Imaging modality
Sensitivity
Limitations
CR
Radiation exposure
May take 7–10 days after injury to diagnose fracture
2-D representation of 3-D information
Sensitivity varies widely depending on anatomic location of injury
Assumes technically well done studies that use proper MAs, kVp, etc., and include all pertinent views
CT
Radiation exposure
Limited effectiveness in diagnosis of incomplete and non-displaced, complete fractures
MRI
95 %
Specificity less for small avulsion-type fractures that are often better detected with CR or CT
BS
95 %
Imaging not performed until 3–4 h after injection
Usually takes 2–3 days after injury to diagnose fracture with high sensitivity in elderly adults
Specificity less for non-acute fractures
Another problem with both BS and MRI is that many institutions, for economic reasons, do not offer these modalities 24 h a day or even every day of the week. If neither BS nor MRI is available, CT is the next best examination.
In contrast to extremity fractures, CT is used routinely in the initial evaluation of acute spine trauma. According to the National Emergency X-Radiography Utilization Study (NEXUS) criteria or Canadian C-Spine Rule (CCR) for cervical spine injury (CSI) criteria, MDCT with sagittal and coronal reconstructions is generally the preferred first imaging study for patients at high risk for fracture [1, 6]. This migration from CR to CT has occurred in part because CR only has 70 % sensitivity for cervical spine fractures. In pediatric patients less than 14 years of age where the incidence of spinal injury is lower, CR remains the initial imaging procedure of choice for acute spinal injury, in the cervical, thoracic, and lumbar spine in order to minimize radiation exposure [1, 6].
Generally, in cases where MDCT is used for initial assessment of acute spinal trauma, the entire spine should be imaged because severe trauma patients have a high incidence of multiple, noncontiguous injuries [1, 6]. It should be noted that thoracic and lumbar CT reconstructions derived from thoracic-abdomen-pelvic examinations are adequate substitutes for primary spine imaging, obviating the need for additional, formal spine CT imaging and thus avoiding unnecessary radiation dose to the patient.
Spine MRI is excellent for evaluation of patients in which there is clinical suspicion for spinal cord injury, cord compression, or ligamentous instability. Thus, MDCT of the cervical spine should be supplemented with an MRI in patients with posttraumatic myelopathy, with clinical or imaging findings worrisome for ligamentous injury, or with a mechanically unstable spine for presurgical planning [1, 6]. In cases with clinical or imaging findings suggestive of arterial injury, MDCT of the cervical spine should typically be accompanied by CTA or MRA of the head and neck [1, 6].
In both pediatric and adult populations, the major role of CT in evaluation of extremity fractures is for surgical planning. CT provides extensive information on the 3D anatomy and spatial relationships of fracture fragments. It is able to assess whether or not a fracture involves a joint and show how much diastasis and step off is present at the articular surface [2, 3, 7, 8]. CT may occasionally provide information about tendon entrapment. Typically, it is at the orthopedist’s discretion to request a planning CT once the decision has been made to operatively reduce the fracture. CT angiography can be useful to confirm arterial injury in cases where vascular compromise is clinically suspected from signs and symptoms such as abnormal pedal pulses, skin pallor, parathesias, and coolness of the extremity [9].
MRI can occasionally be of use in preoperative planning of extremity fracture reduction. Its role relates to identifying accompanying soft tissue injury [9], typically after fracture-dislocations caused by high-energy trauma, e.g., dislocation of the femorotibial joint of the knee. Here, MRI not only displays the status of the ligaments, but also of the menisci, tendon insertions, and focal articular cartilage defects.
Stress fractures frequently are difficult to visualize using CR, particularly insufficiency type stress fractures because of the associated osteopenia. The sensitivity of CR for early stress fracture detection may be as low as 15 % on initial imaging, with follow-up X-rays sensitivity increasing to only 50 % [10, 11]. Nonetheless, it is reasonable to begin the patient’s evaluation with CR primarily to exclude other pathology. Often, however, an alternative study, either BS or MRI, will be required to diagnose the fracture. Both have a high degree of accuracy for this diagnosis, but MRI is generally the preferred examination because it depicts all of the anatomy and it uses no ionizing radiation. Of course, MRI is more expensive than BS, and this difference should be taken into consideration.
Because BS only shows abnormal metabolic activity, findings are nonspecific and always should be compared with recent CR [11, 12]. This practice will prevent incorrectly interpreting a BS abnormality as a presumed clinical diagnosis. For example, an osteoid osteoma and a stress fracture will have similar BS appearances, but these are very different entities, requiring different therapy.
US plays a limited role in initial fracture diagnosis. US, using CR as a standard, has a sensitivity and specificity of 94 % for lipohemarthrosis and hence detection of occult fractures with intra-articular extension (Fig. 6.1).
