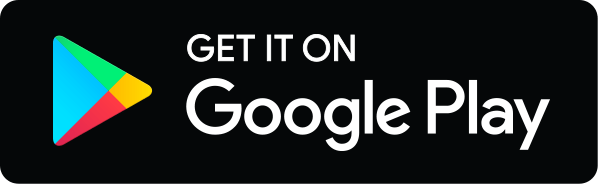