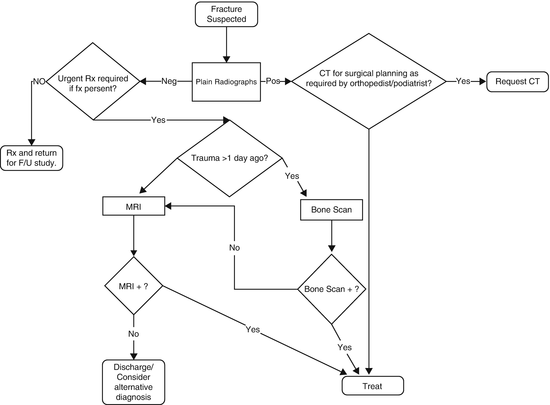
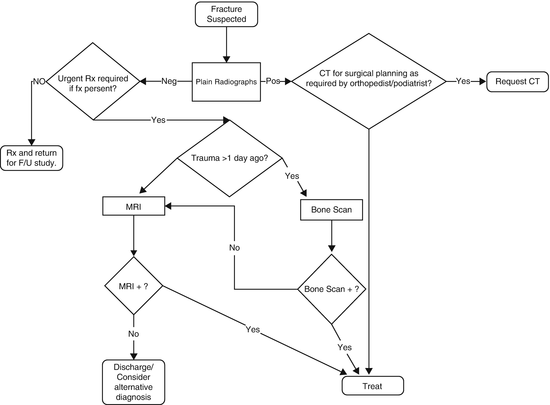
Fig. 6.1
Workup of osseous trauma
Soft Tissue Trauma
Trauma to muscles, ligaments, and tendons may occur acutely as with a sudden muscle strain or from chronic repetitive trauma, as with overuse syndromes like “tennis elbow.” Other common soft tissue injuries include muscle contusions and intramuscular hematomas, cruciate ligament injury and meniscal tears in the knee, rotator cuff tears of the shoulder, shoulder glenoid and hip acetabular labral tears, carpal intrinsic ligament tears, sprains of the ankle, ankle and wrist tenosynovitis, and plantar fasciitis in the foot. Tendons, e.g., the rotator cuff, biceps at the shoulder and elbow, gluteal, hamstring, adductor, quadriceps, and Achilles tendons, may tear as a result of either acute and/or chronic trauma or as a result of other infiltrating pathology that causes degradation of the tendon’s integrity, e.g., fluquinolones, xanthomas, or tophi.
Regardless of whether the trauma is acute or chronic, MRI and US are the preferred imaging modalities for diagnosis. CR may be helpful initially to exclude underlying pathology masquerading as trauma and to provide information about the adjacent osseous structures that MRI or US might not show. For instance, an avulsion fracture from the dorsal triquetrum at the attachment of the ulnotriquetral ligament will be better depicted on CR and aid the underlying soft tissue diagnosis.
MRI easily distinguishes among several different types of soft tissue, displaying a high level of anatomic detail for the evaluation of muscles, tendons, ligaments, fat, fascia, hyaline articular cartilage, and fibrocartilage, e.g., joint labra and menisci, the triangular fibrocartilage complex (TFCC) of the wrist, and the articular disc of the temporomandibular joint (TMJ). It can image any part of the body as long as there is no contraindication to placing a patient in the magnet and there is no artifact inducing material near the part to be imaged.
US is useful in evaluation of tendons and ligaments when the target structure is accessible to the penetrating US waves. The exam is usually most efficacious when applied to specific clinical questions that require focused imaging performed in a small anatomic area. Suspected tears of the Achilles, patellar, quadriceps, hamstring tendons, and rotator cuff fall into this category.
As discussed above, US shows anatomy in real time, allowing for visualization of motion. This can be useful in trauma to elicit extensor tendon subluxation in the fingers related to ligament tears, ulnar nerve subluxation-dislocation in and out of the cubital tunnel at the elbow, ankle tendon dislocations at the hind foot, and myofascial tears of muscles.
CT, in some cases, can diagnose trauma to tendons, muscles, and ligaments, but compared with MRI, its capability is limited. CT suffers from poor contrast resolution in evaluating the musculoskeletal system.
Although soft tissue abnormalities, both traumatic and non-traumatic, occasionally can be detected on BS, this study is not accurate enough to warrant its use for this purpose. In fact, these findings typically are noted incidentally on a BS obtained for a different purpose (Fig. 6.2).
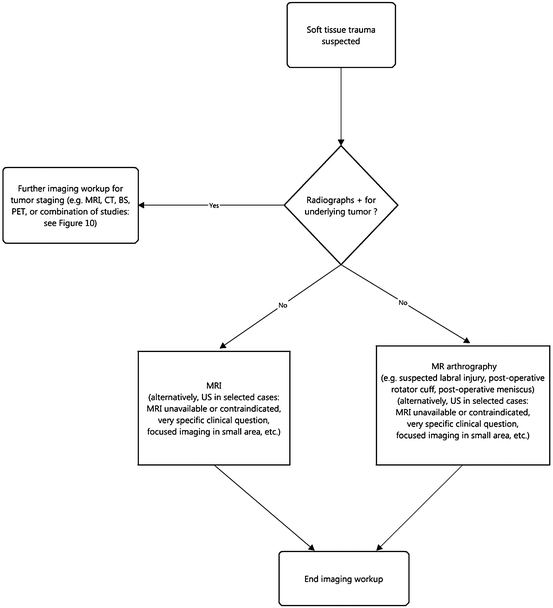
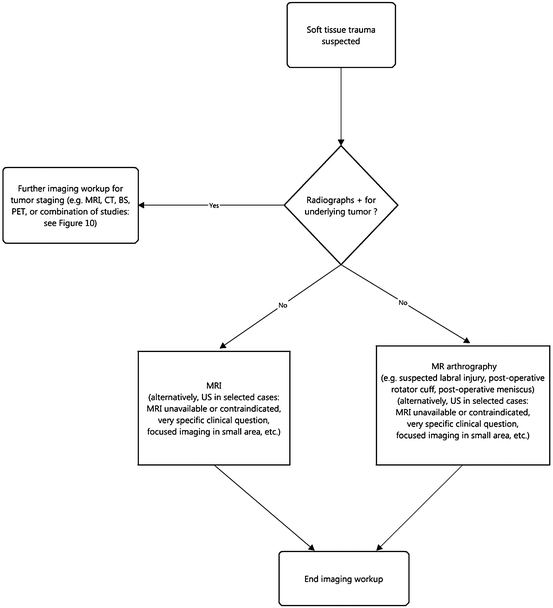
Fig. 6.2
Workup of soft tissue trauma
Infection
Osseous Infection (Osteomyelitis)
Osteomyelitis is common in certain populations, e.g., diabetics. The vast majority (>90 %) of pediatric cases of osteomyelitis arise through hematogenous dissemination of the infectious agent, usually Staphylococcus aureus [13, 14]. Adult osteomyelitis, on the other hand, overwhelmingly (>90 %) results from contiguous spread of adjacent soft tissue infection, whether from a soft tissue ulcer or less commonly pyomyositis [13]. A small proportion of osteomyelitis in adults results from hematogenous spread [13]. This occurs most commonly in patients who have large intravascular boli of organisms, e.g., intravenous drug users (IVDA) in whom the spine and sternoclavicular and acromioclavicular joints are common sites of infection [13, 15]. Osteomyelitis in any bone can spread to adjacent joints and cause septic arthritis [13, 16].
Of course, imaging can be employed not only to diagnose osteomyelitis, but also to evaluate healing in response to treatment. Finally, CR is valuable in defining postoperative anatomy in patients who have had normal anatomy altered either surgically or from neuropathic arthropathy.
Although the specificity of CR for osteomyelitis is moderately high (80 %), its sensitivity is low (54 %). The low sensitivity results from the fact that there must be substantial trabecular bone destruction for osteomyelitis to be evident on plain radiographs, usually 50–70 % [14]. As a result, the destructive changes associated with osteomyelitis typically are not demonstrated by radiographs until 10–14 days after the start of the infection [13, 14, 17]. CR’s sensitivity for sequestra (10–15 %) and cloacae is also low [18]. As with acute fractures, the delay between infection’s onset and visibility on CR is more prolonged in the elderly population.
Although CR may have a lower sensitivity than some other modalities, it is inexpensive. If CR reveals osteomyelitis, the work up can stop there in most cases. Furthermore, if additional imaging is required, a plain radiographic study of the same body part is essential for comparison, especially when there is confusing or altered anatomy, e.g., patients with amputations [17].
MRI and NM have equivalent high sensitivity for diagnosis of osteomyelitis, but the former is able to detect the infection slightly earlier in its course, at most a day or two. As with occult fractures, BS may not show osteomyelitis in elderly adults until 2–3 days from the onset of infection. Once again, BS is less expensive than MRI.
Thus, while CR is the initial imaging modality of choice in the diagnostic workup for osteomyelitis, MRI is usually the second imaging study chosen if CR is non-diagnostic [13, 14]. MRI is exquisitely sensitive to cortical destruction and also can show bone marrow and soft tissue edema. Typically, IV contrast does not increase MRI’s sensitivity for acute osteomyelitis. On the other hand, contrast often can be helpful in detection of findings typically associated with osteomyelitis such as soft tissue and intra-osseous abscesses and bony sequestra [13]. MRI with added IV contrast plus fat suppression has been reported to raise specificity for osteomyelitis from 81 to 93 % [19]. Furthermore, contrast can aid in the differentiation of nonviable necrotic soft tissues from viable tissue, thus aiding operative planning [17, 20].
Unfortunately, the specificity of MRI for acute osteomyelitis drops in complicated cases that involve acute or chronic osteomyelitis; patients who are recently postoperative; patients who have had a recent fracture; or who have underlying conditions such as neuropathic or inflammatory arthropathy [14, 17]. In some cases, particularly in patients with neuropathic arthritis, labeled WBC radionuclide scans or FDG-PET scans are more efficacious than MRI to diagnose associated osteomyelitis [14]. Occasionally, bone biopsy will frequently be required to make the diagnosis or if an unusual organism is suspected.
Findings on follow-up MRI studies in patients with osteomyelitis routinely lag the clinical picture, and so can give an incorrect impression of the status of patients who are undergoing or recently had treatment. Findings on MRI such as marrow edema and marrow enhancement may worsen during the treatment phase, not showing improvement until later on. Regardless, evidence of progressive bone destruction should not be evident and indicates worsening infection.
In patients who are unable to have MRI, whether due to unavailability of or contraindication to the exam, BS may be used instead. As mentioned, BS has equally high sensitivity for detection of osteomyelitis as MRI and as a result has extremely high negative predictive value; a negative study virtually excludes osteomyelitis [14]. In addition, BS allows imaging of the entire skeleton, making it valuable in cases of suspected multifocal infection such as chronic recurrent multifocal osteomyelitis. The main drawbacks of BS are its inability to detect infection as early as MRI, and its lower specificity compared with MRI, CR, and other studies [14]. The development of single photon emission CT (SPECT) in registration with standard CT has mitigated some of these issues but also has increased the cost of nuclear medicine studies substantially. In some special circumstances, such as cases of multifocal osteomyelitis and osteomyelitis around prostheses, BS combined with labeled WBC study can be particularly beneficial; the labeled WBC study improves the low specificity of BS alone [17]. Labeled WBC studies are most useful in the appendicular skeleton. Many studies have shown problems with false negatives and low sensitivity for osteomyelitis of the spine evaluated with labeled WBC [21].
Diagnosing ongoing chronic osteomyelitis can be difficult. Early studies using FDG-PET showed higher sensitivity and specificity than other NM studies and MRI both [14]. A recent meta-analysis that reviewed the accuracy of multiple imaging modalities for the diagnosis of chronic osteomyelitis showed FDG-PET to be the most accurate, with a sensitivity of 96 % and specificity of 91 %. In comparison, the sensitivity and specificity of MRI was 84 % and 60 %, respectively. Labeled WBC study had a sensitivity of 84 % and a specificity of 80 %, but these values decreased considerably when cases involving the axial skeleton were included [22]. Relative unavailability and high cost are significant stumbling blocks for FDG-PET.
Although MRI often can diagnose a sequestrum, CT is slightly more sensitive because it is exquisitely sensitive for detecting calcification and ossification [13]. CT is especially applicable if the suspected sequestrum is small or IV contrast cannot be administered with the MRI [13, 14]. On the other hand, if a patient can tolerate IV contrast, MRI is superior to CT in determination of the viability of infected bone, and even more accurate than CT in the detection of necrotic soft tissues that may require surgical debridement [13, 17].
In selected locations in the body where radiographs do not display the anatomy clearly CT is the preferred initial examination in cases of suspected osteomyelitis, e.g., sternoclavicular joints [13, 14]. CT also may be preferred in areas where respiratory motion may degrade MRI image quality, e.g., the chest and abdominal walls [23, 24]. CT, if positive, is capable of providing precise anatomic localization of osteomyelitis. It also is able to guide bone biopsy. CT is very limited in evaluation of the marrow space compared with MRI [14, 17] (Table 6.2).
Table 6.2
Osteomyelitis: efficacy of imaging modalities
Imaging modality | Sensitivity | Specificity | Accuracy | Advantages | Limitations |
---|---|---|---|---|---|
CR | 54 % (22–93 %) | 80 % (50–94 %) | May demonstrate an alternative diagnosis (i.e., fracture nonunion, tumor) | Radiation exposure | |
Excellent overview and delineation of anatomy (especially beneficial in postoperative and neuroarthropathy patients) | 2-D representation of 3-D information | ||||
Aids characterization of the distribution of arthritic changes (including neuropathic osteoarthropathy) | Usually takes 10–14 days after infection onset to diagnose osteomyelitis | ||||
Useful in detection of soft tissue gas | Bone destruction needed for visualization of the abnormality may be 60 % | ||||
Specificity moderate | Sensitivity low to very low | ||||
— | |||||
Endplate cortical destruction in osteomyelitis-discitis usually not evident until at least 4–6 weeks after infection onset | |||||
Specificity in spondylodiscitis reduced by other causes of disc space narrowing (e.g., DDD), endplate erosive changes (e.g., severe DDD), and gross bone destruction (e.g., neuropathic spondyloarthropathy, amyloid arthropathy) | |||||
CT | Helpful in evaluation of deep structures and assessment of atypical and irregular bones and joints, and complex joint anatomy (e.g., sternum, sternoclavicular joint, spine, pelvis) | Radiation exposure | |||
Precise anatomic localization of findings | Soft tissue contrast moderate, but lower than MRI | ||||
Depicts subtle, early bone cortex erosion | Much less sensitivity for marrow infection (vs. MRI) | ||||
Very good for sequestrum detection | |||||
Can guide bone biopsy | Insensitive, inadequate assessment of degree of marrow involvement | ||||
Accuracy in determination of viability of infected bone and soft tissues lower than MRI | |||||
— | |||||
Inferior soft tissue contrast resolution results in decreased sensitivity for epidural abscess complicating spondylodiscitis | |||||
MRI | 92 % (29–100 %) | 84 % (78–89 %) | 89–98%a | Comprehensive assessment of entire spectrum of bone and soft tissue infection pathology | Not always (easily) available |
84%a | 60%a | ||||
Excellent bone marrow evaluation | Expensive | ||||
Outstanding soft tissue contrast aids diagnosis of coexisting pathology (e.g., soft tissue abscess, sinus tract, devitalized soft tissue) | Long study length may result in image quality degraded by motion artifact (difficult for severely ill patients to remain in scanner entire study) | ||||
Superb anatomic detail | Nephrogenic systemic fibrosis (NSF) risk from IV gadolinium-based contrast agents (GBCAs) | ||||
Provides surgeon with preoperative anatomic “road map” of pathology | Specificity decreased in setting of recent fracture, recent surgery, neuropathic osteoarthropathy, and inflammatory arthritis | ||||
Sensitivity and specificity high (>90 %) | Nonspecific result is not uncommon but may help in evaluation of complex cases of chronic osteomyelitis | ||||
May help in evaluation of complex cases of chronic osteomyelitis but nonspecific result is not uncommon | — | ||||
— | Limited ability to accurately differentiate pyogenic vs. mycobacterial etiology in osteomyelitis-discitis | ||||
Highest sensitivity (96 %), specificity (92 %), and accuracy (94 %) modality for spondylodiscitis | |||||
BS | 91 % (69–95 %) | 46 % (38–100 %) | Allows for evaluation of entire body (particularly helpful with multifocal osteomyelitis) | Usually takes 2–3 days after infection onset to diagnose osteomyelitis in elderly adults | |
Sensitivity high (equal to MRI) | Moderate study length (images obtained 3–4 h after radiotracer injection) | ||||
Negative predictive value (NPV) high | Specificity very low | ||||
Low resolution, suboptimal anatomic localization (but improved by SPECT or CT) | |||||
Labeled WBC | 86 % (45–100 %) | 84 % (67–89 %) | 78–90 % | Allows for evaluation of entire body (particularly helpful with multifocal osteomyelitis) | Very long study length (imaging performed 2–4, 24, and possibly 48 h after injection) |
84%a | 80%a | ||||
Sensitivity high | Specificity reduced in setting of recent fracture, recent surgery, neuropathic osteoarthropathy, and inflammatory arthritis | ||||
Negative predictive value (NPV) high | Sensitivity for vertebral osteomyelitis much lower than for bone infection in the extremities | ||||
— | |||||
NM commonly favored in setting of postoperative spondylodiscitis because of reduced specificity of MRI | Low resolution, suboptimal anatomic localization (but improved by SPECT or CT) | ||||
BS + labeled WBC | 88 % (73–100 %) | 82 % (55–91 %) | 81 % | Allows for evaluation of entire body (particularly helpful with multifocal osteomyelitis) | Very long study length (imaging performed 2–4, 24, and possibly 48 h after injection) |
Sensitivity high | Specificity reduced in setting of recent fracture, recent surgery, neuropathic osteoarthropathy, and inflammatory arthritis | ||||
Negative predictive value (NPV) high | Low resolution, suboptimal anatomic localization (but improved by SPECT or CT) | ||||
Specificity of BS increased by addition of labeled WBC study, and vice versa | |||||
— | |||||
NM commonly favored in setting of postoperative spondylodiscitis because of reduced specificity of MRI | |||||
FDG-PET | 96%a | 91%a | 94 %a | Allows for evaluation of entire body (particularly helpful with multifocal osteomyelitis) | Relative lack of availability |
Sensitivity and specificity high | High cost | ||||
Negative predictive value (NPV) high | — | ||||
Particularly useful in difficult, complex cases of chronic osteomyelitis (vs. any modality) | Not reliable for osteomyelitis-discitis even though addition of CT increases specificity | ||||
—NM commonly favored in postoperative spondylodiscitis setting because of reduced specificity of MRI | |||||
Probe to bone | 66 % | 85 % | Presence of granulation tissue over ulcer very uncommon but may give false negative result |
US plays a minor role in the diagnosis of osteomyelitis. The modality cannot detect intra-osseous pathology such as medullary bone destruction, sequestrum, and intra-osseous abscess [14, 17]. US does have very good utility in the detection of infection of the soft tissues adjacent to infected bone and periosteal abnormalities primarily in children. For instance, US can identify periosteal elevation and accompanying subperiosteal fluid collections such as abscess, and it also is able to demonstrate neighboring soft tissue abscesses in patients with osteomyelitis [14]. In addition, in cases where osteomyelitis is intra-capsular in location, such as the femoral neck, US has high sensitivity in detection of joint effusion, but it cannot distinguish whether the effusion reflects complicating septic arthritis or is merely reactive in etiology [14]. US, like CT, can provide guidance for aspiration of fluid collections and joint effusions related to osteomyelitis (Fig. 6.3).
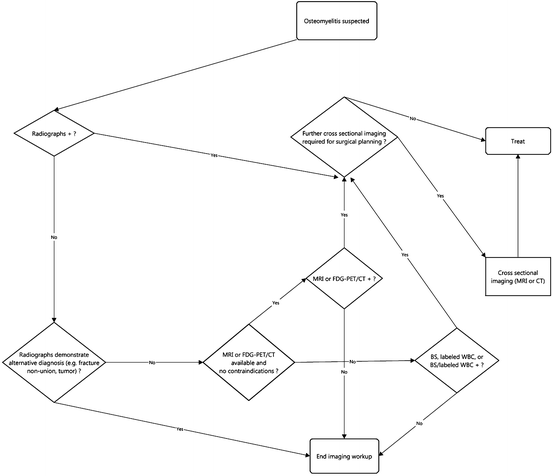
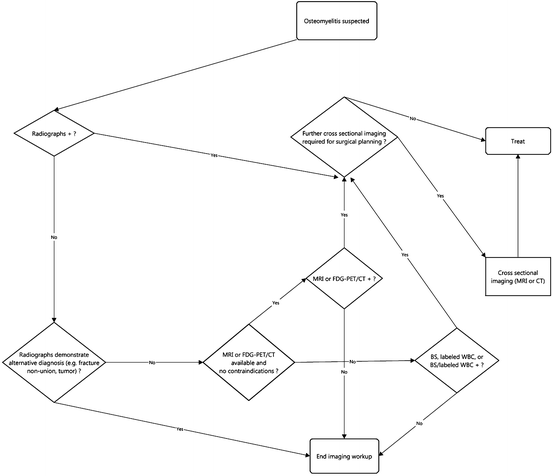
Fig. 6.3
Workup of osseous infection (osteomyelitis)
Spinal Infection (Osteomyelitis-Discitis, Spondylodiscitis)
Spinal osteomyelitis and discitis represents only approximately 5 % of all cases of osteomyelitis. Spondylodiscitis occurs most frequently in the lumbosacral spine. Cervical spine involvement is least common. Epidural spread is not uncommon but is a source of significant morbidity and mortality. Rarely, the infection spreads to the meninges and spinal cord, usually with devastating results [25]. As in the case of patients with extra-spinal osteomyelitis, bacterial infection is much more common than fungal or parasitic etiologies, and again S. aureus is the most common causative organism, accounting for more than half of cases (60 %) [25]. Gram negative pyogenic and polymicrobial infection is also frequently seen. Mycobacterium infection, including M. tuberculosis, is another common etiology, particularly in developing countries, where it is widespread and even endemic [25].
As with evaluation of osteomyelitis elsewhere, CR is the first study for imaging patients with suspected osteomyelitis-discitis. As with other locations, the sensitivity of X-rays for spondylitis is low, especially early in the course of the disease. In adults, endplate cortical destruction, the most specific finding for pyogenic infection, is usually not evident on radiographs until at least 4–6 weeks after the onset of infection [25]. The sensitivity of radiographs for spinal infections for non-pyogenic osteomyelitis-discitis is worse—minimal to none. CR also has limited specificity for discitis-osteomyelitis [25]. Overall, disc space narrowing is most frequently the result of degenerative disc disease and occasionally even erosion and irregularity may be seen in severe degenerative disc disease. Gross bone destruction and osseous fragmentation can be the result of amyloid spondyloarthropathy and neuropathic arthropathy [25].
MRI is the gold standard in imaging of spinal infection [13, 25]. Numerous studies have shown that MRI has very high sensitivity, specificity, and accuracy for osteomyelitis-discitis, approximately 96 %, 92 %, and 94 %, respectively. These figures exceed those of any other imaging modality [25]. MRI’s performance in detection of bone and disc infection stems from its excellent depiction of disc fluid, endplate cortical erosion, overt bone destruction, and bone marrow edema. It is also sensitive for identification of associated inflammatory phlegmon and abscess, usually either epidural or retroperitoneal within the psoas muscle. These usually require drainage for successful treatment. IV contrast can provide additional value, providing better delineation of fluid collections and improved detection of necrotic tissue and sequestra.
CT is sometimes beneficial in the workup of osteomyelitis-discitis. Like MRI, CT is capable of providing precise anatomic localization and detail in osteomyelitis-discitis. As expected, however, CT is beset by the same disadvantages relative to MRI as in the diagnosis of osteomyelitis outside the spine. Unless MRI is unavailable or contraindicated, CT is generally no longer used for this diagnosis.
NM studies play a small role in the initial diagnosis of osteomyelitis-discitis except in postoperative patients where distinction between operative changes and infection is difficult on MRI [25]. As with extra-axial infection, BS and labeled WBC studies either alone or in combination are typically used in postoperative patients. PET has not proven to be dependable in the diagnosis of spondylodiscitis, although the addition of CT improves anatomic localization and specificity (Fig. 6.4).
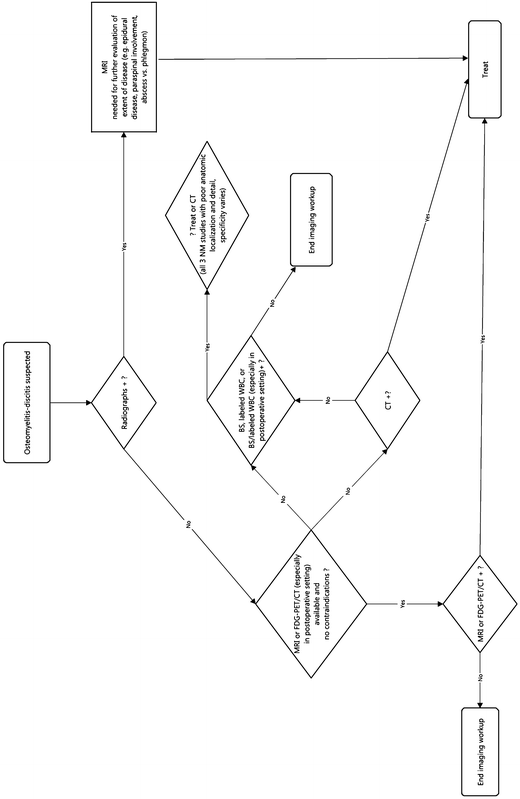
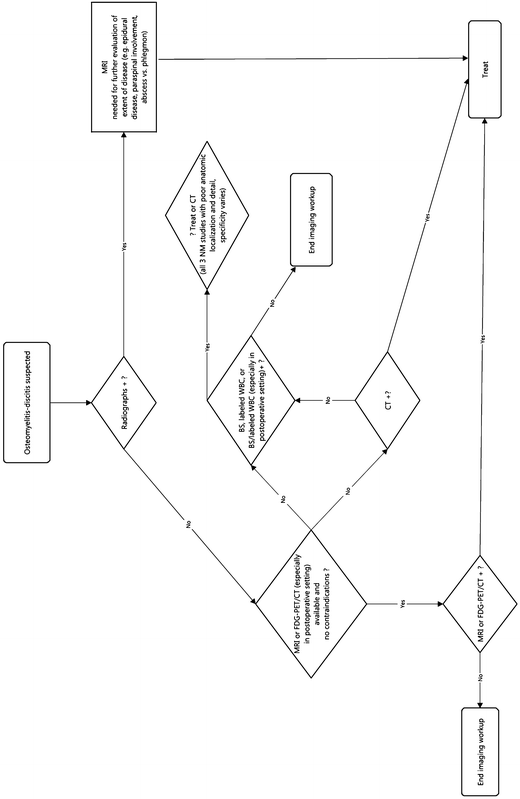
Fig. 6.4
Workup of spinal infection (osteomyelitis-discitis, spondylodiscitis)
Joint Infection (Septic Arthritis)
In the clinical setting of a single acutely painful joint, septic arthritis should be strongly considered and evaluated emergently to avoid rapid irreversible destruction of the joint [26]. Septic arthritis in children typically arises from hematogenous inoculation of the joint, while in adults it arises from direct inoculation of the joint. Osteomyelitis that is intracapsular to a joint also can give rise to septic arthritis [13, 16].
Certain patient populations have a predilection to develop septic arthritis, in particular anatomic locations. For instance, in IVDA, the acromioclavicular joints, sternoclavicular joints, vertebral discs, and sacroiliac joints are commonly involved [15]. Patients within 6 months of arthroplasty are also prone to infection.
Joint aspiration remains the gold standard in the diagnosis of acute septic arthritis [13, 26]. MRI and US can confirm the presence of joint fluid prior to joint aspiration, but they cannot reliably distinguish sterile joint fluid from infected joint fluid [26]. In fact, routine imaging cannot exclude septic arthritis even with a normal examination [13]. Regardless, both MRI and US are only occasionally performed and almost always are unnecessary since they do not obviate the need for joint aspiration [13]. On the other hand, fluoroscopy and US can be useful to guide joint aspiration procedures. When there is clinical concern for chronic septic arthritis, in the majority of patients joint aspiration remains the initial examination. However, one should at least consider performing synovial biopsy, placing more emphasis on evaluation for less common infectious agents such as mycobacteria and fungi [26].
To summarize, although laboratory results may be normal in an acutely infected joint, clinical data, i.e., elevated CRP, sedimentation rate, leukocytosis, fever, systemic infection, and joint pain, should be emphasized over and pursued earlier than most imaging studies. Judicious use of advanced imaging techniques such as MRI, US, and nuclear medicine may help exclude alternative diagnoses, but joint aspiration and culture is the examination of choice in cases of septic arthritis (Fig. 6.5).
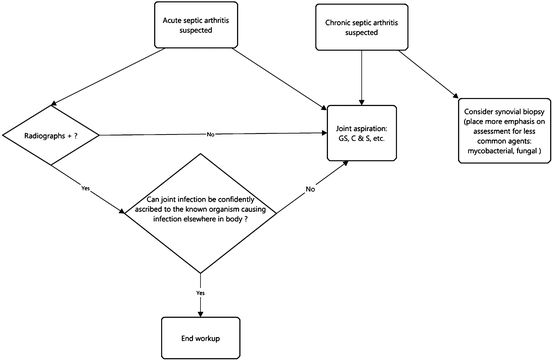
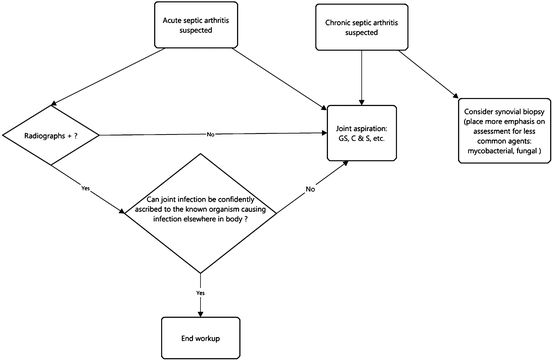
Fig. 6.5
Workup of joint infection (septic arthritis)
Soft Tissue Abscess
Soft tissue abscesses may arise through multiple pathways: direct implantation, infection in the adjacent soft tissues, or most commonly hematogenous spread of infectious organisms (usually bacteria) [13]. These fluid collections are generally seen more often in patients who have depressed immune systems, bacteremia, sepsis, infectious endocarditis, or a history of recent surgery or penetrating trauma. In the IVDA population, abscesses in the soft tissues are commonly related to the use of unsterilized needles and injectates, and so they tend to arise in areas where users inject.
Although CR has very low sensitivity for soft tissue abscess, this is the standard first imaging study, usually to exclude foreign bodies and soft tissue gas [17]. Radiographs rarely demonstrate a discrete appearing mass in the soft tissues. They more frequently will show focal soft tissue swelling and edema.
MRI and CT, both with IV contrast, routinely detect fluid collections in the soft tissues. Although MRI without IV contrast can detect abscesses, contrast enhanced MRI has greater sensitivity, particularly for smaller abscesses as may be seen with pyomyositis [13]. The soft tissue contrast resolution of CT with IV contrast is moderate and inferior to that of MRI. Moreover, depending on the timing of CT image acquisition relative to administration of the IV contrast, the fluid collection may have poor conspicuity and go undetected. Therefore, MRI with IV contrast is the preferred examination for diagnosis of a soft tissue abscess [13, 17, 26]. In addition to its utility in evaluation for soft tissue abscesses, MRI can characterize the extent of tissue devitalization and so facilitate operative planning for soft tissue debridement or amputation [17, 20].
Abscesses are also easily diagnosed with targeted US. Using color Doppler, US can add further value in some cases by assessing the vascularity of the wall of the collection and adjacent soft tissues. The presence of hypervascularity in the wall and surrounding soft tissue favors a diagnosis of abscess over a noninfected collection such as seroma or hematoma [17]. It should be cautioned, however, that there can be significant overlap in the vascularity and central echogenicity of these different types of fluid collections because seromas and hematomas can become superinfected. As a result, fluid aspiration often is needed for definitive diagnosis. Both CT and US can provide excellent guidance for this procedure [17, 27].
The differential diagnosis of soft tissue abscess on MRI, CT, and US includes muscle infarction and necrotic tumor [28]. Differentiating between abscess and necrotic tumor often can be done clinically. On the other hand, distinguishing between abscess and muscle infarction, most commonly seen as a complication of diabetes, typically requires aspiration to determine the cause of the collection.
Labeled WBC study for soft tissue abscess is less often utilized than MRI, CT and US because it provides limited anatomic localization of abscesses [29]. Furthermore, labeled WBC exams take much longer to perform than other modalities, and this can be a problem in acutely ill patients or because it can increase an inpatient’s length-of-stay. Nonetheless it has high sensitivity and excellent specificity for abscess (Table 6.3, Fig. 6.6).
Table 6.3
Soft tissue abscess: efficacy of imaging modalities
Imaging modality |
---|